How fast does the Earth move through the Universe?
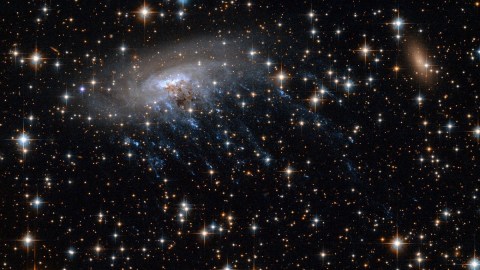
And if relativity tells us there’s no such thing as “absolute motion,” how do we measure it?
“The slow philosophy is not about doing everything in tortoise mode. It’s less about the speed and more about investing the right amount of time and attention in the problem so you solve it.” –Carl Honore
Most likely, as you’re reading this right now, you’re sitting down, perceiving yourself as stationary. Yet we know — at a cosmic level — we’re not so stationary after all. For one, the Earth rotates on its axis, hurtling us through space at nearly 1700 km/hr for someone on the equator.
That’s not really all that fast, if we switch to thinking about it in terms of kilometers per second instead. The Earth spinning on its axis gives us a speed of just 0.5 km/s, hardly a blip on our radar when you compare it to all the other ways in which we’re moving. The Earth, you see, much like all the planets in our Solar System, orbits the Sun at a much speedier clip. In order to keep us in our stable orbit where we are, we need to move at right around 30 km/s. The inner planets — Mercury and Venus — move faster, while the outer worlds like Mars (and beyond) move slower than this. This was true in the distant past and will continue to be true into the distant future.
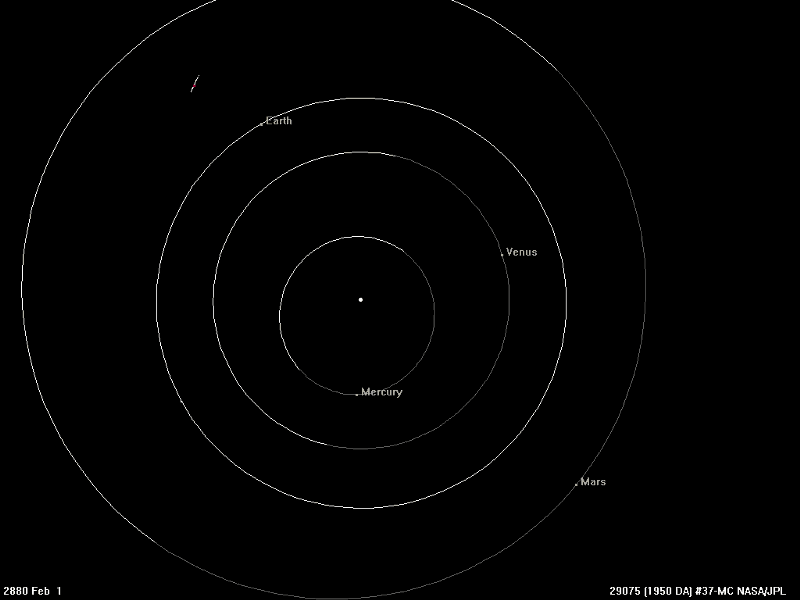
But even the Sun itself isn’t stationary. Our Milky Way galaxy is huge, massive, and most importantly, is itself in motion. All the stars, planets, gas clouds, dust grains, black holes, dark matter and more — everything contained within — move around inside of it. Every particle of matter and energy both contribute to and are affected by its net gravity.
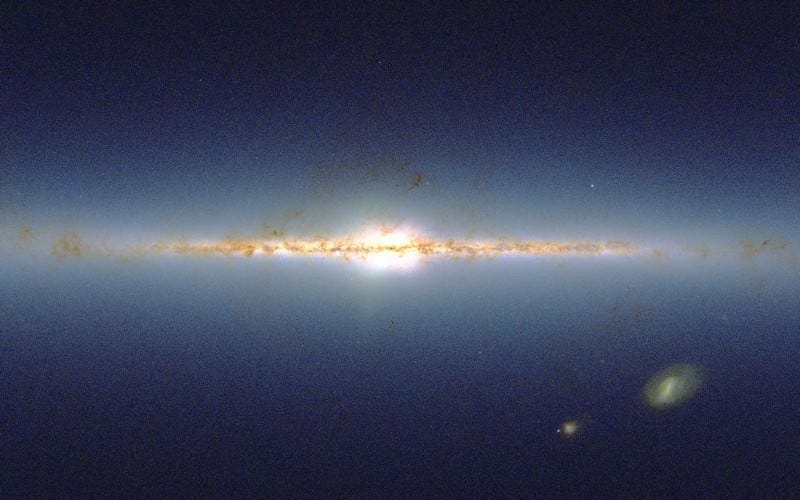
From our vantage point, some 25,000 light years from the galactic center, the Sun speeds around in an ellipse, making a complete revolution once every 220–250 million years or so. It’s estimated that our Sun’s speed is around 200–220 km/s along this journey, which is quite a large number compared to not only our rotational speed of Earth but of all the planets’ revolutions around the Sun. Nevertheless, we can put all these motions together, and find out what our motion through the galaxy is.
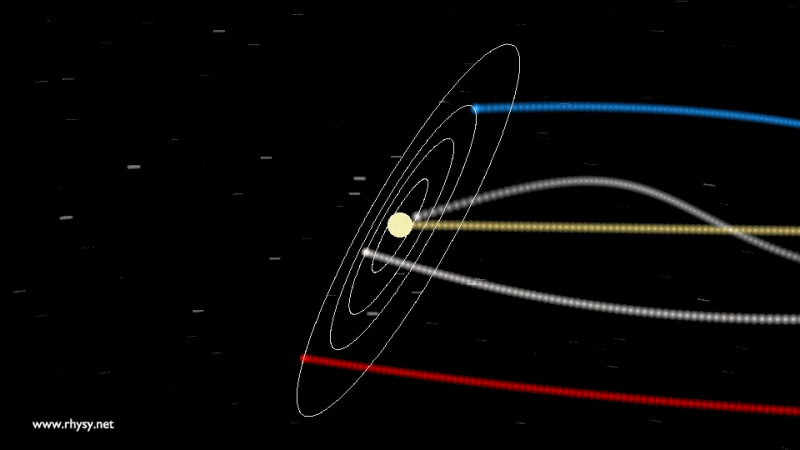
But is our galaxy itself stationary? Most certainly not! In space, you see, there’s the gravitation of every other massive (and energetic) object to contend with, and gravitation causes any masses around to accelerate. Give our Universe enough time — and we’ve had some 13.8 billion years of that — and everything will move, drift and flow in the direction of the greatest gravitational attraction. That’s how we go from a mostly uniform Universe to a clumpy, clustered, galaxy-rich Universe in relatively short order.
That’s the cosmic story of structure formation, taking place within the expanding Universe. So what does that mean out near us? It means our Milky Way is being pulled by all the other galaxies, groups and clusters in our vicinity. It means that the closest, most massive objects around are going to be the ones that dominate our motion, and that they have for the entire cosmic history. And it means that not only our galaxy, but all the nearby galaxies are going to experience a “bulk flow” due to this gravitational force. Recently, this has been mapped to the greatest precision ever, and we’re continually coming closer to understanding our cosmic motion through space.
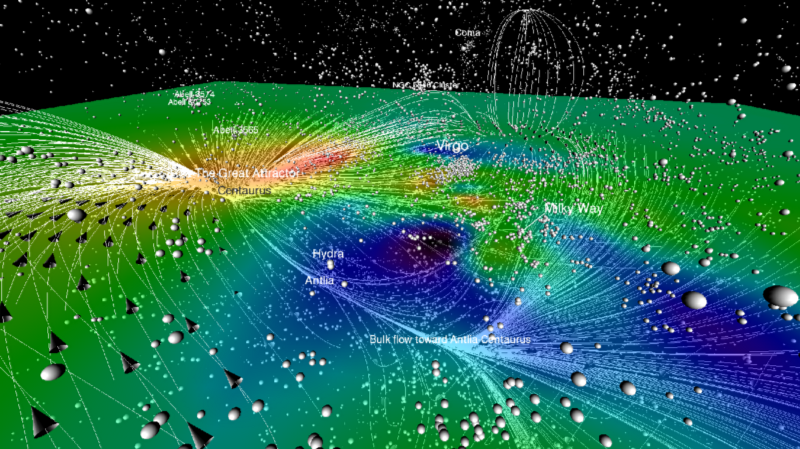
But until we fully understand everything in the Universe that affects us, including:
- the full suite of initial conditions under which the Universe was born,
- how each individual mass moved and evolved over time,
- how the Milky Way and all the associated galaxies, groups and clusters formed, and
- how that happened at every point in cosmic history up through the present,
we won’t be able to truly understand our cosmic motion. At least, not without this one trick.
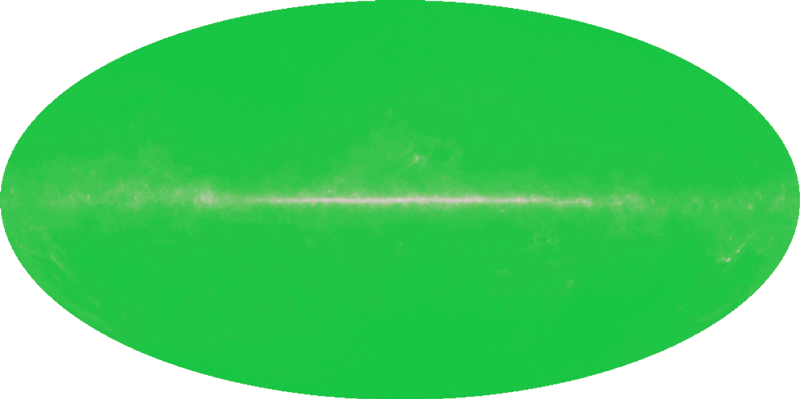
You see, everywhere we look in space, we see this: the 2.725 K radiation background that’s left over from the Big Bang. There are tiny, tiny imperfections in various regions — on the order of just a hundred microkelvin or so — but everywhere we look (except in the polluted plane of the galaxy, where we cannot see), we observe that same temperature: 2.725 K.
This comes about because the Big Bang happened everywhere at once in space, 13.8 billion years ago, and the Universe has been expanding and cooling ever since.
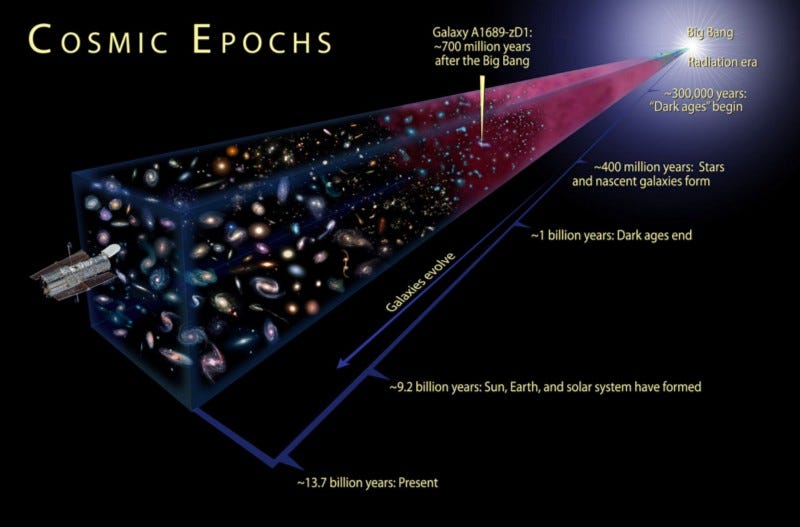
This means that in all directions that we look in space, we should see that same “leftover radiation” where neutral atoms formed for the first time. Prior to that time, some 380,000 years after the Big Bang, it was too hot to form them, as photon collisions would immediately blast them apart, ionizing their components. But as the Universe expanded and the light redshifted (and lost energy), it eventually became cool enough to form these atoms after all.
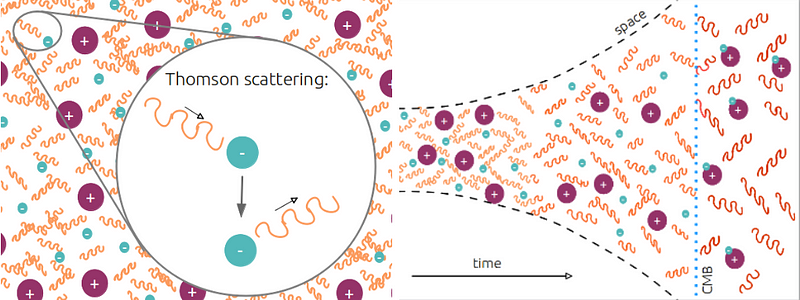
And when it did, those photons would simply travel, unimpeded, in a straight line until they finally ran into something. There are so many of them left today — a little over 400 per cubic centimeter — that we can measure it easily: even your old “rabbit ears” on your television sets with antennae pick up the cosmic microwave background. About 1% of the “snow” on channel 3 is the leftover glow from the Big Bang. Aside from those microkelvin imperfections, it should be uniform in all directions.
But the thing is, we don’t actually see a completely uniform 2.725 K background everywhere we look. There are slight differences from one region of the sky to the other that are actually very, very smooth. One “side” looks hotter, and one “side” looks colder.
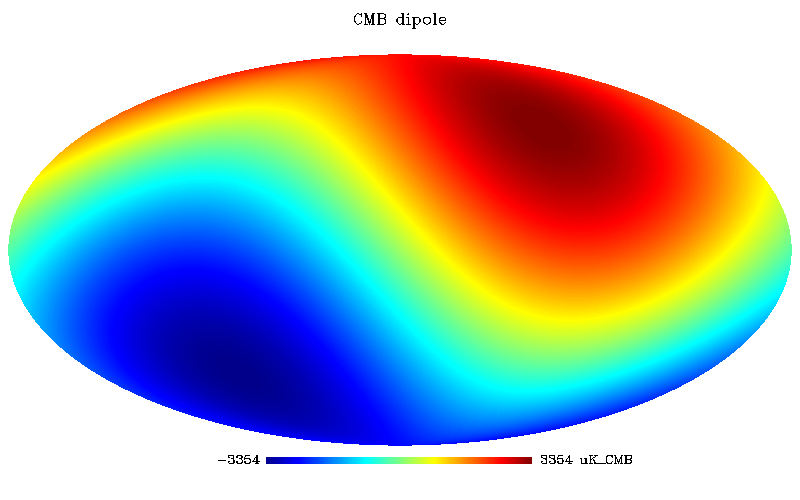
It’s actually by a fair bit, too: the “hottest” side is about 2.728 K, while the “coldest” is about 2.722 K. This is a larger fluctuation than all the others by almost a factor of 100, and so it might puzzle you initially. Why would the fluctuations on this scale be so huge compared to all the others?
The answer, of course, is that it isn’t a fluctuation in the CMB.
Know what else can cause the light — and the microwave background is just light — to be hotter (or more energetic) in one direction and cooler (or less energetic) in the other? Motion.
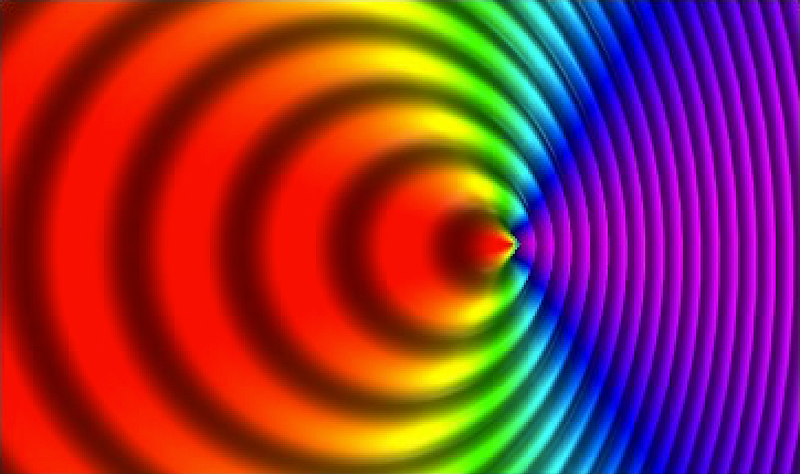
When you move towards a light source (or one moves towards you), the light gets blueshifted towards higher energies; when you move away from a light source (or one moves away from you), it gets redshifted towards lower energies.
What’s going on with the CMB is not that one side is inherently more or less energetic than the other, but rather that we are moving through space. From this effect in the Big Bang’s leftover glow, we can find that the Solar System moves relative to the CMB at 368 ± 2 km/s, and that when you throw in the motion of the local group, you get that all of it — the Sun, the Milky Way, Andromeda and all the others — are moving at 627 ± 22 km/s relative to the CMB. That uncertainty, by the way, is mostly due to uncertainty in the Sun’s motion around the galactic center, which is the most difficult component to measure.
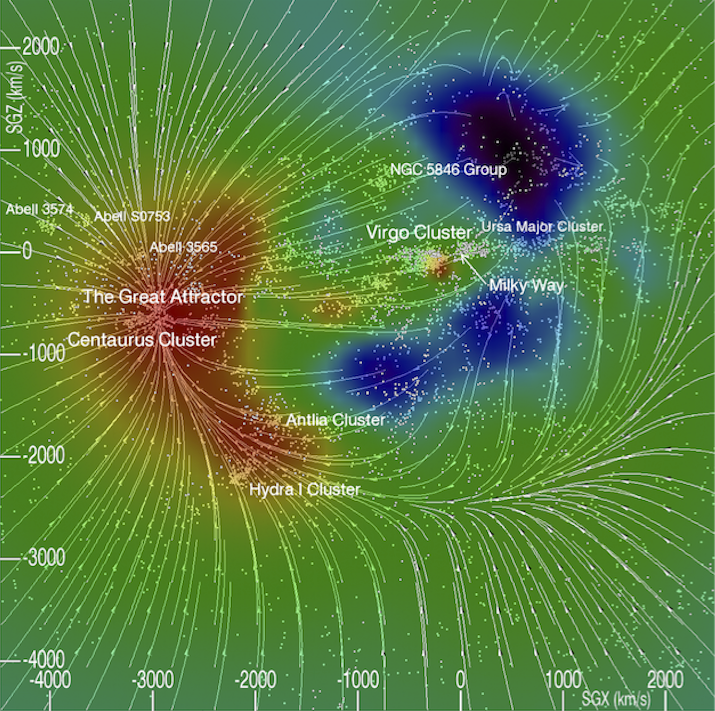
There might not be a universal frame of reference, but there is a frame of reference that’s useful to measure: the rest frame of the CMB, which also coincides with the rest frame of the Hubble expansion of the Universe. Every galaxy we see has what we call a “peculiar velocity” (or a speed atop the Hubble expansion) of a few hundred to a few thousand km/s, and what we see for ourselves is exactly consistent with that. Our Sun’s peculiar motion of 368 km/s, and our local group’s, of 627 km/s, matches up perfectly with how we understand that all galaxies move through space.
Thanks to the leftover glow from the Big Bang, we can not only find that we’re not a special, privileged place in the Universe, but we’re not even stationary with respect to the ultimate event in our shared cosmic past.We’re in motion, just like everything else around us.
This post first appeared at Forbes. Leave your comments on our forum, check out our first book: Beyond The Galaxy, and support our Patreon campaign!