Five Brilliant Ideas For New Physics That Need To Die, Already
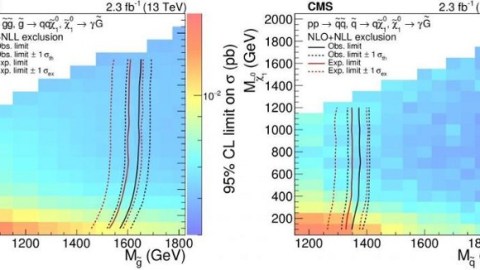
If your favorite theory made the list, you might want to consider a new favorite to bet on.
“Some people believe holding on and hanging in there are signs of great strength. However, there are times when it takes much more strength to know when to let go and then do it.” –Ann Landers
Plenty of people complain that science is too monolithic, that it’s a victim of “groupthink” and that people who come up with new ideas are routinely labeled as crackpots. But as much as novel ideas and theories are valued, creativity does not always equate to correct. The history of physics, in particular, is littered with ideas that were brilliant, creative, out-of-the-box, and completely wrong. From alternatives to relativity like “tired-light” to the “Steady-State” alternative to the Big Bang, to even the “Sakata model” alternative to the Standard Model, alternative ideas are important for comparing the actual Universe to our predictions and expectations.
But when the data comes in, it’s important to let those invalid ideas go. Hanging on to them only slows down the progress of science, forcing a field to fight a continuing battle whose outcome has already been settled. Unfortunately, as unbiased and objective as science itself may be, the scientists doing it are not. They fall in love with ideas, and when the data shows that they are bad ideas for describing the actual, physical Universe, it does not lead them to change their minds. It is the very reasoning that led Max Planck to quip:
“A new scientific truth does not triumph by convincing its opponents and making them see the light, but rather because its opponents eventually die, and a new generation grows up that is familiar with it.”
With that in mind, here are five brilliant ideas for new physics that have been very popular since the 1980s that continue to be popular today. But based on the evidence, it’s long past time for them to die.
1.) Proton decay: The Standard Model unified the electromagnetic force with the weak nuclear force, which led to the discovery of the W-and-Z bosons. What would happen if the strong nuclear force then unified with the electroweak force? A number of consequences were worked out for the first Grand Unification Theories, and one of them was surprising and compelling: a new, superheavy boson would exist that would mediate the decay of the proton. With an expected lifetime of around 10³⁰ years, the experiment was to gather some 10³⁰ protons (in the form of water), build a detector around them, and wait for a decay signature. While this experimental setup turned out to be a great neutrino detector, it didn’t see a single proton decay. By the present point in time, we’ve constrained the proton lifetime to be greater than about 10³⁵ years. Based on what we’ve seen so far, there’s no reason to think that the proton will ever decay.
2.) Modified gravity: When you look at rotating galaxies, you quickly find that the rate of rotation doesn’t match the amount of matter we can see. This is true not just for the matter in stars, but in gas, dust, plasma, and black holes, too. You could think to either add a new form of mass (e.g., dark matter) to make up for this discrepancy, or you could try to change the laws of gravitation by modifying them. Either way gives good results for individual galaxies. But then there are other things we look at:
- Large-scale structure formation,
- the fluctuations in the cosmic microwave background,
- the motions of individual galaxies within galaxy clusters,
- the amount and shape of gravitational lensing,
- the gravitational effects of merging galaxy clusters,
- the Sachs-Wolfe and Integrated Sachs-Wolfe effect, and
- the varied ratio of dark matter to normal matter (as inferred from motions of individual stars) on galaxies of different scales/sizes.
When we add dark matter, they all match. When we modify gravity, the modifications we need to make to solve one problem fail to solve the others. Many variants of modified gravity have been concocted over the past 35+ years; all fail to reproduce what we observe. It’s long past time to stop using the pipe dream of a successful modified gravity theory to argue against dark matter.
3.) Supersymmetry: Why is there such a difference in mass between the Planck scale (at 10^19 GeV) and the masses of the particles we know (peaking at ~10^2 GeV)? One idea to solve this problem is supersymmetry, which posits that for every one of the Standard Model particles, there should be a superpartner particle to protect this mass. While there are many elegant reasons to favor supersymmetry, the fact is that these particles should exist at approximately the same masses as the highest-mass Standard Model particles. With the advent of the LHC, we have determined that if these particles exist, they are many times heavier than the Standard Model particles, so much so that they would no longer solve the mass-difference problem. As a theory to explain this hierarchy problem, supersymmetry is completely dead.
4.) Technicolor: We all now know that the Higgs gives rest mass to the particles in the Universe. But what if there hadn’t been a Higgs; could there have been another way to get mass? There sure is: technicolor! Instead of the Higgs boson, additional gauge interactions provide another mechanism for giving mass to particles and, incidentally, avoid the hierarchy problem. But in theory, they should have produced new physics at the electroweak scale that wasn’t seen and flavor-changing neutral currents (a certain type of particle decay) that also isn’t seen. But the nail in the coffin was the experimental confirmation of the existence of the Higgs boson, rendering the idea of technicolor moot. Nevertheless, work continues on this discredited idea.
5.) WIMP-based dark matter: This one is reallycontroversial, because the evidence that dark matter exists is overwhelming. It had to be created somehow, and there are a whole slew of extensions to the standard model that produce particles that are massive, neutral, and that don’t interact through the electromagnetic or the strong nuclear forces. Somewhere in there should be a particle (or set of particles) responsible for the missing mass in the Universe: the dark matter. The indirect, astrophysical evidence for it is overwhelming. But for some reason, the overwhelming majority of direct detection efforts have focused on one specific, narrow sub-class of models: on weakly-interacting massive particles in a particular mass range: around ~10^2–10^3 GeV. All we have are constraints and lower limits, and ill-motivated models that haven’t panned out with their other predictions. The original motivation for WIMP-based dark matter, the so-called WIMP miracle, has been disproven. It’s long past time to invest in earnest in the search for other forms of dark matter.
The fact of the matter is that the best thing a new scientific theory can do is make predictions about what you can expect to observe in this Universe. When you go out there and look for it, that should be where the answer lies. If it isn’t, either you made a mistake somewhere, or you should abandon your theory. The tactic of altering your parameters, little-by-little, to insist that the key discovery is just out of reach of your experiments is a never-ending descent into wrongness. Unless there’s a new reason to take an interest in these ideas (among many others), such as new data, a new theory, or a previously-discovered mistake, continuing to look for new physics in these places will be no different from the drunk looking for his keys under the lamppost. Just because it’s the only place you can see doesn’t make it any more likely you’ll find them there.
Ethan Siegel is the author of Beyond the Galaxy and Treknology. You can pre-order his third book, currently in development: the Encyclopaedia Cosmologica.