Ask Ethan: How fast is space expanding?
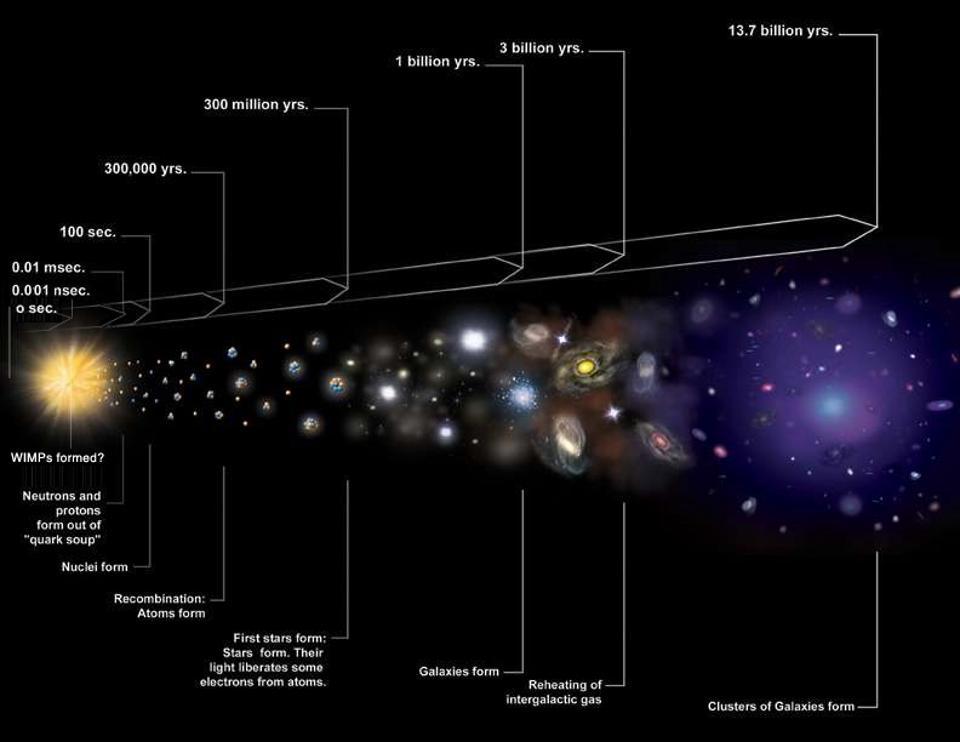
- It’s been nearly 100 years since we first discovered, observationally, that the Universe itself is expanding.
- However, we normally give the expansion as a rate, not as a speed, and yet some objects really do appear to be receding from us faster-than-light.
- If we decided to describe the Universe’s expansion with a speed, how fast would it actually be expanding? The answer isn’t just surprising, but outright alarming.
In one of the most monumental discoveries of the 20th century, we learned that the Universe is not simply a static, unchanging background, but rather that space itself expands as time marches on. It’s as though the very fabric of the Universe itself is stretching so that distant objects get farther and farther apart. We see this phenomenon in all directions and in all locations in space when we look beyond the Local Group. And yet, nearly 100 years after it was all worked out, it’s still a puzzling, counterintuitive phenomenon, even for experts in astronomy and astrophysics.
It’s only natural to wonder, if the Universe is expanding, how fast is the expansion of space? That’s what Darren Bobley wants to know, asking:
“Hi! Would you kindly help me to understand how fast space is expanding compared to light – in lay terms? (That mega-parsec idea is too heady for me.) Is it roughly 2x the speed of light? 100x times? Etc.”
It’s common, when we think about something expanding, to think in terms of speed. And we can do that if we so choose, but the answer is going to be different for every single object we look at. Here’s why.
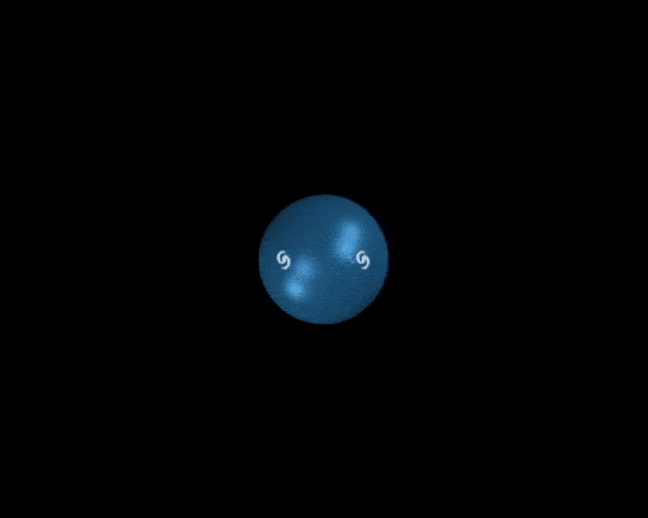
When you take any object that’s detectable through the science of astronomy, you’re always measuring some form of energy — usually light — that’s either emitted or absorbed by the object in question. Objects that are heated up to a certain temperature, like stars, will radiate light away with a specific spectrum that spans a range of wavelengths. Objects made of electrons bound to atomic nuclei, like atoms, ions, or molecules, will emit and/or absorb light only at specific wavelengths: the wavelengths that are dictated by the specific quantum transitions that are allowed to occur.
Since the laws of physics are the same everywhere in the Universe, including for other stars and galaxies, you might anticipate that those very same atomic and molecular transitions that we observe in laboratory experiments here on Earth would also, equivalently, appear for any astronomical object we look at. If there’s hydrogen there, you might expect to see the same emission and/or absorption lines in the spectrum of a distant object as you see on Earth.
A reasonable starting point to test this assumption would be to look at the Sun, and then to look at other stars (or collections of stars) to see how well it holds up.

When we break the light from our Sun up into the different wavelengths that compose it, we’re performing the science of spectroscopy. We can easily see the signatures of many different elements, and can identify the lines that are there with specific transitions in atoms with different numbers of protons in their nucleus.
Now, here’s the important thing that you must realize: when we look at the absorption and/or emission features of other objects in the Universe, they are made of the same elements that our Sun and Earth are made out of. The atoms they possess absorb and emit light with the exact same physics the atoms we know of do, and therefore, they emit and absorb light of the same wavelengths and frequencies that the atoms we interact with do.
But when we observe the light from other objects in the Universe, we pretty much never see the exact same wavelengths and frequencies that we see from the light generated in a lab or by our Sun. Instead, the spectral lines that we see are all systematically shifted from one another depending on which object we look at. Moreover, every single line that belongs to a particular object will be shifted by exactly the same factor when we view it.

There are three major factors that can cause such a shift, and in principle, every object can experience all three of these.
- There’s a difference in the gravitational potential between where the light was emitted and where it’s absorbed. When things move deeper into a gravitational “hole,” the light gains energy and gets shifted toward shorter wavelengths: blueshifted. When things climb out onto a gravitational “hill,” the light loses energy and gets shifted toward longer wavelengths: redshifted. This is predicted within General Relativity, as the curvature of space not only tells matter how to move, but tells light and all forms of radiation how to shift.
- There’s also the relative motion between the source and the observer: what we conventionally know as the Doppler shift. We most commonly experience it with sound. When a sound-emitting vehicle — like a police car, an ice cream truck, or a bass-heavy enthusiast — moves toward you, the sound you receive comes in at a higher pitch. When it moves away from you, the sound is lower in pitch. The same thing happens for light and for all waves: if the source and observer are moving toward one another, the light the observer sees will be blueshifted, where as if they’re moving relatively away from one another, the light the observer sees will be redshifted.
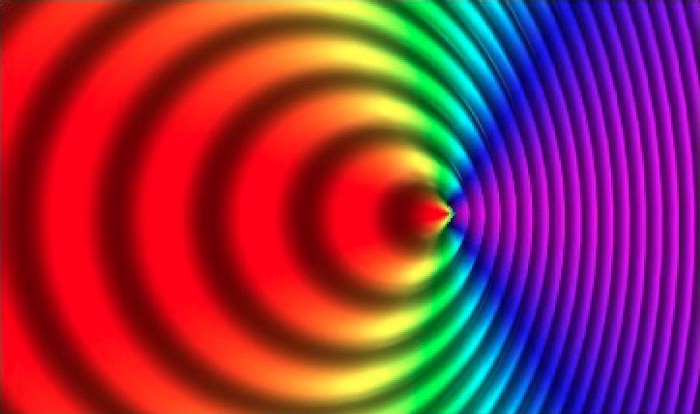
- And finally, there’s the effect of the expanding Universe. As light travels through the Universe, each individual photon — the quanta from which all light is composed — has a specific wavelength, and that wavelength defines the photon’s energy. If the Universe expands, the wavelength of that light gets stretched as well, causing a redshift; similarly, if the Universe contracts (which is also allowed, but isn’t what’s observed), the wavelength would have compressed instead, causing a blueshift.
If you want to understand how the Universe is expanding, then the task before you is clear. You have to observe a large suite of objects, in a variety of directions and at a variety of distances, and measure the cumulative redshift (or blueshift) of each one. You then have to map out the Universe to the best of your ability, and use that information to infer the effects of both gravitational redshift/blueshift and also what the effects of the motion of individual objects are relative to you. Whatever’s left, when you account for everything else, represents the effects of the expansion of the Universe.

So what do we learn when we do precisely this? A few things that might interest you, including the following.
- For objects nearby — within a few tens of millions of light-years — the effects of local motions dominate. You cannot reliably measure the expansion of the Universe solely by looking at objects in our own neighborhood.
- Objects that are gravitationally bound together, including stars, stellar systems, star clusters, globular clusters, individual galaxies, and even bound groups and clusters of galaxies, do not experience the effects of the expanding Universe.
- Gravitational redshift and blueshift, thankfully, is a largely negligible effect, showing up with a magnitude that’s universally much less than even 1% of the total measured effect.
- But on large cosmic scales, which translates into objects that are at relatively large distances from us (hundreds of millions, billions, or even tens of billions of light-years away), the expansion of the Universe is the only effect that matters.
That’s the best method for measuring how space expands as the Universe evolves over cosmic time: to look at all these objects scattered throughout the Universe, ignore the nearest ones, and to infer, on average, how the Universe is expanding.
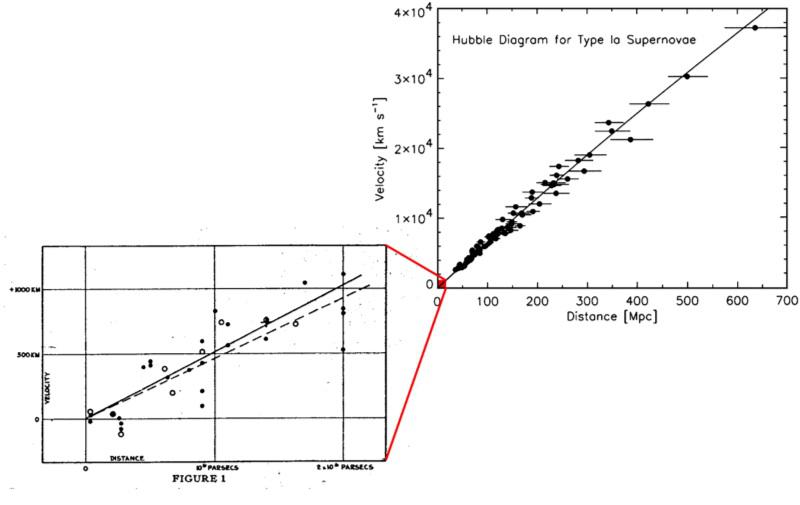
All the way back in 1923, Edwin Hubble measured the distance to the first galaxy beyond our own: Andromeda. Over the next few years, he not only measured the distance to many such galaxies, but combined them with previous observations of how the light from those galaxies was, overall, either redshifted or blueshifted. Working with his preliminary data, Georges Lemaître published a paper in 1927, drawing the conclusion that the Universe was expanding and measuring the expansion rate for the first time. The next year, independently, Howard Robertson did almost the exact same thing. But it wasn’t until Hubble himself, along with his assistant, Milton Humason, published their 1929 paper that the larger astronomy community began to pay attention to this groundbreaking result.
The most important part of this story isn’t the specific value that they measured; the most important part is understanding what it means that the Universe is expanding. It means that, for any two gravitationally unbound objects in the Universe, the space between them expands over time. When an observer at one of those locations looks at the other, they see the light generated in the other one appears to be redshifted by the time it arrives at their eyes. And the farther away the object is that they’re looking at, the greater the amount that the light appears redshifted.

When we’re asking the question, “How fast is the Universe expanding?” we are translating from one cause of redshift into another. We know that the expanding Universe causes redshifts; we know how two objects moving away from one another causes a redshift. If you want to translate the expansion of the Universe into a speed, that’s what you have to do: ask yourself, “Based on the redshift that I’m measuring due to the fact that space is expanding, how fast, in terms of a relative recession speed between the source and the observer, would things need to be moving to give the same value for a redshift?”
The answer, fascinatingly, is dependent on how far away that object is. Here are some examples.
- For an object 100 million light-years away, we infer a recession speed of 2150 km/s.
- For an object 1 billion light-years away, we infer a recession speed of 21,500 km/s.
- For an object 5 billion light-years away, we infer a recession speed of 107,000 km/s.
- For an object 14 billion light-years away, we infer a recession speed of 300,000 km/s: just about the speed of light.
- And for an object 32 billion light-years away, the current cosmic record for most distant galaxy, we infer a recession speed of 687,000 km/s: more than double the speed of light.
We can perform this computation for any object located any distance away, and for any particular distance, we get a unique recession speed.

This is the reason why, typically, we don’t talk about the expansion of the Universe as being a speed. Instead we talk about it as a rate: a speed-per-unit-distance. For every 3.26 million light-years away an object is, its light is redshifted by approximately an additional 70 km/s. For historical reasons, astronomers rarely use light-years, but rather more frequently speak in terms of parsecs, where a parsec is about 3.26 light-years. When you hear the term “megaparsec,” abbreviated Mpc, just translate that in your head into “about three and a quarter million light-years.” The most common way to express the expansion of the Universe is in terms of kilometers-per-second-per-megaparsec, or km/s/Mpc.
Today, we have multiple different ways of measuring the expansion of the Universe, and they all yield results that fall within a relatively narrow range: between 67 and 74 km/s/Mpc. There’s a lot of controversy concerning whether the true value is at the high end or the low end of that range, and whether there’s some new physical phenomenon at play that’s responsible for why different methods seem to yield different, mutually inconsistent results. At present, the best scientists in the world are looking for additional, superior data to try and learn more about this puzzle.

This means, when we put all the puzzle pieces we have together today, that there’s a specific distance away from us, around 14 billion light-years distant, where the expansion of the Universe pushes objects away at the equivalent of the speed of light. Closer than that distance, objects recede from us at speeds that are slower than light; farther away, they’re receding faster than light. In reality, these objects aren’t moving through the Universe at that speed at all, but rather the space between bound objects is expanding. The effect on the light is equivalent — it gets stretched and redshifted by identical amounts — but the physical phenomenon causing the redshift is due to the expanding Universe, not from the object speeding away through space.
One of the more fascinating aspects of this is that the expansion rate doesn’t remain constant, but rather varies depending on how dense the Universe is: as the Universe expands, it gets less dense, and the expansion rate therefore drops over time. Even with the presence of dark energy, some of the galaxies that are currently moving away from us faster-than-light are actually reachable by us, even if we were limited in our travels by the speed of light. Galaxies more than 14 billion light-years distant but less than 18 billion light-years away are still within our grasp, if we leave soon enough and travel quickly enough: containing about the same number of galaxies as there are located within 14 billion light-years of us. The Universe isn’t expanding at a particular speed, but for any object you look at, you can calculate how fast it’s zipping away from us. All you need to measure is how far away, right now, it actually is.
Send in your Ask Ethan questions to startswithabang at gmail dot com!