Earth isn’t alone: Venus once had plate tectonics, too
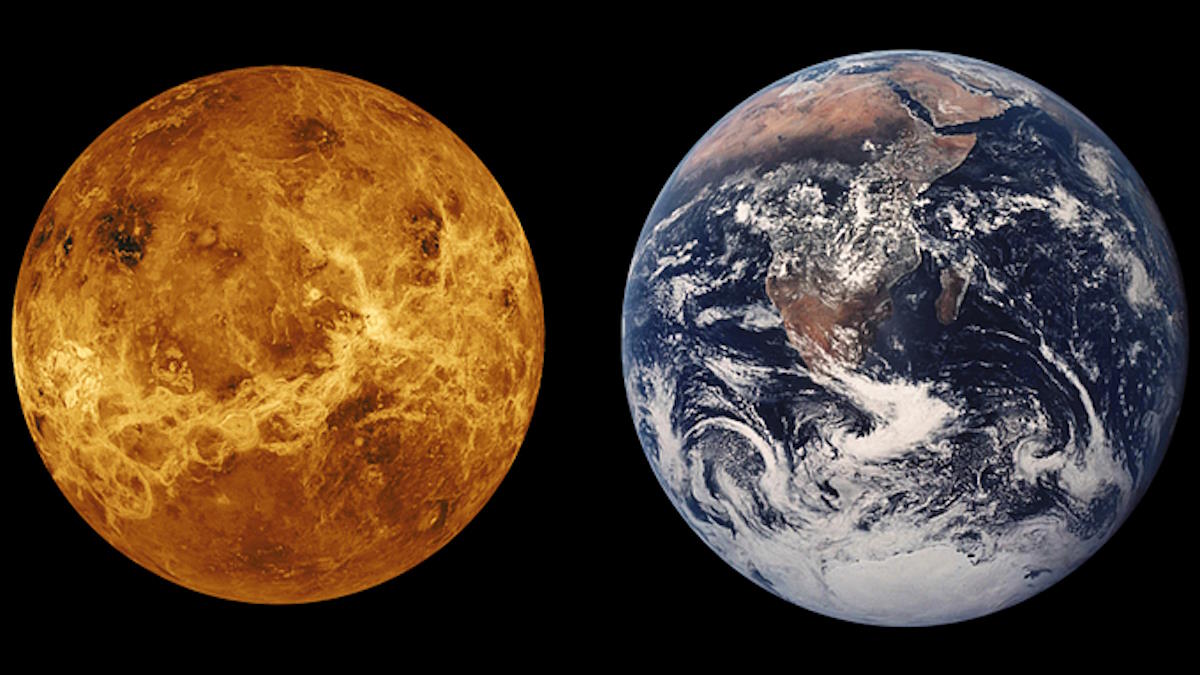
- Among the planets in our Solar System, only Earth has active plate tectonics today. Some outer moons may have ice-plate tectonics, but Mars and Mercury are both uni-plate planets.
- While Venus appears to have only one plate as well, its surface is relatively young, with recent volcanic activity wiping out any trace of its tectonic history during the early and intermediate phases of our Solar System.
- However, a new study looks at the link between Venus’s atmosphere and interior, and concludes that an ancient phase of plate tectonics, lasting at least 1 billion years, must have occurred in its past.
When it comes to the worlds present in our Solar System, we need look no farther than our closest planetary neighbors to realize just how good we have it here on Earth. On Earth, we have stable, life-supporting conditions here on the surface, with a thin but stable atmosphere, liquid-water oceans and the right temperatures and pressures to support them, and active plate tectonics that gradually causes mountains, oceans, islands, and other continental and sub-ocean features to grow, shrink, and otherwise evolve. None of the other planets in our inner Solar System, as far as we know, possess any of these features.
While Mars is small, distant and cold and Mercury is scorchingly baked and atmosphere-free, Venus represents an interesting case of an alternative pathway for an Earth-sized planet. Although Venus is about the same physical size as Earth and only somewhat closer to the Sun, any Earth-like conditions once present on it have been left in the distant past. Today, Venus has a thick, cloud-rich atmosphere of dense greenhouse gases, with surface temperatures hot enough to melt lead and tremendous evidence for copious volcanic activity. Although it has no active, moving tectonic plates today, a new paper argues that Venus, like Earth, once possessed active plate tectonics. Here’s the compelling case for Venus’s early phase of plate tectonics.
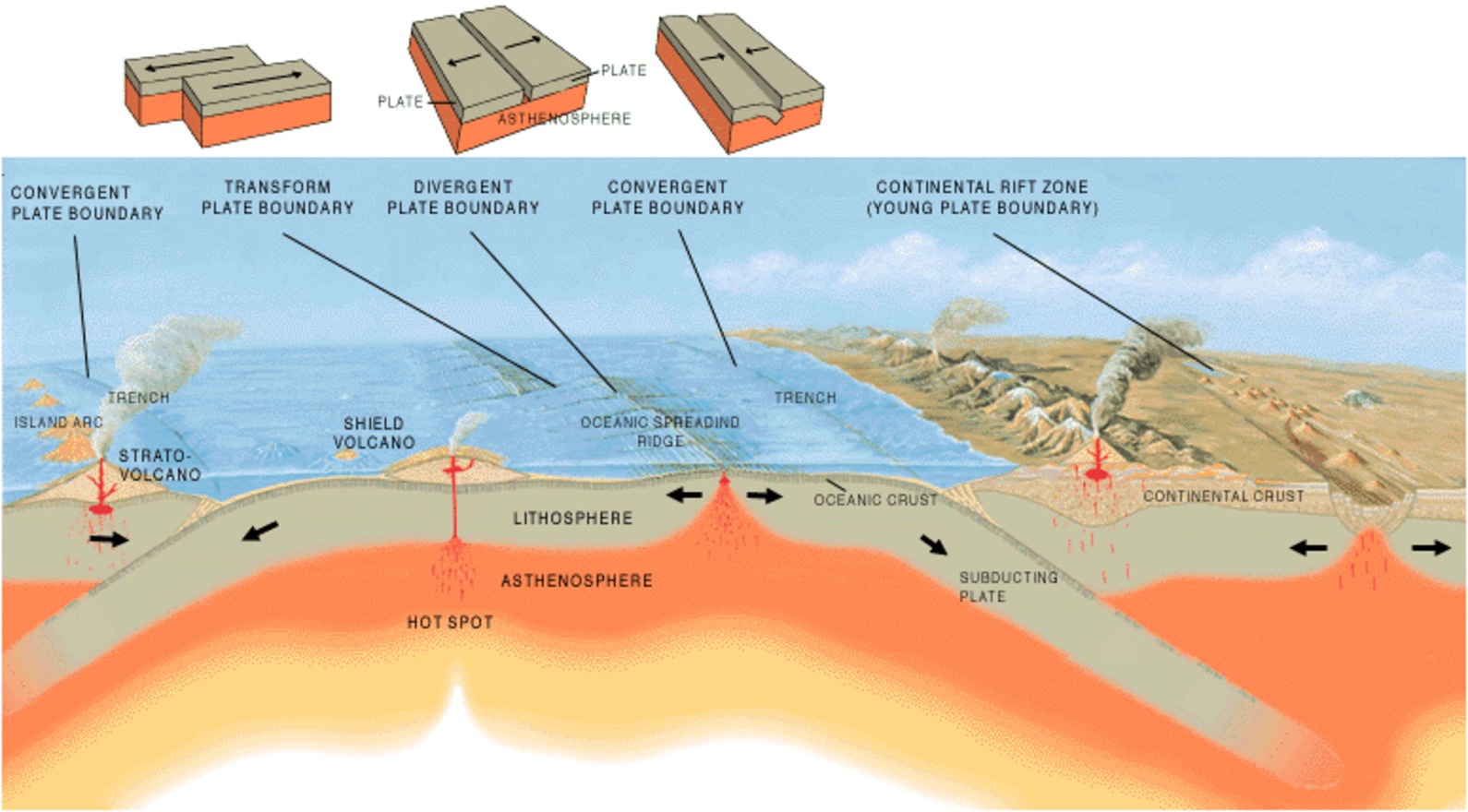
There’s a lot we still don’t understand about plate tectonics, both on Earth and elsewhere in the Solar System. On Earth, we understand that our planet’s lithosphere — the combination of our crust and the mantle’s topmost layer — are fragmented into a series of plates, which in turn:
- collide,
- spread apart,
- uplift,
- and subduct,
among other behaviors, creating a rich diversity of surface features. These can include new land masses, large mountain ranges, and can even recycle old sections of the Earth’s surface back into the planet’s interior.
This is certainly true of modern-day Earth, but did our planet always possess the plate tectonics we’re accustomed to at present? That question is far more uncertain, with no consensus within the community as to whether plate tectonics are basically as old as the Earth, whether they began hundreds of millions or even 1-1.5 billion years after Earth’s formation, or whether they only came about relatively recently. From looking at other worlds in the Solar System with ice-plate tectonics, such as Europa and possibly even Pluto, it may be that the combination of internal heat along with the lubricating effects of water are what enable the plate tectonic behaviors that are present on Earth today.

It’s a pretty good bet that Mercury, our Moon, and Mars all never had plate tectonics on them, for a variety of reasons. For Mercury and the Moon, the weight of evidence from craters and cratering rates support the notion that no tectonic activity was ever present on them, and Mercury itself even lost most of its mantle early on in our Solar System’s history; it has the largest metallic core of any planet relative to its size. For Mars, meanwhile, the fact that it has only a few notable volcanoes — and that the volcano-forming hotspots beneath its crust are still in the same location they were 3+ billion years ago — constrains any plate tectonic scenario to its most extreme early stages.
It’s enough to make one wonder what it is about Earth, if anything, that makes our planet special? These other worlds show no evidence for plate tectonics in either recent or ancient history, and in fact may all have been uni-plate planets for all of planetary history in our Solar System.
But in order to even consider that Earth is something special here, we need to consider the curious case of Venus. Venus is comparable to Earth in terms of both mass and physical size, and is currently volcanically active today, with recent evidence emerging from the Magellan mission suggesting that volcanic eruptions are still occurring there, and creating local resurfacing events, at present.
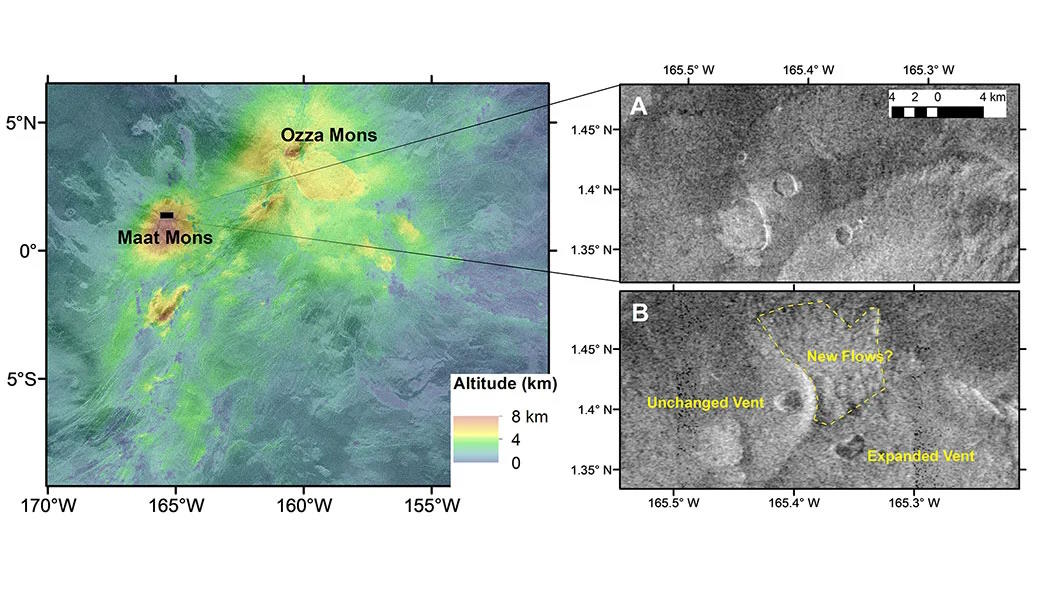
However, when we examine the surface of Venus and look at the cratering rates on the surface of that world, we can conclude that 80% or more of the Venusian surface is young: only about 1 billion years old at the oldest. This means that for most of Venus’s history — the first 3.5 billion years of its planetary history — we have practically no information about what its surface was like.
How, then, can we be expected to draw conclusions about Venus’s history and whether it had plate tectonics or not, particularly when Earth’s early tectonic history, even with all the data we have about our planet, is still in doubt?
It’s a challenging proposition, to say the least. Keep in mind that, unlike the Earth, for Venus, we have:
- no rock samples from it,
- no high-resolution mapping of its surface,
- and only a limited geologic record,
- that only goes back to cover approximately 20-25% of the planet’s total history.
Even with that, Venus, as we observe it, shows a pattern of tectonic deformation, and may indeed have a globally fragmented and mobile lithosphere, despite not having Earth-style plate tectonics right now.

The answer, as counterintuitive as it may seem, might be something we can learn by examining Venus’s atmosphere, rather than anything occurring on (or even beneath) Venus’s surface. One of the key reasons this may be possible is that the interior evolution of Venus is directly related to the evolution of Venus’s atmosphere, with the gases and atomic components of gases that make up its atmosphere primarily originating from within the interior of the planet itself.
If you can take a look at what Venus’s atmosphere is like today, for example, and compare it with various models that take into account the interplay of Venus’s atmosphere with:
- the thermal/heat contents of Venus’s interior,
- the chemical evolution of Venus’s interior,
- and the tectonic evolution of Venus’s crust and upper mantle,
then it may become possible to look at the atmospheric contents of Venus today — including the quantities of gases like molecular nitrogen, carbon dioxide, and anything that contains sulfur — and consider which models are consistent with the data and which ones conflict with it. That’s precisely what this new paper seeks to do.

We know that Venus, as our starting point, is nearly as large and massive as Earth, and is composed of very similar materials on the inside to our own planet, as evidenced by Venus’s similar density to Earth. (The comparison is 5.24 grams per cubic centimeter for Venus, overall, versus 5.51 for Earth.) If that’s the case, then much like Earth, Venus must have formed with a substantial amount of internal heat, and should also possess similarly sized layers to Earth:
- a solid iron/metal inner core (and, perhaps, an innermost core within that as well),
- surrounded by a liquid iron/metal outer core,
- with an enormous solid-rock mantle outside of that, composing most of the planet’s volume,
- followed by a thin crust.
With all of that heat inside all of those layers, it’s a near-certainty that the mantle itself is going to have an enormous temperature gradient within it, and that temperature gradient is going to lead to some sort of convective (or churning-over) behavior.
However, the big uncertainty involved with Venus, which also concerns uncertainties when we consider Earth’s ancient past, is just what type of convection occurs within it. Does the whole mantle convect? Does the mantle convect in separate layers, and do those layers mix? And does any part of the mantle that convects include the surface/crust, allowing the surface to participate in what’s called “mantle overturn” or not?
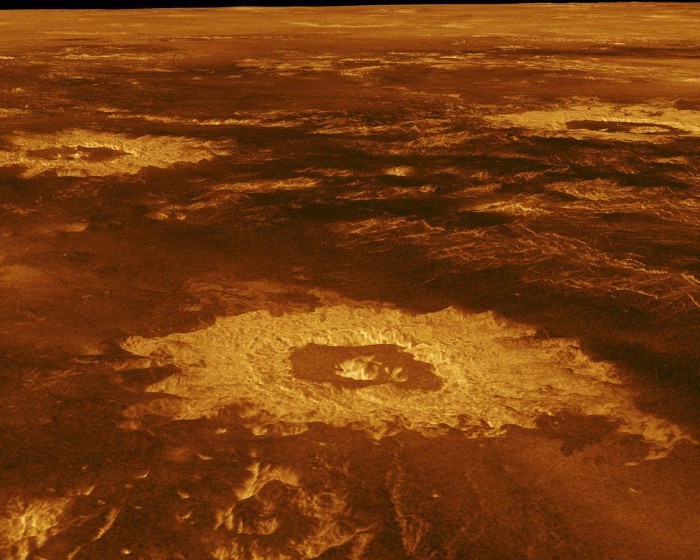
Here on modern Earth, we have upper-mantle convection (which includes the overturn of Earth’s crust, along with the upper layer of Earth’s mantle), lower-mantle convection (which involves the asthenosphere but not the lithosphere), and also whole-mantle convection, all occurring simultaneously within our planet.
However, on modern-day Venus, it’s thought that this is not occurring. Instead, present-day Venus has what the geophysics community calls a stagnant lid, which means that the topmost layer of the planet — the lithosphere that includes the crust and the uppermost mantle — is cold, solid, and relatively stable and immobile. This leads to stagnant lid tectonics, which includes virtually no horizontal (side-to-side) motions, which means that even if the lithosphere is fragmented into plates, these plates don’t migrate across the planet’s surface, but rather stay in place.
As you may have guessed from noticing that hot lava flows, but that colder rocks do not flow, a cold, solid lithosphere would be very strong and not easy to break apart, which would mean that any lower-mantle convection wouldn’t affect the stagnant lid very much at all.

Today, Venus very much has a stagnant lid. Early on in Earth’s history, before achieving our present state of active plate tectonics (which is sometimes called “mobile lid” or “active lid” tectonics), we may have also had a stagnant lid on our world; it was recognized as early as 1989 that the stagnant lid regime is a very stable configuration, and may have even applied to Earth long ago.
But did Venus always have a stagnant lid? For nearly 30 years now, it’s been pretty clear that the data we’ve acquired only allows us to say that the stagnant lid phase of Venus is at least around 500 million years old, but that early on, this may not have been the case. Just as Earth may have switched “modes” in its ancient past, so too, may have Venus, as its young surface offers little in the way of constraints on its early properties.
But this is why Venus’s atmosphere is so interesting: because it’s thick and massive, but not so thick and massive as to have turned Venus into a mini-Neptune-like world. At its surface, Venus has 93 times the atmospheric pressure as there is at Earth’s surface, with a whopping 4.8 × 1020 kilograms of mass composing the Venusian atmosphere. (For comparison, that’s about 40% as massive as all of Earth’s water stores, including the oceans, combined.)
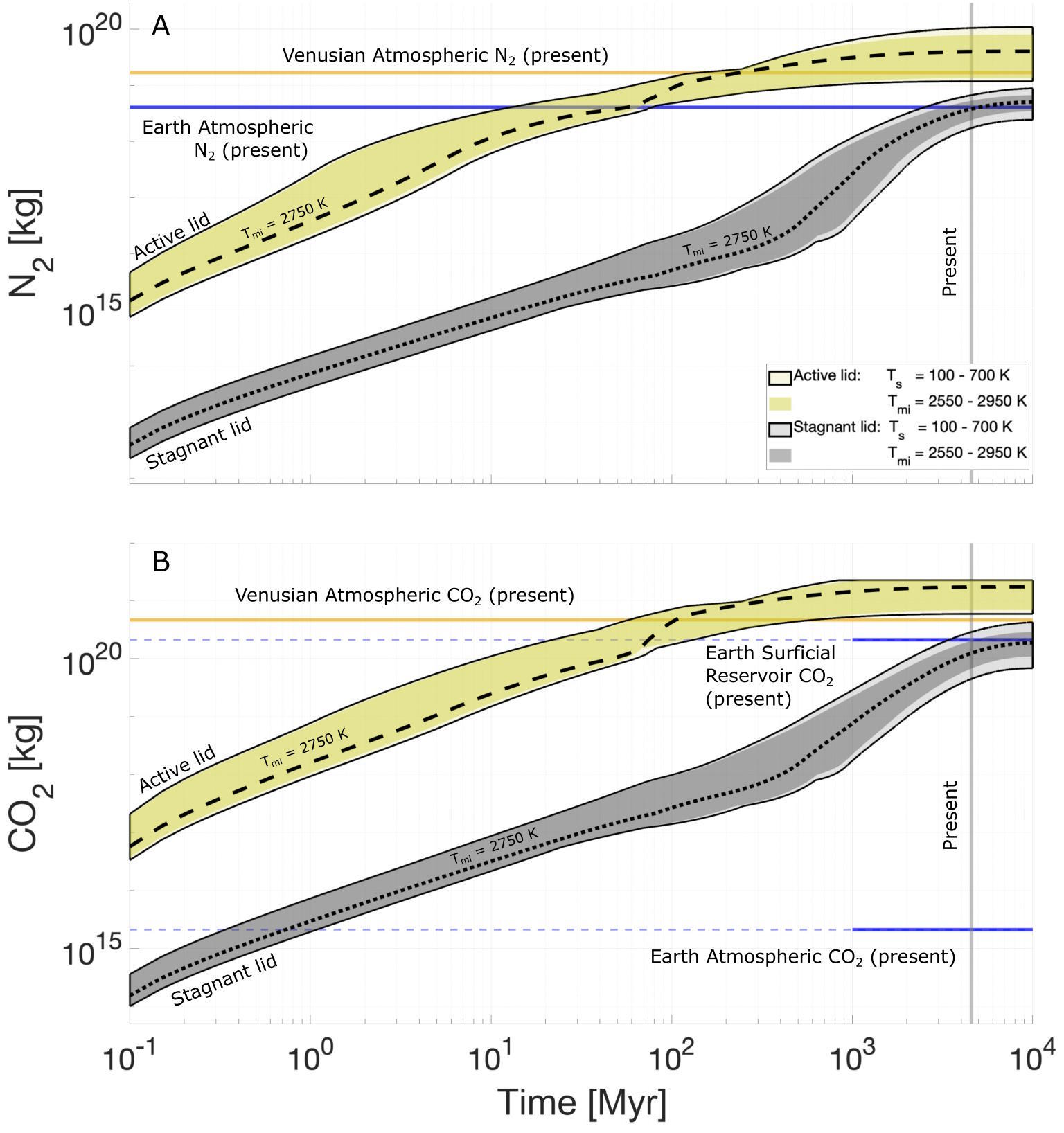
Venus’s atmosphere is made up mostly of carbon dioxide (96.5%) and nitrogen (3.5%), with the next most abundant component, sulfur dioxide, coming in at only 0.015%. The big question that the authors of the latest study looked at is, based on a realistic thermal model for early Venus’s interior and either stagnant or active lid tectonics, how much nitrogen and carbon dioxide would have been produced.
If there was a stagnant lid the entire time that Venus has been around, there’s no way to arrive at the present amount of nitrogen, or the present amount of carbon dioxide, or the present amount of total atmospheric pressure that Venus exhibits today.
On the other hand, if there was an active lid for too long — for about 2 billion years or more — on Venus, then you would actually wind up overproducing the gases we see: particularly carbon dioxide. It’s an implausible scenario that Venus would have been a planet with active lid tectonics for most of its history.
Instead, what you want to do is match all three observables: nitrogen abundance, carbon dioxide abundance, and total atmospheric pressure, even when you account for solar and planetary evolution and how they affect a planetary atmosphere over time.
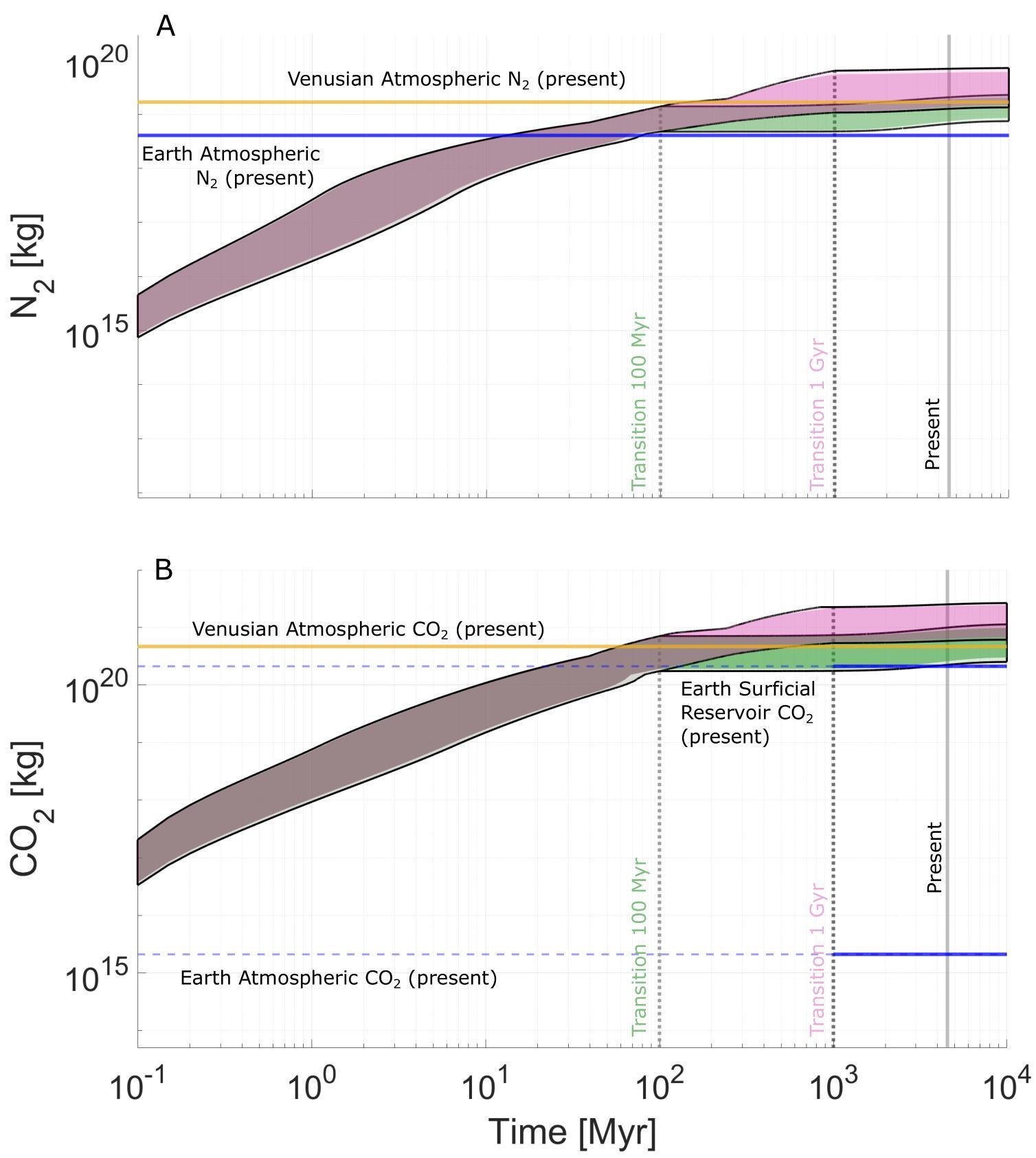
The scenario that best matches the data, according to this latest study, is one where Venus possessed an early, active phase of plate tectonics (active lid tectonics) that produced large amounts of nitrogen and carbon dioxide early on, lasting for around the first ~1 billion years, and possibly slightly more, of Venus’s history. This had to have been followed by a transition from active lid to stagnant lid tectonics: what the authors call a “great climatic-tectonic transition,” where the stagnant lid mode then persists up through the present day. Although the stagnant lid mode still includes volcanic activity, the outgassing rates are greatly reduced over the active lid scenario.
This paints a novel but grim picture of how Venus came to be the way it is today. Perhaps, early on, the active lid tectonics released large amounts of carbon dioxide gas, and that gas may have accumulated rapidly in the atmosphere. If you can’t recycle, lose, or sequester that carbon dioxide fast enough, it leads to an extreme greenhouse state, and that’s a fatal condition for any surface-dwelling life. However, if life arose early enough on Venus, before the runaway greenhouse process, it’s still possible that life’s vestiges still exist in Venus’s atmosphere today, and could potentially be detected by probing carbon-and-nitrogen isotope ratios. Whether there was life or not, the DAVINCI mission, among others, will be able to measure the nitrogen-15 to nitrogen-14 ratio, which will help determine how much atmosphere was lost to space over Venus’s history.
But most importantly, in the history of plate tectonics, we know that Earth is no longer alone in the Solar System: Venus once had active plates, too, and its atmosphere, not surface, is what lets us know for sure!