How to save Earth from a doomsday impact as seen in “Don’t Look Up”

- Although there are many existential threats to human life on Earth, one unlikely but catastrophic scenario involves a major impact of an asteroid of comet on Earth.
- Just as the non-avian dinosaurs, and some ~70% of all species, were wiped out by a massive impact 65 million years ago, a similar impact could bring an end to humanity and much, much more.
- The movie Don’t Look Up highlighted our social and political failures to act or care, but the capabilities to save Earth from such a threat are right within our grasp.
As our planet orbits around the Sun, relics from our Solar System’s ancient past constantly exert tiny, almost imperceptible gravitational forces. While we’re normally aware of the largest objects — the Sun, planets, moons, and the largest asteroids and Kuiper belt objects — the uncomfortable truth is that there are millions of objects out there that pose an existential threat to most life on our planet. If an energetic enough impact — from an asteroid, Kuiper belt object, Oort cloud object, or even an interstellar object — were to collide with Earth, it could be the demise of not only our entire species, but of more than half of all species on our planet.
65 million years ago, some ~70% of all species on our planet went extinct when a large asteroid about 5 kilometers wide slammed into what is now known as the Gulf of Mexico, creating Chicxulub crater and wiping out every non-avian dinosaur on the planet. As recently depicted in the film Don’t Look Up, it’s always possible that, all of a sudden, a new object will be discovered that happens to be on a direct collision course with Earth.
If such a discovery were made, what would our response be? Although you might think the same answer would apply regardless of the specific scenario, it turns out that the best way to “save the Earth” is highly dependent on the type of threat we face. Here’s what you should know, including what questions you should be asking yourself should you ever find yourself tasked with saving the planet from certain doom.

What makes an impact potentially dangerous?
There are lots of factors that go into determining just how hazardous an impact is going to be. The location of impact could make a big difference, particularly for a smaller impactor: one that hits the North Pole will result in far less loss of life than one that strikes New York City. One that comes in at a very shallow angle, grazing the atmosphere, will lose substantial amounts of speed, and therefore energy, before impact compared to one that strikes almost perpendicular to the Earth’s surface. Additional factors, such as composition, density, size, and whether it’s been tidally disrupted or not, can all play a role as well.
But the overwhelming factor is simply energy: good old kinetic energy, or ½ times the mass of the impactor times the velocity (with respect to Earth) squared. That energy, for the most part, will get transferred into the Earth, causing effects like:
- creating an extraordinarily powerful blast wave,
- including atmospheric, oceanic, and crustal shocks,
- with tsunamis, earthquakes, and possibly volcanic activity accompanying it,
- kicking up a large amount of debris that will effectively block the Sun planet-wide for weeks,
and much more. If it were as energetic as the impact that wiped out the dinosaurs — the equivalent of billions of atomic bombs going off all at once — the catastrophe that would ensue would be comparable to what our planet went through during the previous great mass extinction event.

How “bad” would it need to be to wipe out humanity?
You have to understand that the two components that determine a potential impactor’s energy, mass and velocity, are intimately related to the object’s size and its location of origin. Asteroids that originate from the asteroid belt tend to move relatively slowly with respect to the Earth: in the ballpark of ~10-20 km/s or so. Comets from either the Kuiper belt or the Oort cloud tend to move about three times as fast as a similarly-massed asteroid does when it passes by Earth, and interstellar objects, although more rare, can travel four to eight times as fast as an asteroid. Remember, since energy scales as ~velocity squared, this means that a comet could only be ~11% as massive as an asteroid, or an interstellar object could be just ~2% as massive, and still have the same destructive effects.
- City-killers are common; we seem to get struck by one of those every century or few centuries, with 1908’s Tunguska event and the impact that created Arizona’s Barringer crater being two such examples. These events arise from objects that are ~10-100 meters across or less and are about as energetic as a single atomic bomb, causing an equivalent amount of damage: mostly localized to the region of impact.
- Planet-killers, like the Chicxulub crater impact, occur rarely: on average, every few tens-of-millions of years. Billions of times as energetic as a city-killer, they’re all well over ~1 km in size. (Remember, an object that’s 10 times the diameter of another will have ~1000 times the volume, and more than ~1000 times the mass.) Although planet-killers are rare, they are in many senses the most dangerous objects of all.
- There’s also an intermediate range of objects that are bigger than city-killers, but won’t necessarily have catastrophic effects that extend to the whole planet. These regional-level impacts could have damage extending across multiple countries or even multiple continents, and could cause oceanic tsunamis that wipe out coastal cities entirely. These occur, typically, on timescales of tens of thousands of years.
Categorizing these different classes of impacts was one of the major advances of implementing the Torino scale.
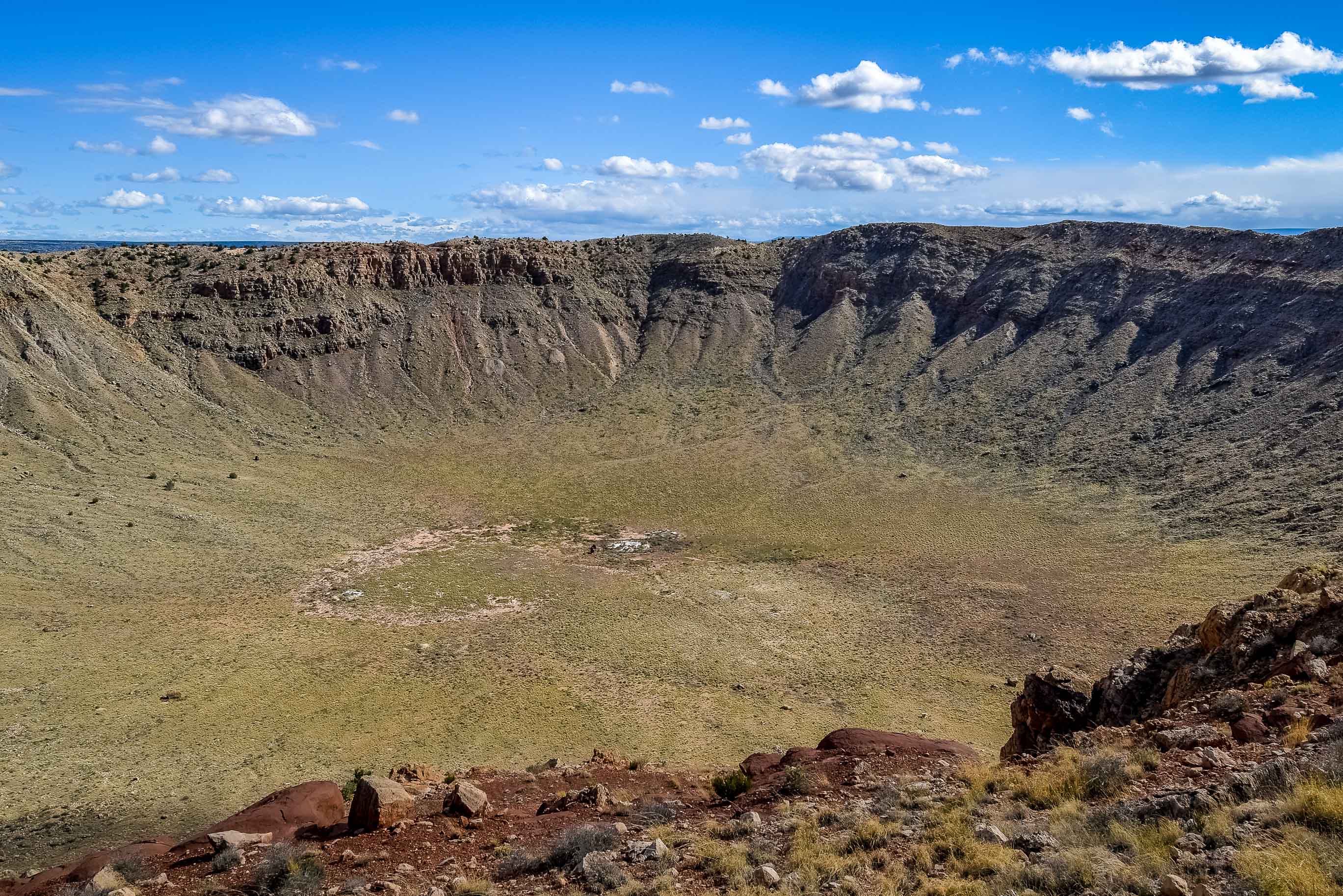
Three keys to mitigation
If we want to adequately defend our planet from such a threat — after all, it’s quite clear that the dinosaurs didn’t have a sufficient planetary protection program in place — there are three major keys that we have to address. They are:
- maximizing the time from hazard identification to impact,
- minimizing the time it takes to intervene: i.e., to reach the hazardous object,
- and to intervene in the right way, either deflecting the object so that no collision takes place or minimizing the damage done by whatever collision(s) actually occur.
This is one of those endeavors that we absolutely have to prepare for in advance. Unless we build, create, utilize, and maintain a system to identify these objects well before the threat arrives, no mitigations can take place. Unless we develop the technology to reach and rendezvous with a potentially hazardous object, swiftly and with the necessary trajectory, we’ll be unprepared for whenever the inevitable comes, and we’ll simply have to absorb the damage. And unless we prepare, in advance, to take the optimal pathway when it comes to preventing an otherwise catastrophic impact, we run the risk of facing the same fate that all land animals larger than about an iguana suffered some ~65 million years ago: global extinction.

The first step in any of this is identification. In an ideal world, we’d have a dedicated set of surveys to track and measure all objects above, say, ~10 meters in size that were gravitationally bound to our Solar System, including asteroids, Kuiper belt objects, and the Oort cloud. Of course, there’s a tremendous problem with that: we can only see objects that are bright enough to appear in our telescopes, and smaller, more distant objects are harder to see.
How much harder to see are they? The answer surprises most people. An object that’s half the diameter will only reflect one-quarter of the light as another, as surface area is proportional to the radius of an object squared. But an object that’s twice as far away as another will appear only one-sixteenth as bright! Why’s that? Because light spreads out like a sphere. If an object is twice as far away, then it only receives a quarter of the light compared to the closer object. That light then has to travel back from the object to Earth, where again, the farther object is twice as far away, so its apparent brightness is further reduced by another factor of one-quarter.
Moreover, determining an object’s orbital trajectory requires multiple observations over a time where it has changed positions significantly. For an object that’s in the asteroid belt, a few days or weeks might be enough, but for one that’s far off in the Kuiper belt, you need independent sets of images over much longer timescales — perhaps even multiple years — before you can accurately determine its orbit.

Assuming that we can determine an object’s trajectory, we can anticipate when, precisely, it will be at risk (or, if no intervention is taken, when it will certainly) impact the Earth. At that point, the next step is to launch a mission to intercept the object as quickly as possible.
That doesn’t, however, necessarily mean simply launching a space probe on a direct collision course at maximum speed toward the object we’re interested in. Right now, in fact, we don’t even have the active technology capable of achieving that end; we’d have to plan out a trajectory that took advantage of gravitational assists from other objects, like the other planets in our Solar System, to reach a target that was located in the outer Solar System.
Moreover, you don’t necessarily want to arrive moving extremely quickly relative to the object; you may want to make a “soft landing” on it, which requires adjusting your speed to match that of the incoming hazard. This is not a trivial problem, and in the history of the Solar System, we’ve only had a controlled rendezvous and successful soft landing on two objects, via the Hayabusa and Hayabusa-2 missions. Although we’ve rendezvoused with asteroids and comets previously, including Comet Halley, near-Earth asteroid Eros, and Comet 67P/Churyumov–Gerasimenko, no successful landings took place.

And finally, assuming you do get there with precisely the parameters you’ve designed, you still have to enact the proper, and more importantly, sufficient intervention. It’s important, at this point, to recognize that there is no one-size-fits-all intervention that will work for every scenario. Rather, which intervention we should take depends on four major factors.
- Composition. An object that’s ice-rich, low-density, and covered in volatiles is a very different case study than a solid, rocky body. An object that’s held together as a loose collection of rubble, like the near-Earth asteroid Itokawa, would need to be handled much more delicately than a more tightly-bound object. This factor must not be ignored.
- Trajectory. A massive object moving at a low speed presents a very different scenario to a less massive object moving very rapidly. Even though they may have the same momenta as one another, the energy, and the path toward optimally altering their orbits, may be very different for them both. Additionally, an object that will be perturbed by a giant planet, like Neptune or Jupiter, will be much easier to course-correct if we can reach it before that interaction, rather than afterwards.
- Mass. This factor, all on its own, informs how difficult it is to change a potentially hazardous object’s speed. The very solution that works for a less massive object might be unworkable for one with the exact same trajectory but a slightly heavier mass.
- Time remaining. This, again, is vital. If you need to alter an object’s trajectory by ~6,000 kilometers (roughly the radius of Earth), then if you get there with a year remaining, you only need to change its speed by 19 cm/s. If you get there with a month remaining, you need to change its speed by 2.3 meters-per-second. And if you don’t get there until there are 24 hours left until impact, you’ll need to change its speed by 70 m/s, or over 150 miles-per-hour.
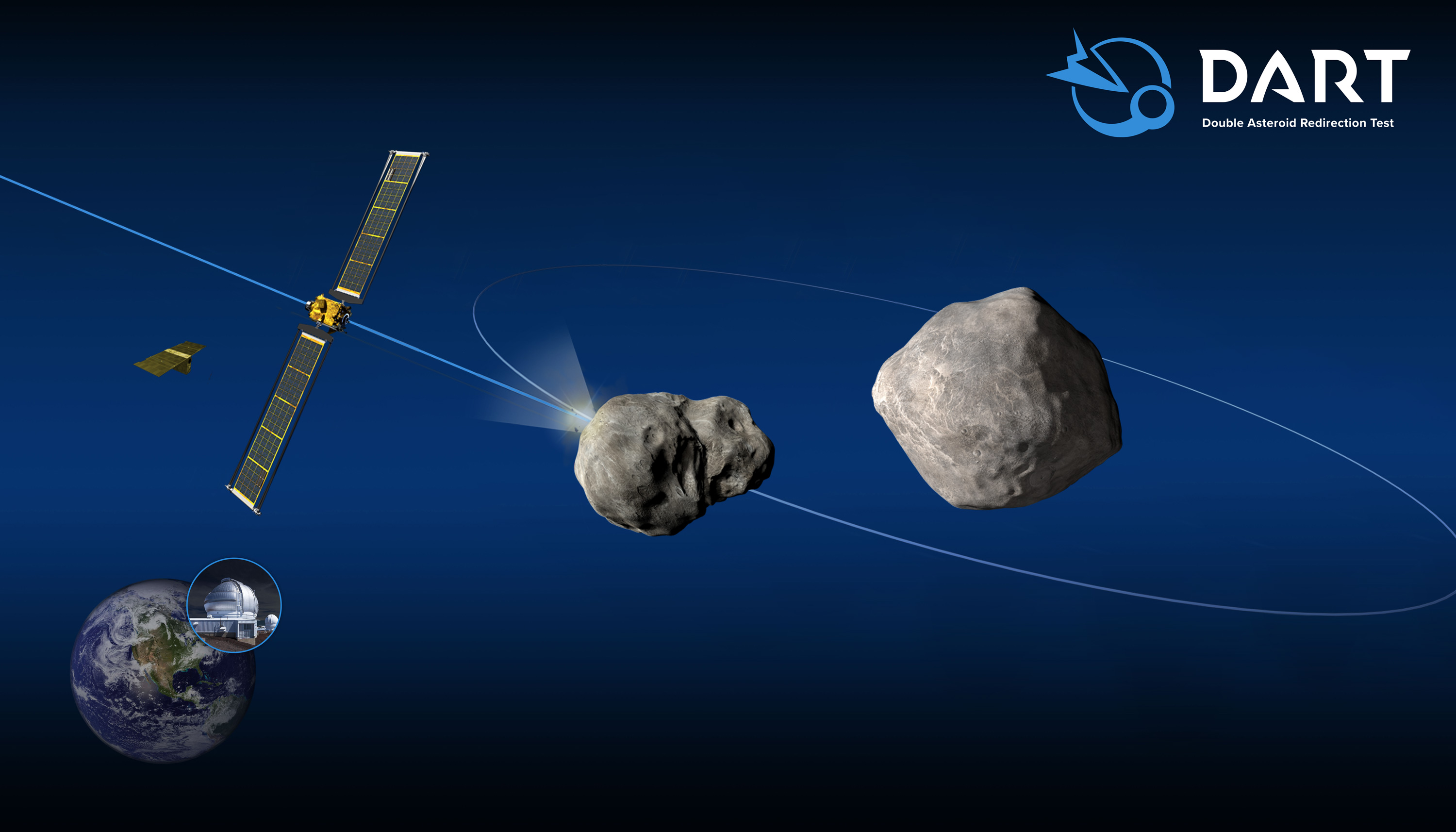
Options for saving the Earth
So let’s say we take all of those steps. We identify the potentially hazardous object before it hits us, we prepare and launch a mission to rendezvous and (if necessary) dock with the object, and then we’re ready to intervene. What should we do?
If you watched Don’t Look Up, you’ve seen the strategy of carving the object up into smaller components, with the idea that the Earth could more easily survive many small impacts than a single, large one. This idea, unfortunately, is simply stupid. If you hit Earth with 100 small masses with the same speed versus 1 large mass with that exact speed, you are imparting the exact same amount of energy into the Earth. Just as when Comet Shoemaker-Levy broke apart and all ~2 dozen of its components hit Jupiter, the level of the catastrophe would remain unchanged; it would simply be more evenly distributed around the globe.
You might next think to make a direct kinetic impactor, similar to NASA’s recent DART mission. If the object were solid enough and low-enough in mass, this is a potential option, as the small amount of momentum carried by the spacecraft could, in principle, change the course of the hazardous object by a great enough amount, particularly if it arrived early enough, to divert it away from Earth. This would be less effective for rubble-pile objects, however, and would be virtually unworkable on either short timescales or for very massive objects.

If you have the luxury of time, attaching a passive thruster — such as one that’s driven by ions — to your object can provide a gentle, sustained push. If you can get there early enough, this slow application of momentum, particularly if we can control its direction, could be enough to slowly nudge a hazard out of the way. The heavier the mass, however, and the less time you have, the more thrusters you’ll need.
And there’s always the Bruce Willis option: a nuclear device. If you have a rubble pile asteroid or a volatile-rich object like a comet, you’ll want to detonate the nuclear device in space near the object, otherwise you’ll simply blow a hole in it. By detonating it away from the object, you serve three purposes: you keep the object intact as a single entity, you change its momentum in the direction opposite to the location of the explosion, and you don’t fill the hazard with radioactive materials, which will then come back to Earth. If the object is solid and massive, however, the exact intervention becomes murkier; it depends on the coupling of the energy of the device to the motion of the object.
Although there are no existential threats headed our way, that we know of, over the next century, there is an object worth keeping an eye on: Comet Swift-Tuttle, the parent body of the Perseids. It’s larger, more massive, and faster than the object that created Chicxulub crater; if it were to strike Earth, it would impart some ~25-30 times the energy of that impactor. In 4479, there is a non-negligible chance, after a gravitational close pass by Jupiter, that it will, in fact, collide with the Earth. NASA’s Planetary Protection Office has called it, “the single most dangerous object known to humanity.”
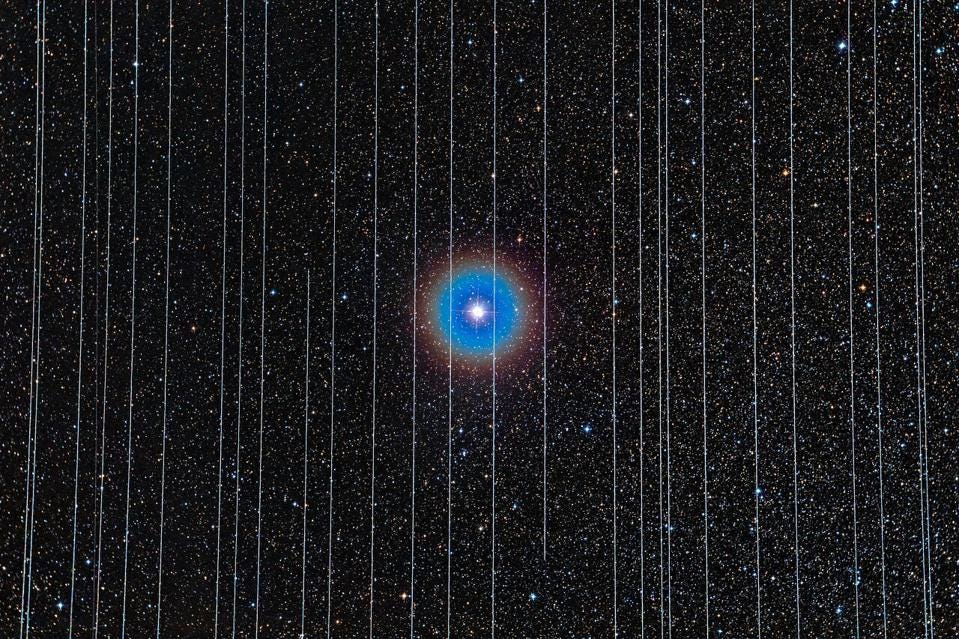
Of course, the largest problem we’re facing today, from a scientific perspective, is with the endeavor to identify and track these objects themselves. Of all the astronomical endeavors that are being impacted by the current set of bright, novel satellites being launched to provide high-speed, low-latency internet across the world, identifying, tracking, and determining the orbits of potentially hazardous objects is the one field of research that is suffering and will suffer the most losses. We are literally playing a game of chance with our very survival by allowing the presence of these satellites, further underscoring the need for swift, global regulation of the environment surrounding the Earth.
Ideally, we should be monitoring the skies with large, wide-field telescopes, looking for tiny changes in the brightness and position of Solar System objects to determine the threat level. We should be testing technologies, with international cooperation and significant planet-wide investments, to mitigate the various likely scenarios. And we may even want to consider space-based observatories at L3: the point in the Earth’s orbit located on the far side of the Sun, where the most difficult-to-identify potentially hazardous objects are located. If we took those interventions, we’d have approximately a decade to prepare for any likely potential impact. Without them, which is where we are today, we simply have to rely on not getting unlucky to ensure our survival.
Ethan Siegel thanks Dr. Almog Yalinewich of the University of Toronto for an extremely helpful discussion concerning mitigations and interventions in the event of a Don’t Look Up scenario as respects the Earth.