Ask Ethan: Why do we have an Oort Cloud?
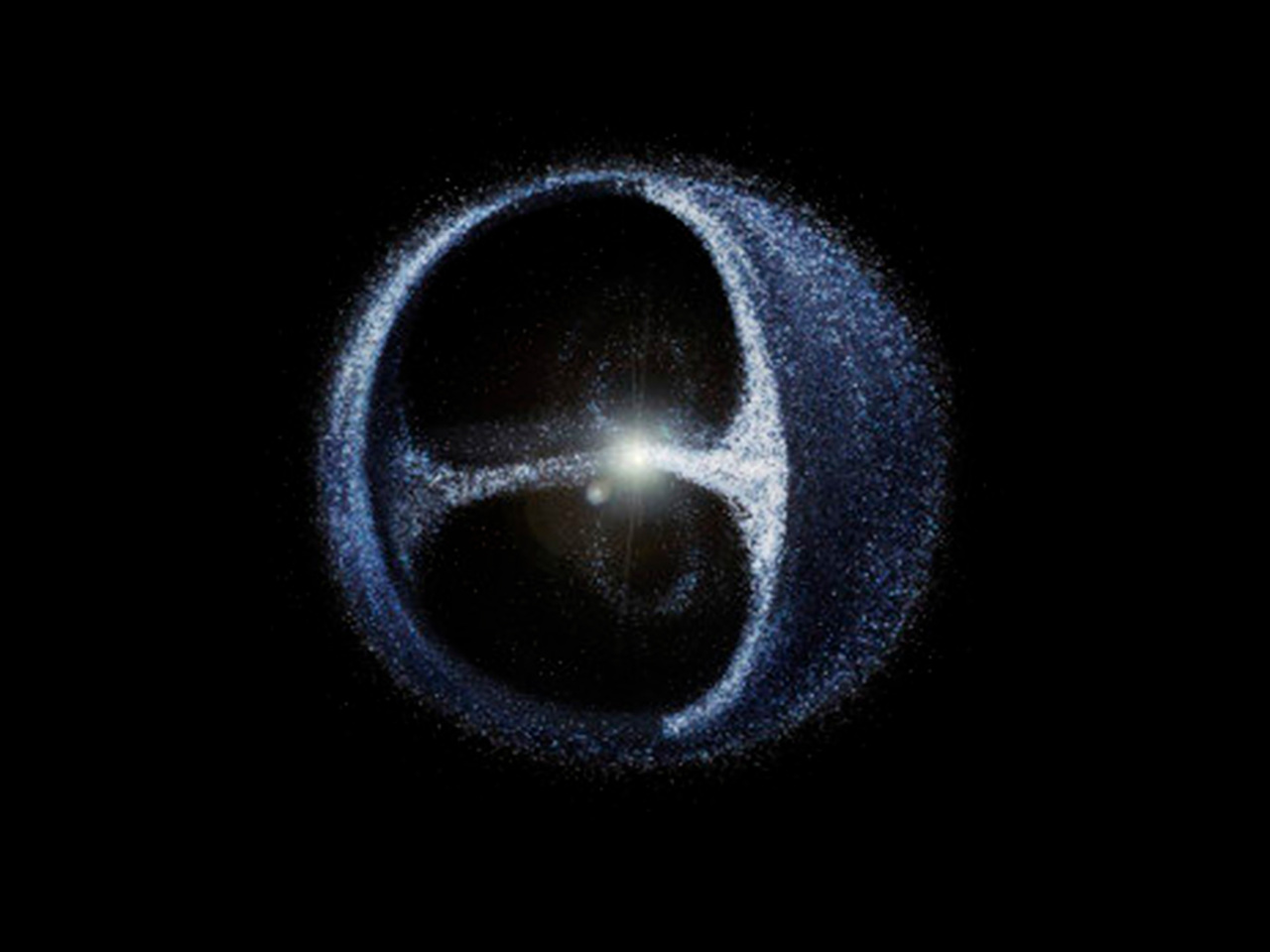
- Out beyond the Kuiper belt and the outermost object ever observed lies the Oort Cloud: a collection of rocky and icy bodies extending light-years into space.
- Although we've never seen even a single object at that distance from the Sun, we're almost certain this cloud exists, and have been since the 1950s.
- From ultra-long-period comets to the science of how planetary systems form, here's what the Oort Cloud is, and why it's practically inevitable.
What, precisely, is in our Solar System? And how far out do we have to look before our Solar System truly comes to an end? For the first question, you might think that looking around close to the Sun is a great way to answer that question, but it’s literally just the tip of the iceberg. For the second question, you can turn to the pull from the Sun itself and ask where the Sun’s gravitational force becomes negligible compared to the influence from the other stars in the Milky Way. Between those two extremes — the objects we can see and the edge of the Sun’s gravity — lies the Oort Cloud.
At least, that’s what we assume. First theorized in 1950 by Jan Oort, we fully suspect that there’s an enormous cloud of objects surrounding the Sun, from well beyond the Kuiper belt all the way to multiple light-years away. But what is it and where did it come from? That’s what Patreon supporter Dwayne Williams wants to know, asking:
“[P]lease write an article on the Oort Cloud. What is it? Why is it in that region of space? And what’s it made of?”
It’s one of the most curious and bold predictions astronomy has ever made. But Oort didn’t come up with this idea in a vacuum. When we look at what we know, it’s virtually impossible to explain what’s out there without an Oort Cloud.
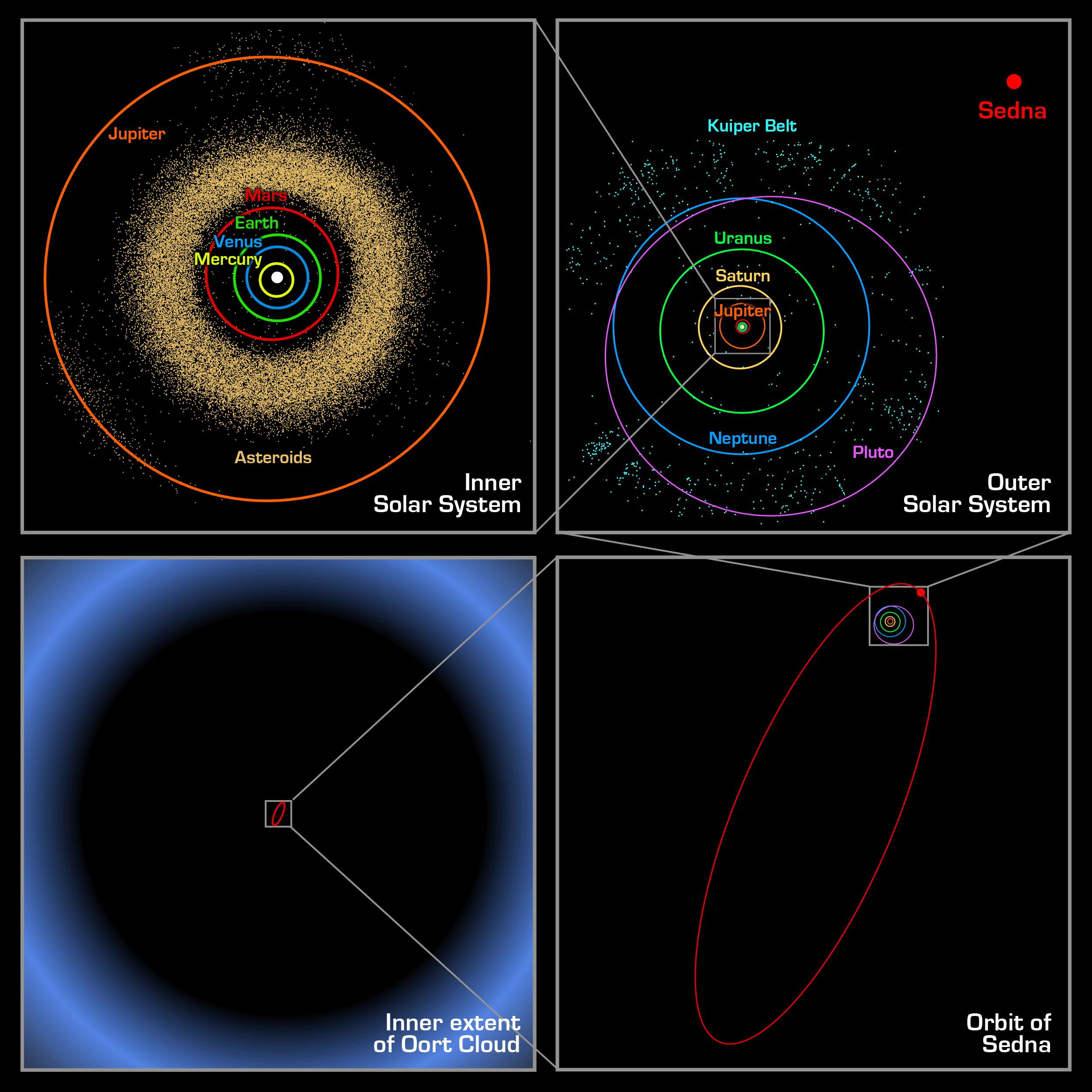
It might not seem like it, but there’s a reason why the first thing we should ask ourselves is the question of what, precisely, is in our Solar System? For example, when we see our Sun, our Moon, or a planet, we know — even if we don’t know precisely what the answer is — that there exists a physical explanation for that object’s existence. There’s a reason why it’s there with specific properties, even if that reason is pure random chance within a star-forming region. The combined influence of gravity, radiation pressure, angular momentum conservation, and the initial conditions that occurred within the molecular cloud that gave rise to us is what led to the planets forming.
Similarly, when we see objects like Saturn’s moon Phoebe or Neptune’s moon Triton, we can immediately recognize that they didn’t form along with their parent planets from their orbital properties; they must have been gravitationally captured and originated elsewhere. We now know that Phoebe likely originated from much farther out, perhaps as a Centaur or a Kuiper belt object, and was gravitationally captured. Triton, similarly, must have originated from the Kuiper belt, which is no surprise given its similarities to Pluto and Eris.
If an object exists here, it must have an origin story to account for its existence.
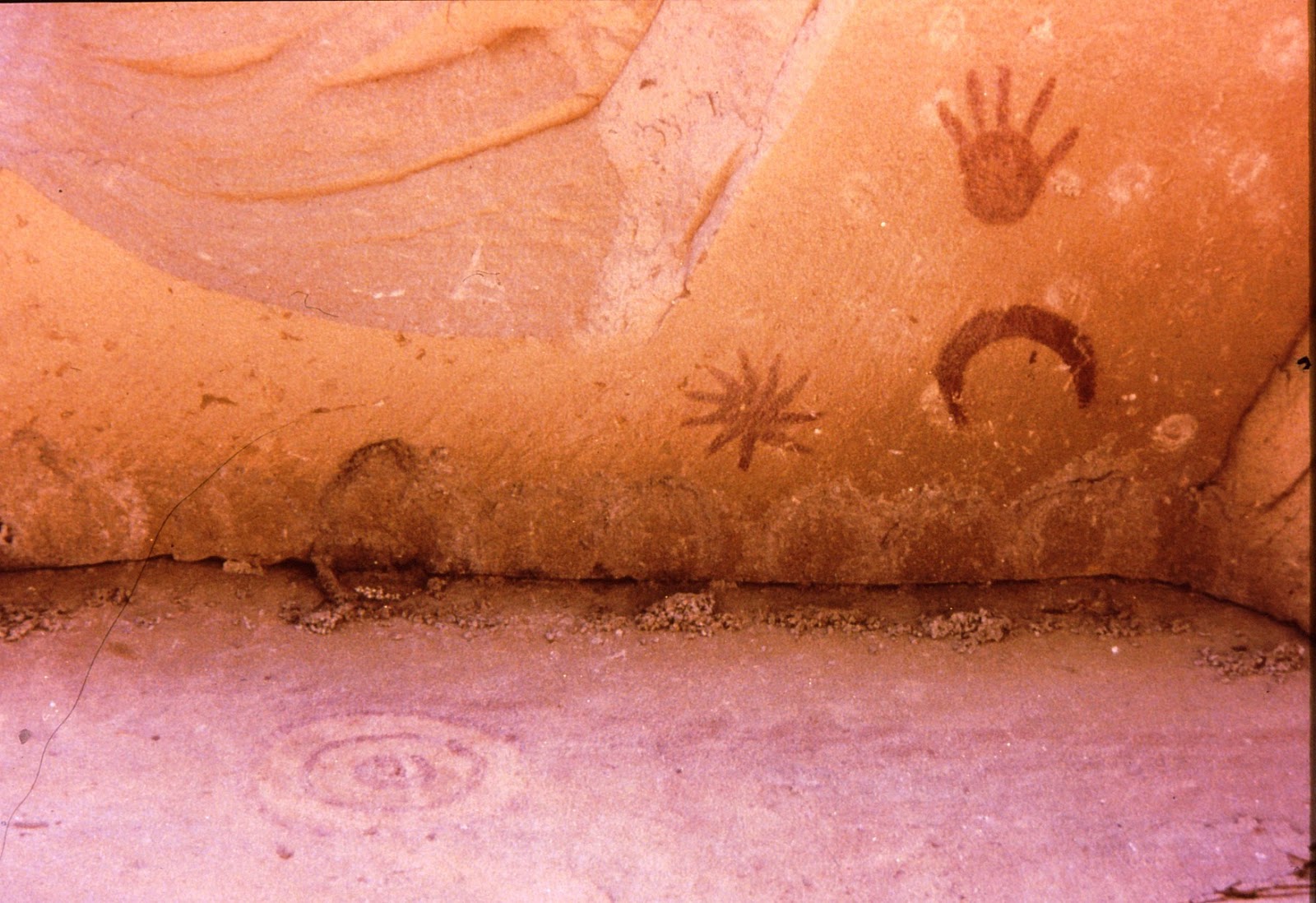
This also holds true for comets that pass through our Solar System. Observed by humans since at least pre-historic times, it wasn’t until the work of Edmund Halley that we began to realize that many of the comets that appeared in our night sky were periodic. Today, we know of over 100 independent periodic comets: comets that plunge through the inner reaches of the Solar System, developing tails and comas, reaching their closest approach to the Sun, and then heading back out once again, far beyond the limits of not only human vision, but beyond what even the most powerful telescopes ever built can image.
And yet, despite their orbits carrying them far out of our range, we can be confident of their eventual return. After all, the law of gravity has been known — at least at the Newtonian level, itself remarkably accurate for describing objects in distant orbits around the Sun — for over 300 years. Many of the periodic comets return on timescales of around a century or so, including:
- Comet Halley
- Comet Pons-Brooks
- Comet Olbers
- Comet Westphal
- Comet Crommelin
- Comet Tempel-Tuttle
- Comet Swift-Tuttle
- Comet Barnard
There are more than 100 others — enough to make you wonder where they all came from.

Interestingly enough, these listed comets all have a number of properties in common with one another. They’re on very eccentric orbits, with eccentricities of 0.9 or higher (where an eccentricity of 1.00 is the border between being gravitationally bound to our Sun, or not); they all have aphelions that carry them out beyond Saturn (with almost all of them going beyond Neptune, as well); and, at their farthest from the Sun, they all travel extremely slowly. Comet Halley, for example, last reached aphelion in 1948, where it was traveling at a mere 0.91 km/s. Swift-Tuttle is similar, with a minimum speed of 0.8 km/s.
So, with that said, where did these comets originate from?
The tremendous number of similarities between this class of comets strongly suggests that somewhere, out beyond the orbit of Neptune, there exists a large population of sub-planetary bodies moving very slowly with respect to the Sun, but still in a stable orbit. Every once in a while, something occurs — perhaps a gravitational tug of some sort — to perturb their orbits, hurling them into the inner Solar System. When that occurs, and they get close enough to the Sun, heat up, and begin expelling their volatiles. If enough time passes, either they’ll get perturbed again — potentially hurling them into one body or another or out of the Solar System entirely — or they’ll simply evaporate, boil, or sublimate away.

We’ve since discovered the source of most of those comets: the Kuiper belt. Starting in the 1990s and continuing through the present day, we now know that our outer Solar System contains a tremendous number of objects in a belt that extends well beyond Neptune. Perhaps ironically, the person it’s named for — Gerard Kuiper — thought that it might no longer contain any objects, which he thought might have been swept clear by gravitational interactions.
It turns out there are other populations of comets as well. Some arise from the Centaurs, which are comet- and asteroid-sized objects primarily located between Jupiter and Neptune. Some arise from asteroids themselves; the parent body of the Geminid meteor shower, gracing our skies every December, is the asteroid 3200 Phaethon.
And some of them plunge into the Solar System only to disappear, never to appear again for all of human history. These comets were initially thought to be on parabolic or hyperbolic orbits — where they would pass through once, never being gravitationally bound to our Sun — eventually returning to interstellar space. But as our telescopes and observations continued to improve, we started discovering a remarkable set of facts about these comets. If you calculated their speeds as they were leaving the Solar System, their speed matched almost exactly the escape velocity needed to escape from the Sun. It was as though they fell into our Solar System from being practically at rest.
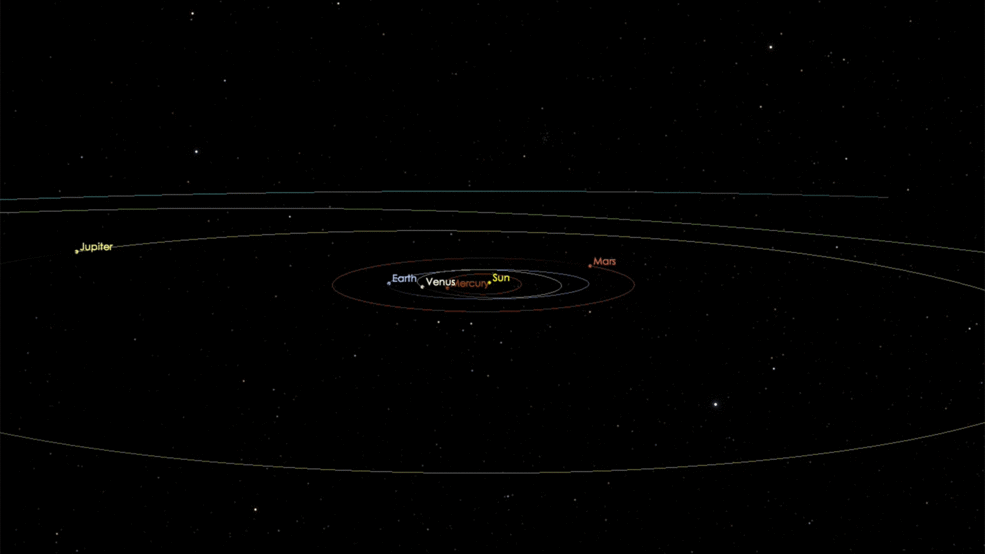
This makes no sense and led to a paradox. When we measure the stars of the Milky Way, all move with respect to the Sun: typically with speeds between 10 and 40 km/s. Why would there be a large population of interstellar objects, indistinguishable from our Kuiper belt objects in nature, where none of them were moving at all with respect to our Solar System?
Before Oort came along, a couple of people proposed there might be a population of objects originating from extremely far away, but still bound to our Sun. One was Armin Leuschner, who suggested these comets might actually be on very eccentric elliptical orbits. Another was Ernst Öpik, who theorized the existence of a “cloud” around our Solar System that acted as a reservoir for these objects.
But if a cloud existed, it would have to be right at the edge of what was gravitationally bound to our Solar System. In astronomy, a rule of thumb that we use to calculate gravitational stability is known as the Hill sphere, which is the region of space around an object where satellites can remain gravitationally bound to it. The Earth’s Hill sphere goes out to about 1.5 million kilometers: roughly where the James Webb Space Telescope will be launched to — beyond that, the Sun’s gravity dominates. The Sun’s Hill sphere goes out a few light-years, and beyond that, the stars in interstellar space start to become just as important.
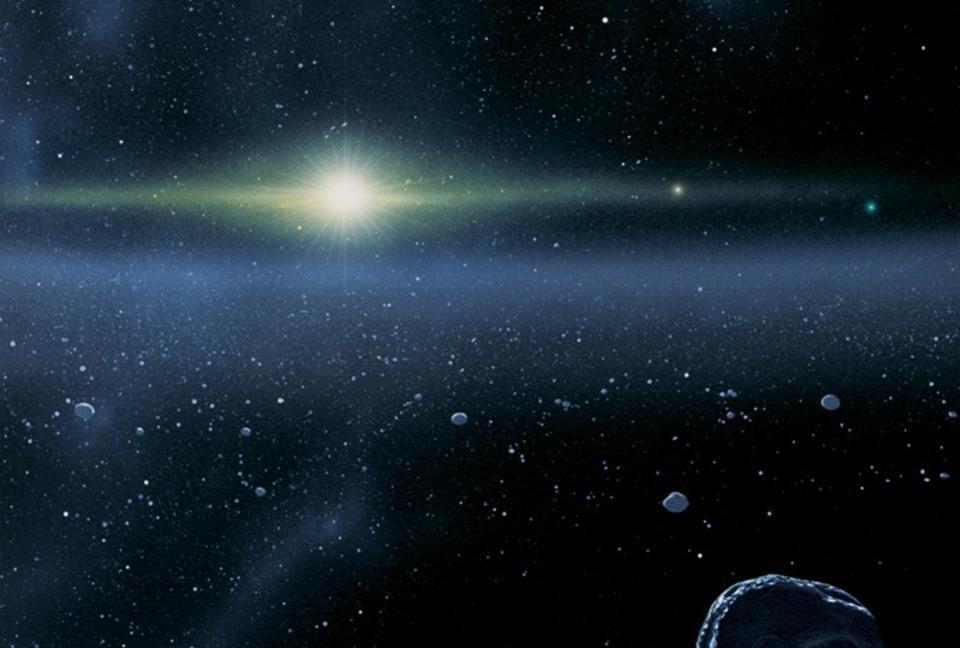
The attribution of the cloud’s existence is generally granted to Oort, however, on account of Oort putting forth the following paradox that, he reasoned, necessitated its existence.
- Given that the Solar System has existed for a long time, and that cometary bodies are small, their existence is unstable.
- Dynamically, they will either collide with the Sun, a planet, or a moon, or be ejected entirely owing to planetary perturbations; they cannot survive for even millions, much less billions, of years.
- Compositionally, comets are largely made of volatile ices, which means as they repeatedly approach the Sun, they’ll either run out of volatiles and the comet will be destroyed, or the comet can develop an insulating crust to prevent further outgassing.
Therefore, Oort reasoned, every comet that we see must be relatively “new,” in the sense that it only just started, recently in cosmic time, passing close by the Sun. Given that there are so many of them, and that they appear to have originated from a position nearly at rest with respect to the Sun, they therefore must be somehow held in some sort of reservoir: a collection of objects that’s gravitationally bound to the Sun.
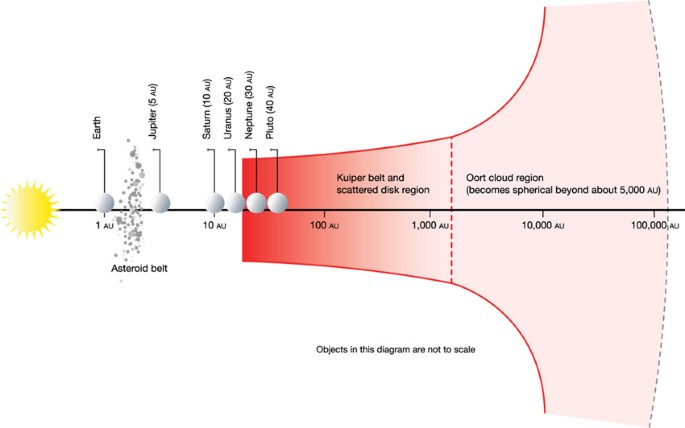
When we examine these comets today, the ones that we’ve measured accurately appear to have aphelia that take them approximately ~20,000 astronomical units from the Sun, or about one-third of a light-year. Not all of them, mind you, but too many of them for it to be mere coincidence. There are rarer long-period comets with aphelia that are more like ~10,000 astronomical units, which is precisely what you might expect for a long-period comet that’s had its orbit affected by the gravitational influence of the planets: drawn inward by a slight amount.
The big open questions, then, are twofold:
- How big is the Oort cloud? How are the objects distributed within it, and how far, both inward and outward, is its extent?
- How did it develop, and when? Does every stellar system have one, or is our Sun “lucky” to have one, in some regard?
Although we have answers that we think are pretty good to these questions, the fact remains that the best ideas we have about them remain unconfirmed. However, as our telescopes improve, both in size and in wavelength coverage, and as we continue to learn more about newly forming stellar systems and about the objects in interstellar space, we inch closer and closer to the answers.

One of the remarkable facts about the long-period comets (hypothetically from the Oort Cloud), the Kuiper belt comets, and the comets that originate from closer to Jupiter is this: they all appear to be made of the same types and ratios and isotopes of materials. They all appear to have formed at approximately the same time: 4.6 billion years ago. And, therefore, they formed from the same nebula in space that the rest of our Solar System formed from.
But then it gets murky.
- Were the Oort cloud’s objects formed in situ, or were they hurled there from closer in by gravitational interactions with planets?
- Did they all form from the part of the pre-solar nebula that formed our Sun and Solar System, or was there a dynamical exchange of material with other young stellar systems?
- Did the Solar System always have an Oort Cloud, or did the cloud’s mass grow as the Solar System evolved for quite a time, before interactions with passing stars began to deplete it?
- Did the objects of the Oort Cloud form from collisional debris of other objects in the outer Solar System?
- Did the scattered disk of objects, from which many Halley-type comets arise, contribute substantially to the Oort Cloud’s population?
- And where is the transition from the inner Oort Cloud, which is more disk-like, to the outer Oort Cloud, which is more spheroidal?
Although estimates typically place the inner Oort Cloud from 0.03-to-0.32 light-years away and the outer Oort Cloud from 0.32-to-0.79 light-years away, these figures are disputed, with some contending that the inner Oort Cloud doesn’t begin until ~0.08 light-years from the Sun, and some contending that the outer Oort Cloud extends for over ~3 light-years from the Sun!
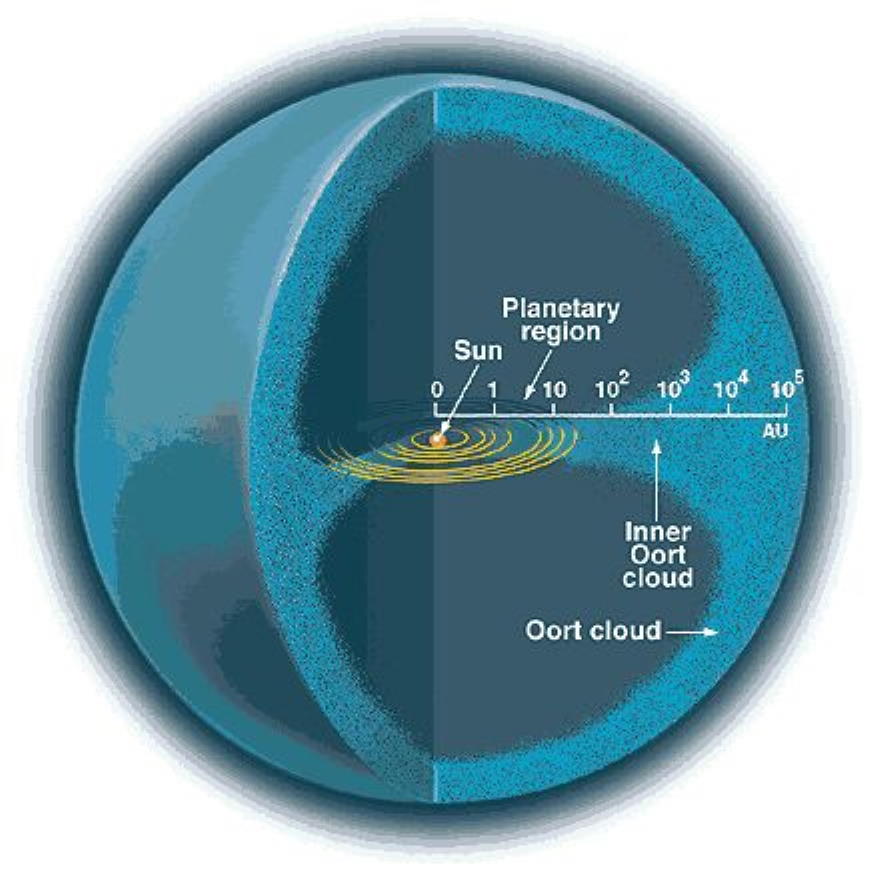
One fascinating thing to consider that isn’t disputed, however, is this: Over time, and specifically over at least the most recent 3.8 billion years, the Oort Cloud has consistently been depleted. The Sun typically experiences a close encounter with another major interstellar object, like another star, a little more often than once every ~1 million years, indicating that there have been thousands of such events in our history. Each such encounter would impart large gravitational “kicks” to any loosely bound object, potentially triggering cometary storms but certainly depleting the Oort Cloud. In environments such as globular clusters or near the galactic center, the persistence of Oort Clouds for billions of years may be nigh impossible.
Although its origin and full extent are still being studied, we can confidently state that it’s made of the same protoplanetary material that the other primordial bodies in our Solar System are made of. The Oort Cloud objects are similar in composition to the other comets, centaurs, and Kuiper belt objects that we see: a mix of ices and rocks. The rocky material is likely very similar to planetary mantles, including Earth’s, while the ices are likely to be a mix of nitrogen, water-ice, carbon/oxygen ices, and possibly even hydrogen ice. For any stellar system that forms, an Oort Cloud likely forms along with it. Only with more science, including better simulations and observations, will we ever know for certain.
Send in your Ask Ethan questions to startswithabang at gmail dot com!