Dark matter may be completely invisible

The LUX experiment just set the tightest bounds ever on dark matter, and may lead us down a different path entirely.
“For me the best answer is not in words but in measurements.” –Elena Aprile
Dark matter is the most elusive substance ever detected in the Universe, and even at that, it’s only been detected indirectly. We know it interacts gravitationally, but it’s so sparse and diffuse that Earth-based experiments don’t stand a chance at seeing that interaction. Instead, if we want to see this new form of matter directly, we have to hope that there’s an additional interaction: a way for dark matter to scatter off of normal matter, and produce a recoil due to a collision. In an announcement earlier today, the LUX Collaboration — running the Large Underground Xenon experiment — performed the longest, deepest, most sensitive search for dark matter ever, using 370 kilograms of liquid xenon with the detector running for a total of 20 months. The final result? Not a single dark matter collision was observed.
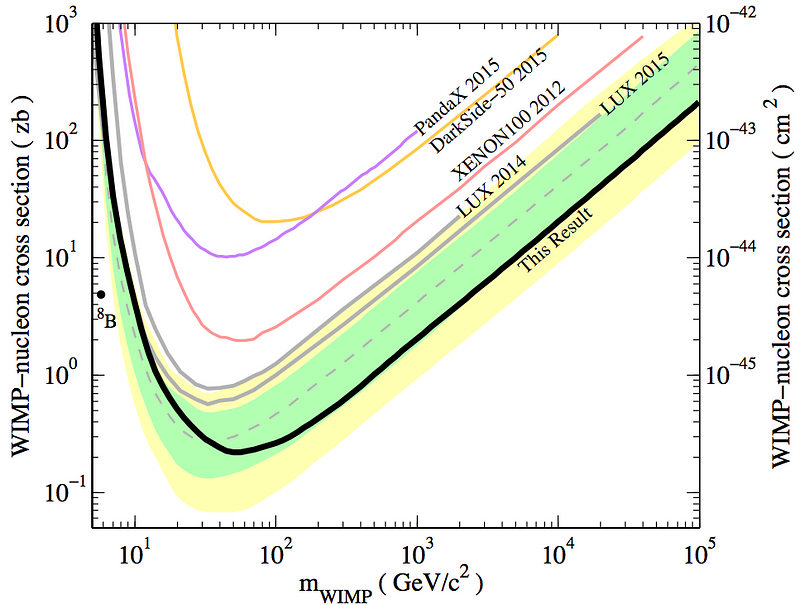
A huge variety of astrophysical observations point to the existence of dark matter, and point to its presence in a massive halo surrounding every large galaxy ever observed. Dark matter is required to reproduce our observations of everything from galaxy rotation curves to the gravitational bending of light around clusters; from the large-scale filamentary structure of the Universe to the tiny fluctuations in the cosmic microwave background; from the correlations of galaxies 500 million light years apart to the existence of the tiniest mini-galaxies of all. Most spectacularly, we observe dark matter separating from normal matter when two massive galaxy clusters collide. Without dark matter, the explanations for these phenomena all fall apart; we know it must be real.
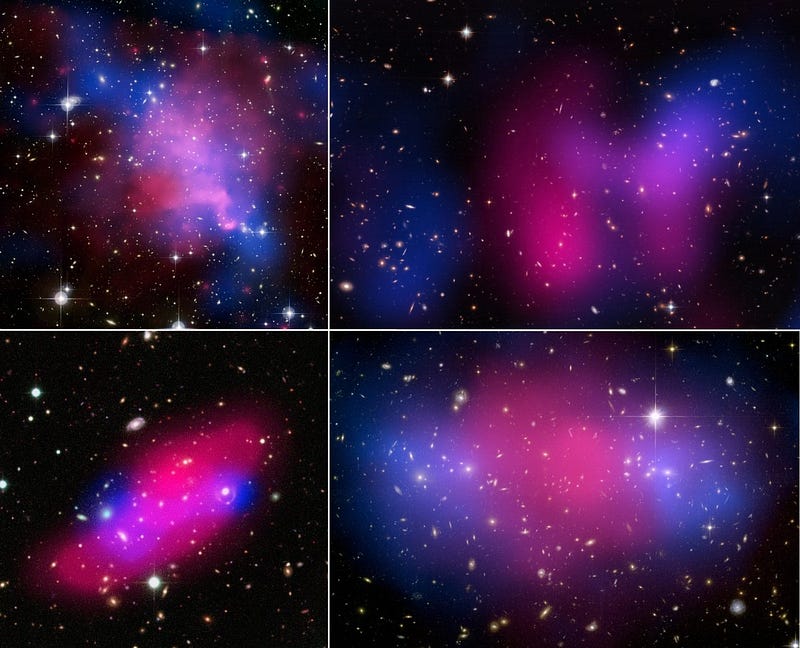
But if it’s real, we really want to be able to detect it directly, under laboratory conditions. To do that, we need to know something about the particle nature of dark matter itself, because we need for it to interact with normal matter: with the particles in the Standard Model, the ones we know how to detect here on Earth.
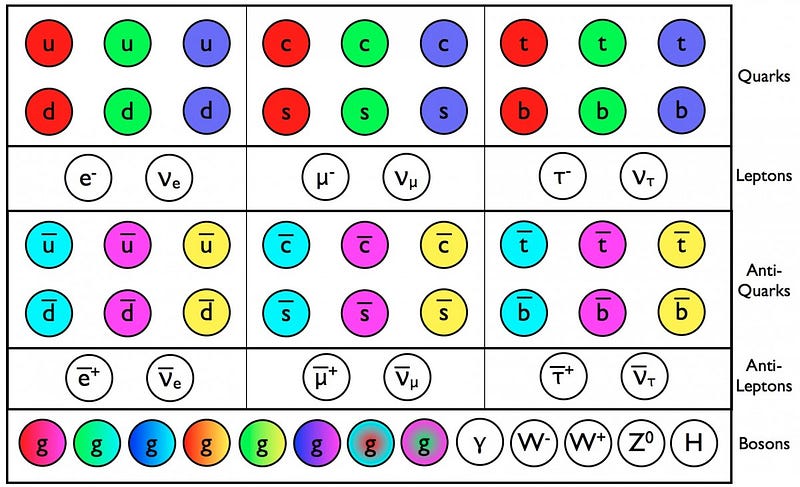
So what are the possibilities for that interaction? It could occur through any number of pathways, with a wide variety of masses allowed for the dark matter. The most common models, though, all have a few features in common:
- They all have dark matter not interacting through the strong nuclear or the electromagnetic interaction.
- They all have dark matter in a mass range that’s heavier than the mass of an electron, and lower than the maximum energy of the LHC.
- And they all have dark matter interacting through either the weak nuclear interaction or a new force that’s weaker than that, but stronger than the gravitational interaction.
If you’re willing to make those assumptions, a general experimental design emerges: take a tremendously large collection of atoms and look for the disturbance a passing, colliding dark matter particle would cause.
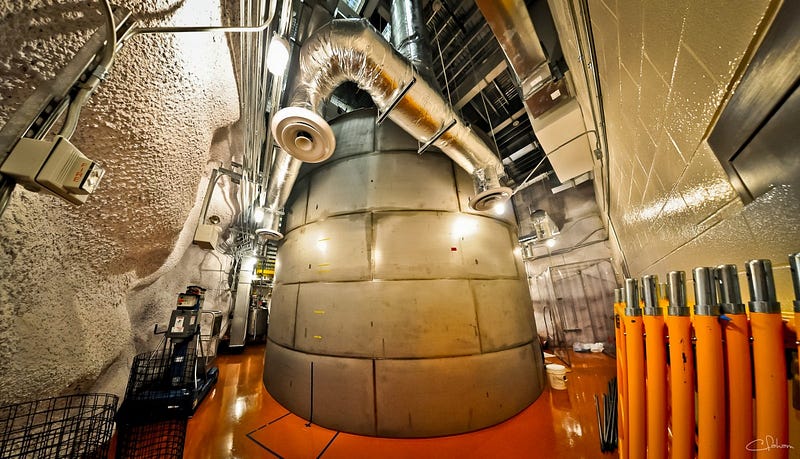
Outdoing previous experiments like CDMS and its successors, Edelweiss, PandaX and Xenon, the LUX collaboration has collected more data at a greater sensitivity than any experiment before it. With a sensitivity range that sets the record from just about a fifth of a proton’s mass (~0.2 GeV/c2) to about ten times the mass of the heaviest known particle, the top quark (more than 1,000 GeV/c2), LUX has pushed the interaction limits not only lower than ever before, but significantly lower than the experiment was even designed to push them.
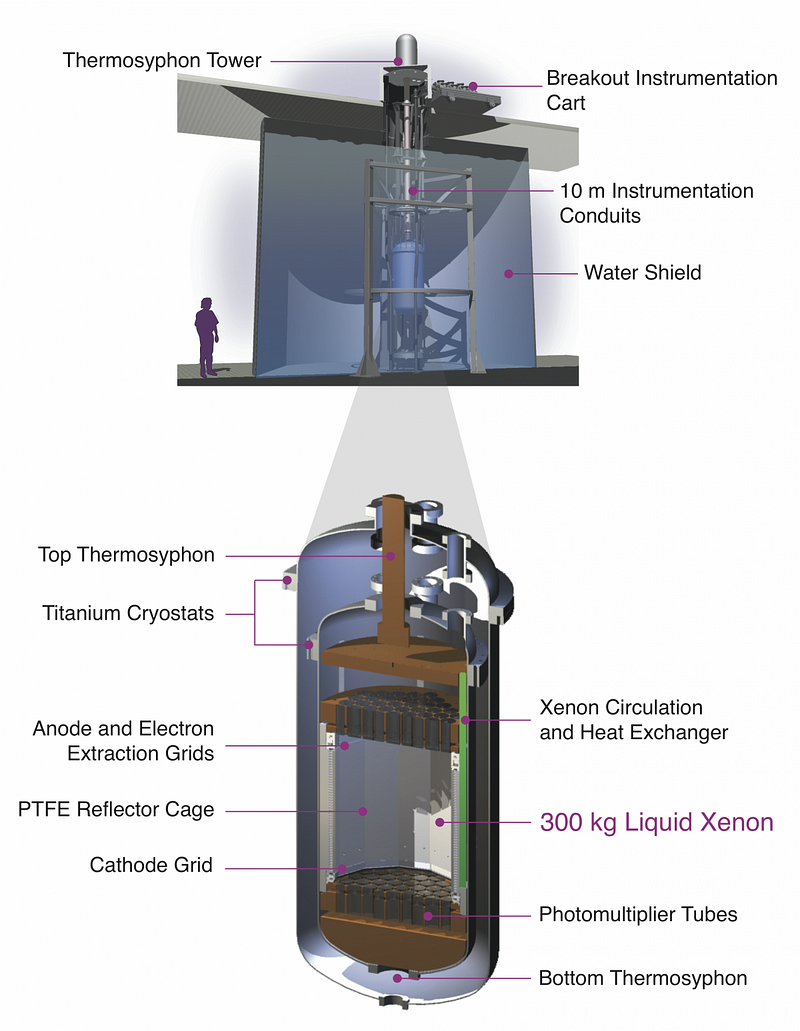
According to Rick Gaitskell, co-spokesperson of LUX:
With this final result from the 2014–2016 search, the scientists of the LUX Collaboration have pushed the sensitivity of the instrument to a final performance level that is 4 times better than the original project goals. It would have been marvelous if the improved sensitivity had also delivered a clear dark matter signal. However, what we have observed is consistent with background alone.
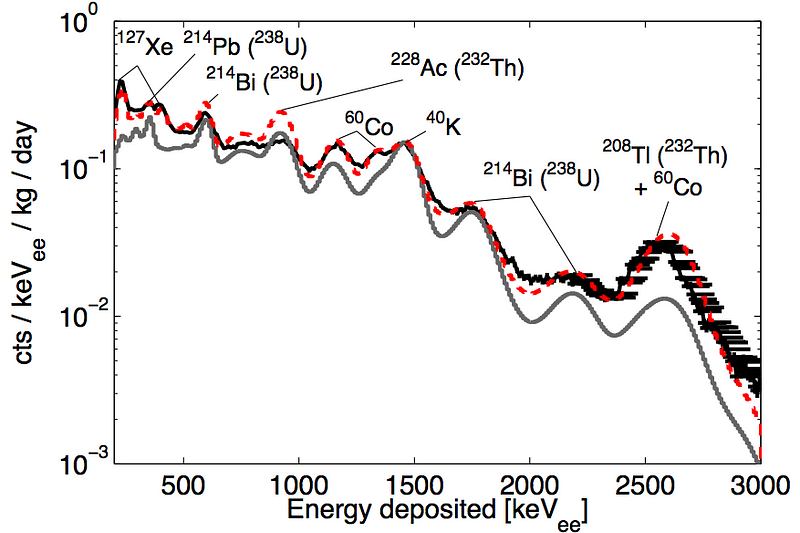
The LUX results rule out all the touted detections from experiments like DAMA, LIBRA and CoGeNT; it rules out most models of dark matter from supersymmetry and extra-dimensions. It means that many ongoing dark matter experiments are destined to find absolutely nothing at all. By filling an ultra-sensitive detector with more than a third of a tonne of liquid xenon, a single collision between a dark matter particle and a xenon nucleus would produce a recoil visible by the photodetectors surrounding it.
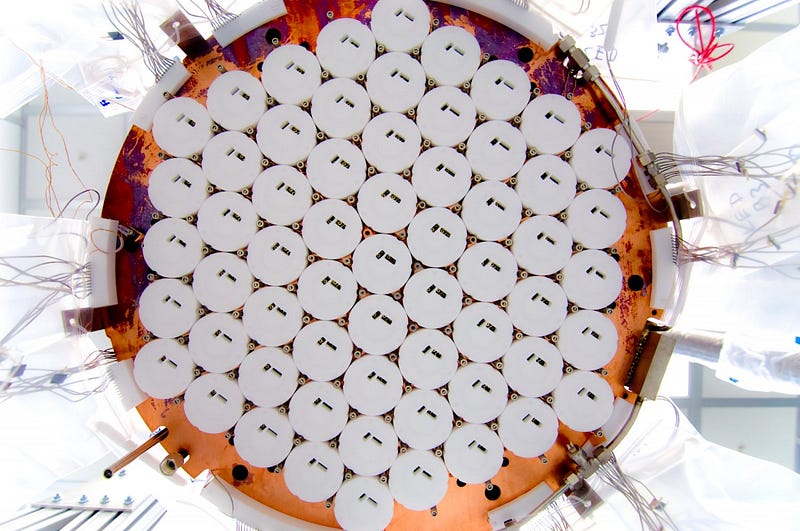
By burying the detector more than a mile underground, shielded by rock, and surrounding it inside a 72,000-gallon, high-purity water tank, it’s protected from cosmic rays, solar events, terrestrial radiation and other sources of contamination. When all the anticipated backgrounds are accounted for — including natural radioactivity, muons and cosmic neutrinos — the LUX collaboration concludes that a total of zero significant events were observed over the 20 month time period the experiment ran, from 2014–2016. According to co-spokesperson Dan McKinsey:
As the charge and light signal response of the LUX experiment varied slightly over the dark matter search period, our calibrations allowed us to consistently reject radioactive backgrounds, maintain a well-defined dark matter signature for which to search and compensate for a small static charge buildup on the Teflon inner detector walls.
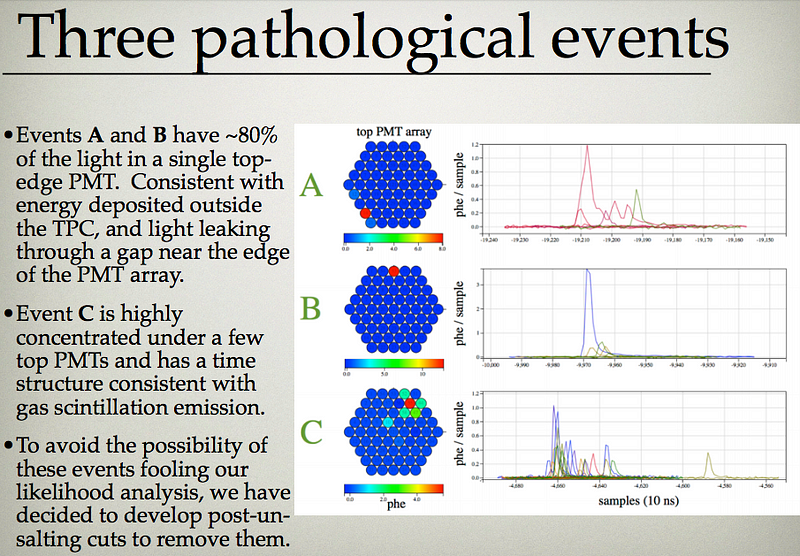
By running a whole slew of new background rejection and calibration techniques, LUX became sensitive to events that would have a fantastically tiny rate. As LUX project scientist Aaron Manalaysay detailed:
These careful background-reduction techniques and precision calibrations and modeling, have enabled us to probe dark matter candidates that would produce signals of only a few events per century in a kilogram of xenon.
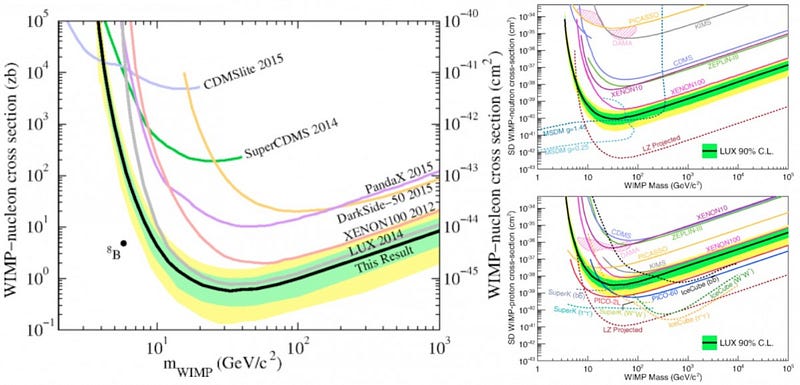
The null detection is incredible, with a fantastic slew of implications:
- Dark matter is most likely not made up, 100%, of the most commonly thought-of WIMP candidates.
- It is highly unlikely that whatever dark matter is, in light of the LUX results, will be produced at the LHC.
- And it is quite likely that dark matter lies outside of the standard mass range, either much lower (as with axions or sterile neutrinos) or much higher (as with WIMPzillas).
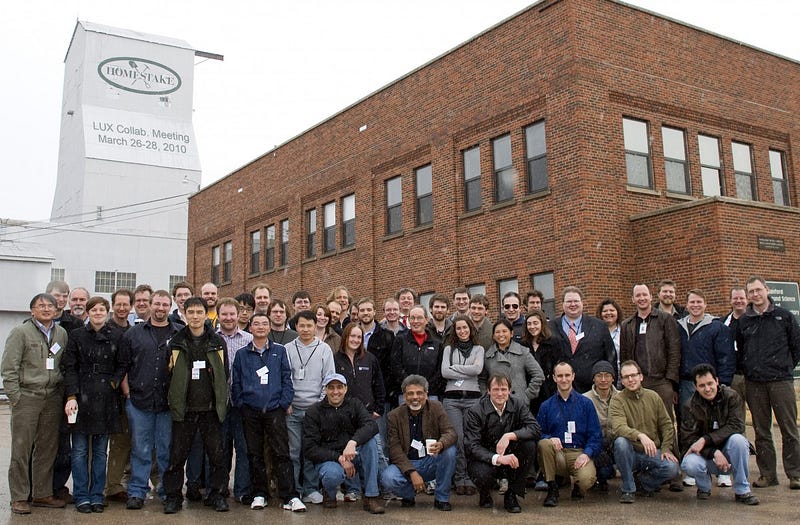
This works for both spin-dependent and spin-independent models of dark matter, which means that it doesn’t matter what type of quantum particle — a fermion or a boson — dark matter happens to be. Whatever it is, we not only haven’t found it, we haven’t found it to such an incredible degree of precision that it’s time to take our “most likely models” of what it is and to start thinking differently. For in this Universe, the stars might have “let there be light,” but LUX has shown us that dark matter isn’t quite what we thought we were looking for.
This post first appeared at Forbes, and is brought to you ad-free by our Patreon supporters. Comment on our forum, & buy our first book: Beyond The Galaxy!