Arguments against dark matter in the Bullet Cluster fall apart
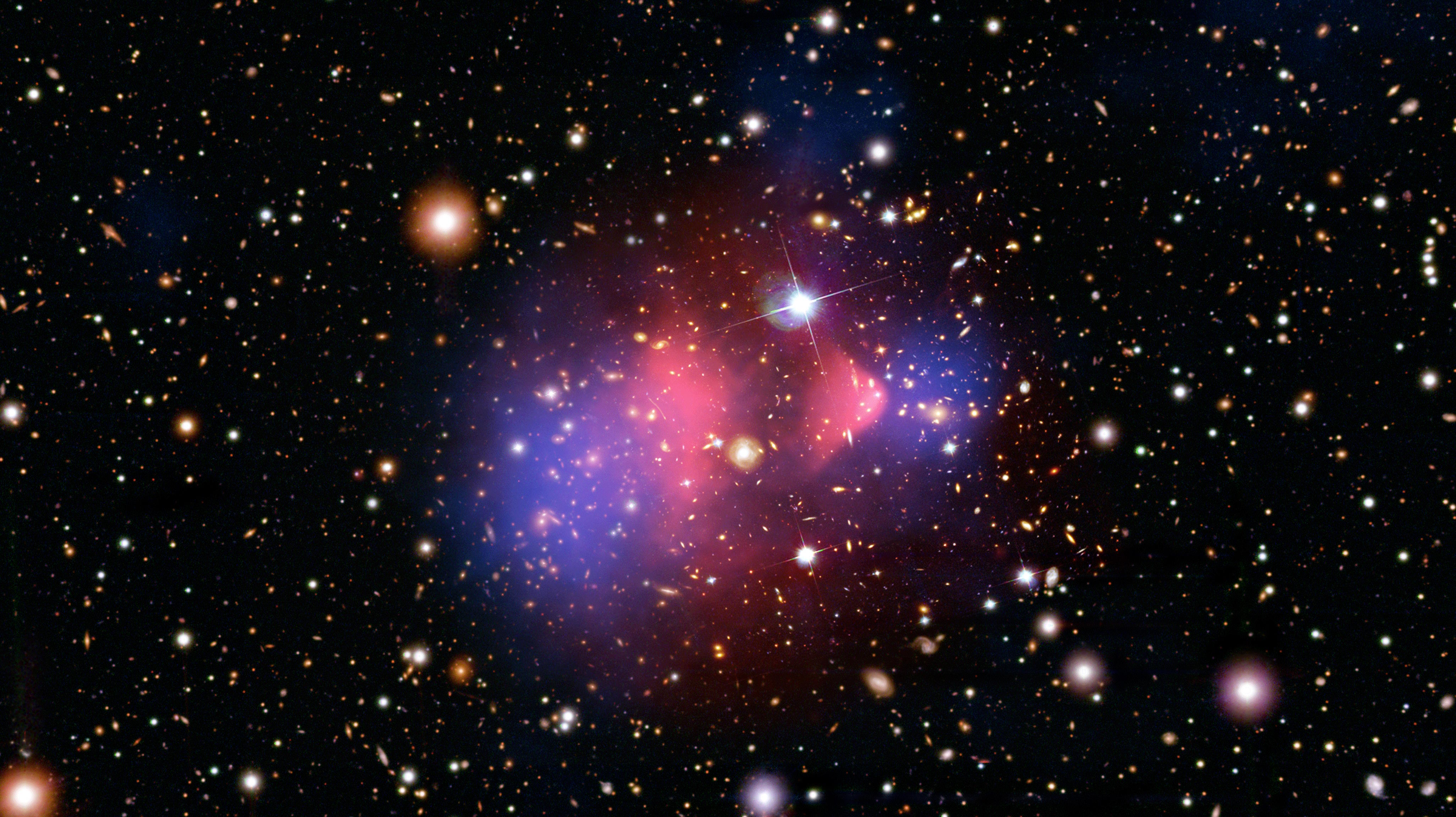
- In the mid-2000s, the Bullet Cluster became the first colliding galaxy cluster to have its X-ray emissions and gravitational lensing signals measured, indicating a clear mismatch between the two.
- This has long been hailed as an “empirical proof” of dark matter’s existence, as the separation between the “mass” signal and the “normal matter” signal is interpreted as revealing dark matter’s existence.
- However, many who favor MOND over dark matter have alternative explanations for the Bullet Cluster, arguing that it conflicts with cold dark matter’s predictions. But how do those explanations hold up?
It’s one of the most well-accepted ideas in the Universe, and yet one of the most controversial: the notion of dark matter. Here in the Solar System, the Sun and planets are enough to explain all of the gravitational effects that we see: simply use the law of general relativity and what you predict matches what you observe exactly. But on larger scales — the scales of galaxies, groups and clusters of galaxies, and even the grand cosmic web — the normal matter that we know of, including everything made of protons, neutrons, and electrons, simply isn’t sufficient to explain what we observe. Some other massive ingredient, i.e., dark matter, is needed to bring things back into alignment.
On the scale of a single galaxy and below, other explanations, such as modifications to gravity, are just as successful as dark matter, with MOND being a prime example. In fact, tests of wide binary stars may soon be able to test these ideas against the notion of dark matter directly. But on larger cosmic scales, such as the scales on which clusters of galaxies collide, those same modifications of gravity are no longer successful, but dark matter remains perfectly valid.
Or does it? Many in the modified gravity community point out two major objections to the standard story of colliding galaxy clusters, such as the Bullet Cluster, as evidence for dark matter. But do those objections actually carry water when we look at them in detail? Let’s investigate for ourselves.
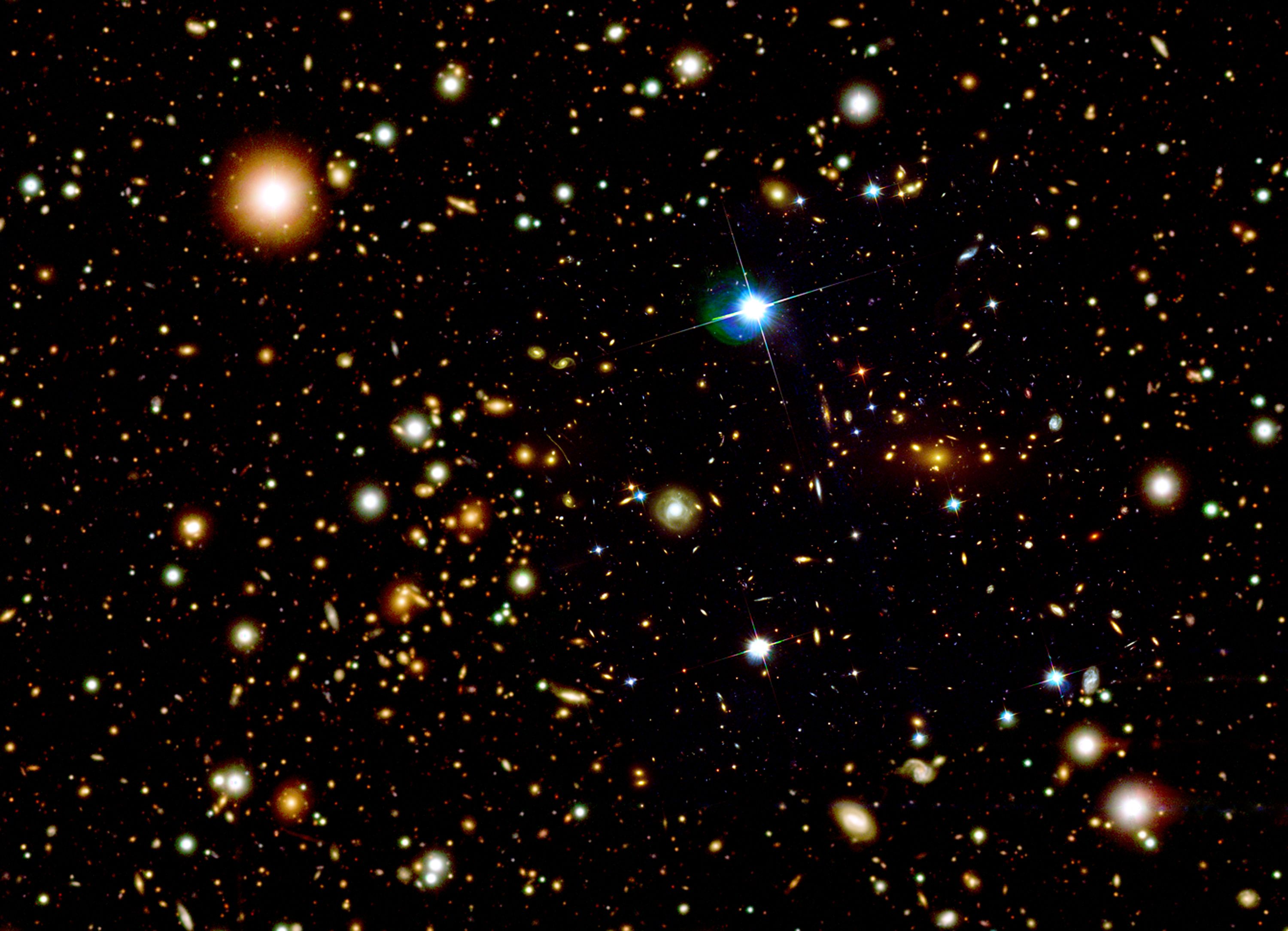
The standard picture of the Bullet Cluster
Above, you can see what’s known as the Bullet Cluster. In optical (visible) light, it simply looks like two collections of galaxies: like two large groups (or clusters) of galaxies that are located relatively near to one another in the night sky. However, these galaxy clusters aren’t just located in the same region of the sky, they’re actually very close to one another in three-dimensional space. Moreover, they aren’t just in close proximity to one another, but rather are in the process of interacting with each other. There are two signals we can look at that tell us precisely how these interactions have occurred.
The first signal comes from looking at X-ray wavelengths of light. Whereas optical light, or visible light as we know it, is excellent at revealing traditionally luminous sources of matter such as stars, X-ray light is instead outstanding at revealing gas that’s been heated up to extremely high temperatures. Gas not only exists copiously inside nearly every galaxy, but in the intergalactic medium: the space between galaxies, particularly in bound structures like galaxy groups and galaxy clusters.
When we look at the Bullet Cluster in X-ray wavelengths, we find a large amount of hot, X-ray emitting gas and plasma in the space between the two galaxy clusters. Not only is this evidence that the two clusters have collided recently, but the “shock” feature, appearing like a tautly drawn bow, tells us that these clusters have collided at great speeds: around 5000 km/s.
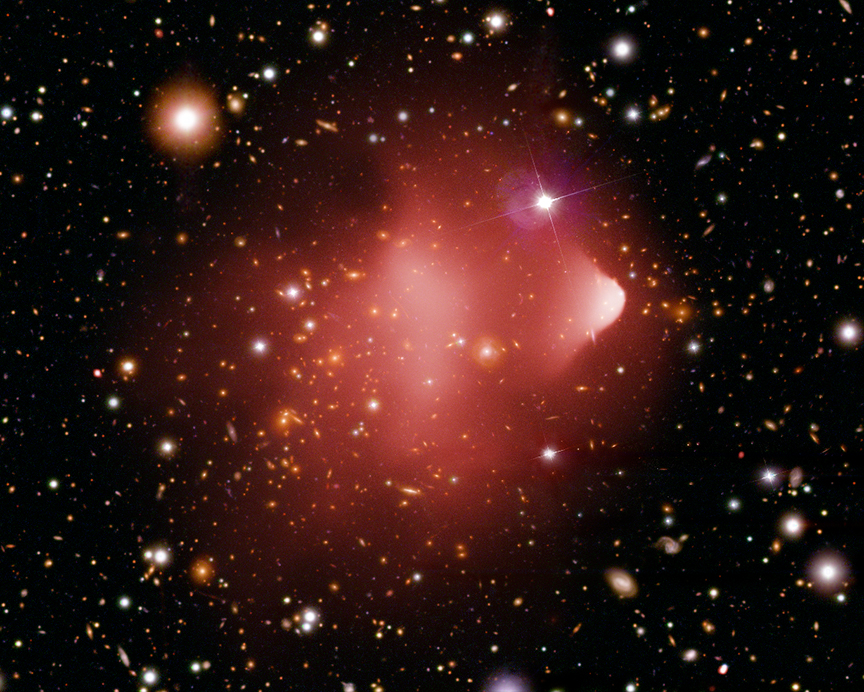
However, there’s a second signal that we can look at as well: the gravitational lensing signal created by all the mass in this system, including the mass within these galaxy clusters as well as the mass in the space between them. Unlike light-dependent signals that rely on properties of matter like its temperature and brightness, gravitational lensing is solely determined by mass: the cumulative amount of mass present along any particular line-of-sight. The presence of this mass bends and distorts the appearance of the space behind it, as well as any light that happens to be emitted from background sources of light along that same line-of-sight.
There are two types of gravitational lensing:
- strong lensing, where background sources are stretched into arcs, rings, and even multiple images, like a reflection in an extremely distorted mirror like the kind you might find in a Fun House,
- and weak lensing, where the shapes and orientations of background sources are preferentially distorted into a series of ellipsoidal shapes, a much more subtle but much more ubiquitous type of gravitational lensing.
It’s largely through weak gravitational lensing that mass reconstruction of the mass distributions within galaxy clusters is carried out, and when that is applied to colliding galaxy clusters — including the Bullet Cluster — you can see a clear signal that the mass distribution follows the distribution of the galaxy clusters themselves, not of the heated gas that’s been stripped out of them.

This has, for a long time, been treated as “smoking gun” proof of dark matter, as the mismatch between:
- where the mass signal is located,
- versus where the normal matter is located,
is extraordinarily significant, as their distributions are inconsistent at significances much greater than 5-sigma, which is traditionally used as the “gold standard” for discovery in astrophysics and particle physics. Regardless of the messy physics that is happening on galactic scales, when we look to larger scales — like the scales of galaxy clusters and in systems of colliding galaxy clusters — the evidence for dark matter over a theory of modified gravity is unambiguous.
Or is it?
In conversation with Prof. Xavier Hernandez, he mentioned two objections to the notion that the Bullet Cluster overwhelmingly supports the dark matter picture. In particular, he noted the following.
- We should not see it as surprising that we see lensing signals arising from where the matter is densest, not where it’s most massive, since it’s mass concentration that determines lensing signals, rather than overall matter.
- And that the speeds at which we observe the two clusters that comprise the Bullet Cluster colliding is too great to be consistent with our standard picture of the Universe: ΛCDM, or a dark energy and cold dark matter dominated Universe.
While these objections are common in the literature among MOND advocates, do they really hold water?
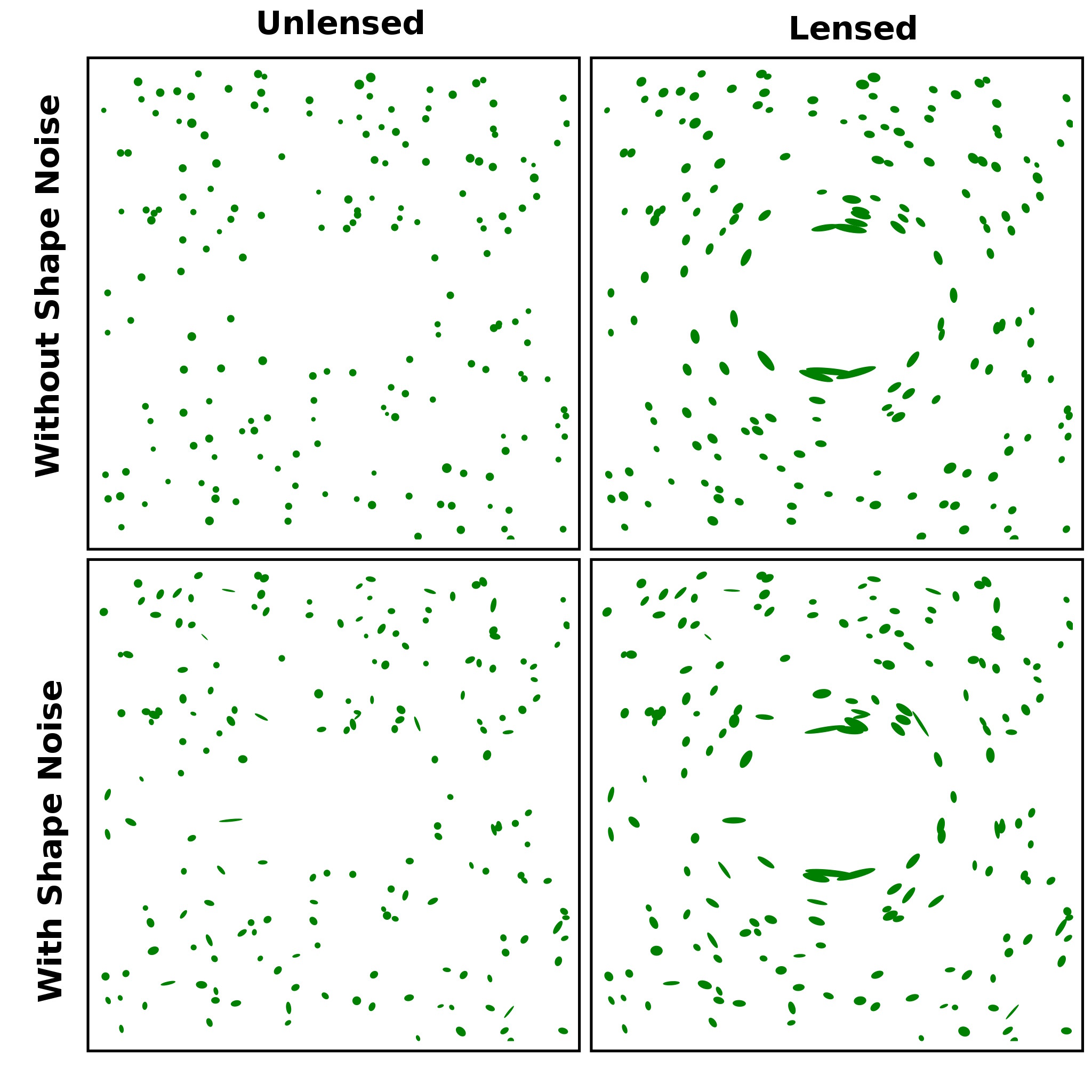
Does gravitational lensing depend on isolated “point” signals, or large-scale matter distributions?
From a physics perspective, the answer to this question is simple: it’s both. Dense concentrations of matter are required for creating the strong lensing signals that are so visually striking, and for the deflection of light that passes near individual stars, for example. These strong lensing signals can increase the magnification of a distant galaxy by factors of tens, hundreds, or even thousands, depending on the configuration, and can stretch individual point sources of light over enormous areas in the sky.
But even sparse concentrations of matter can play a significant role when it comes to weak gravitational lensing, and when we perform things like a “mass reconstruction analysis” of a galaxy cluster, whether of an individual galaxy cluster in isolation or of a set of galaxy clusters in collision, it’s the weak gravitational lensing signals that contain arguably the greatest amount of data.
This is something that’s been known very well for more than 25 years, in fact. There was (among cosmologists) a famous paper published in the journal Nature by Gus Evrard back in 1998, that showed quite surprisingly where the mass within a galaxy cluster is, as reconstructed from its gravitational lensing data. The key figure is shown below.
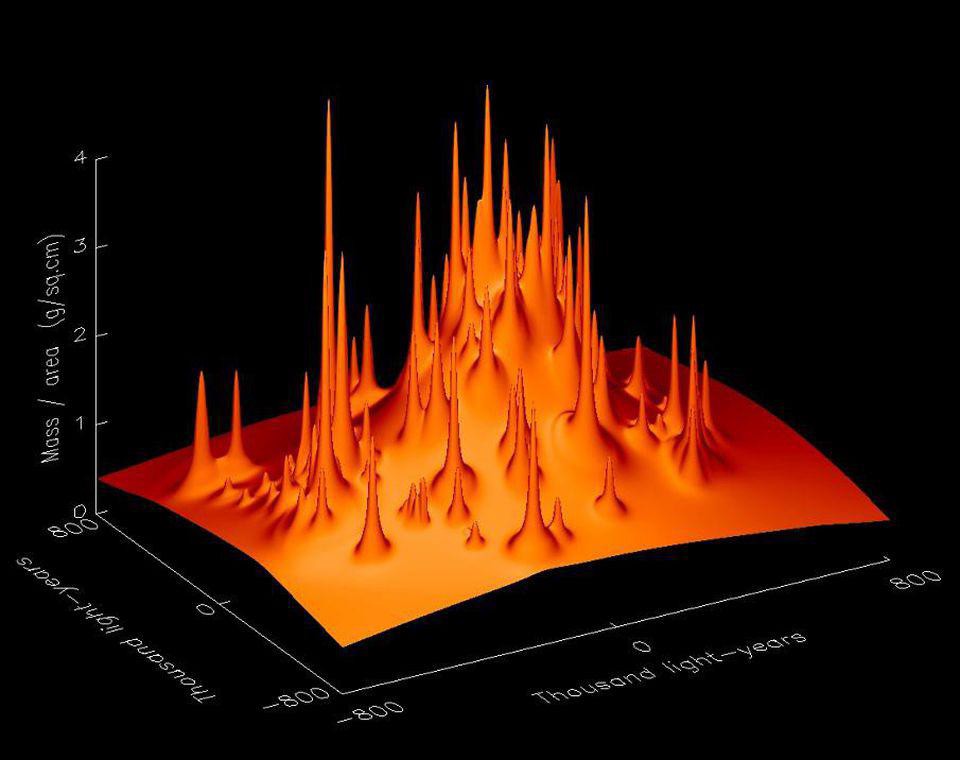
You will immediately, of course, notice the giant “peaks” of matter, which correspond to the lensing signals generated by individual galaxies. You’ll also notice, on the y-axis, that this is not a three-dimensional mass density (mass per unit volume) that’s being plotted, but rather a two-dimensional mass density (mass per unit area-on-the-sky) that’s being shown, as it’s the cumulative mass along a particular line-of-sight that generates a lensing signal.
But the biggest feature that you should notice is also the broadest: a smeared-out distribution of matter toward the center of this object. It’s as though not just much of the mass, but more than 80% of the cluster’s total mass, is not present within the individual galaxies, but rather in the space between them: in the intracluster medium. This is something that’s incredibly consistent with the dark matter hypothesis — that there’s an enormous amount of matter, more than all the normal matter combined — that surrounds galaxies and galaxy clusters in a giant halo.
Whereas the normal matter fragments and collapses into clumps, like galaxies and stars, the dark matter remains diffuse and “puffy,” as its non-collisional nature prevents it from forming dense structures.
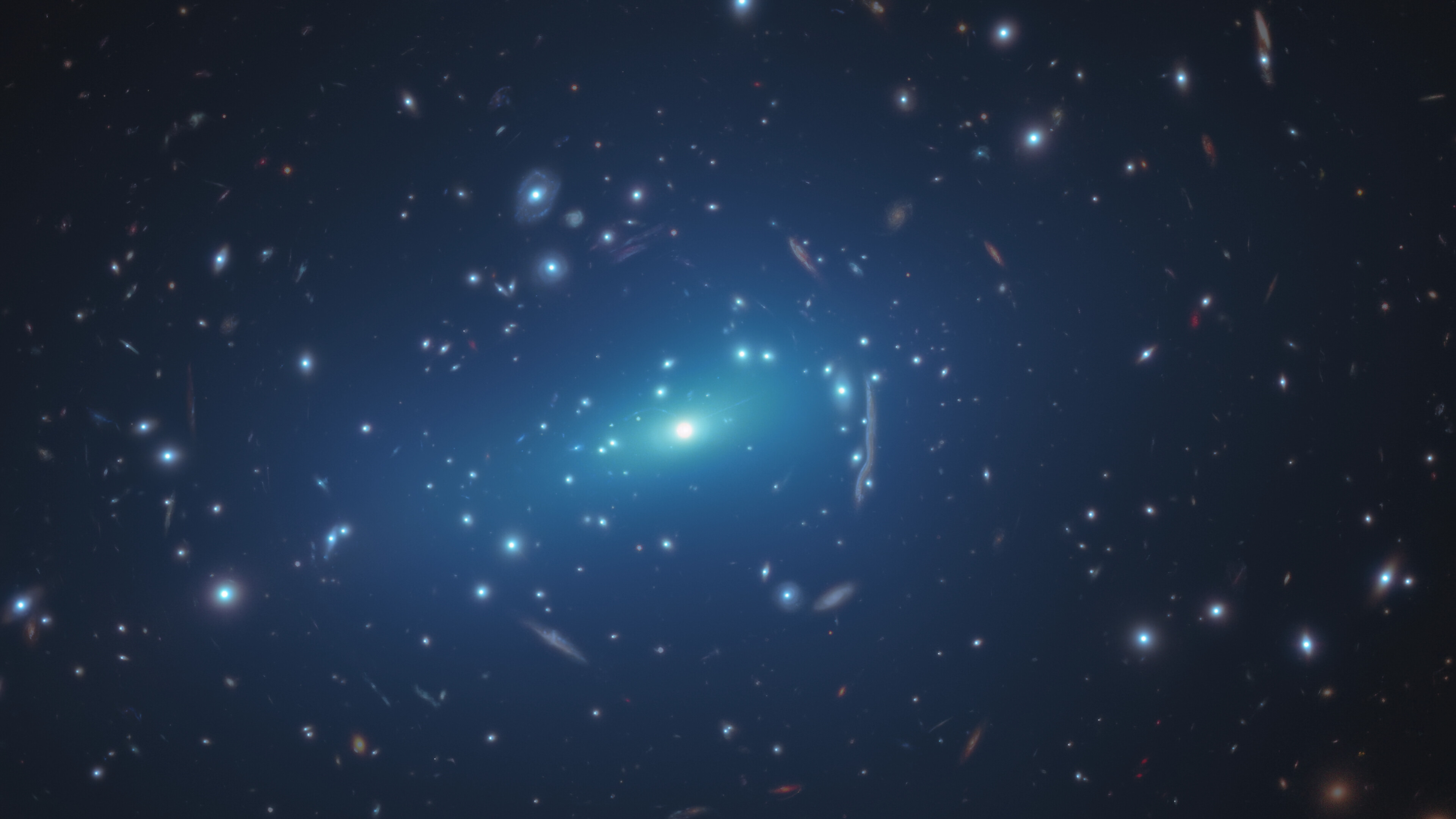
However, this doesn’t mean that dark matter doesn’t clump together at all! In fact, there’s a prediction, from simulations as well as from analytical treatments of structure formation, that every massive dark matter halo should be filled with dark matter substructure: smaller mini-halos with their own mass, their own concentration, and that are distributed all throughout the larger bound structures like galaxies and galaxy clusters. When we look at massive galaxy clusters, like MACS J1206, shown above, we can use the gravitational lensing data — both weak and strong lensing, combined — to reconstruct the mass and density profile of the foreground cluster mass itself.
Is it just:
- galaxies, which can be identified from light,
- plus the smooth distribution of a dark matter halo, which can be reconstructed from weak lensing,
- plus the intracluster light, identifiable as a diffuse blue glow,
and nothing more, that is responsible for the lensing signals we can identify? It turns out that no, that is not all there is. The intracluster light data, as well as the background weak lensing data, indicate the presence of “mass clumps” within this halo that go well beyond any galactic signals. From weak lensing and intracluster light, combined, we can tell that there really is not only an overall diffuse distribution of dark matter, but a “clumpy” distribution of dark matter substructure inside these halos as well.
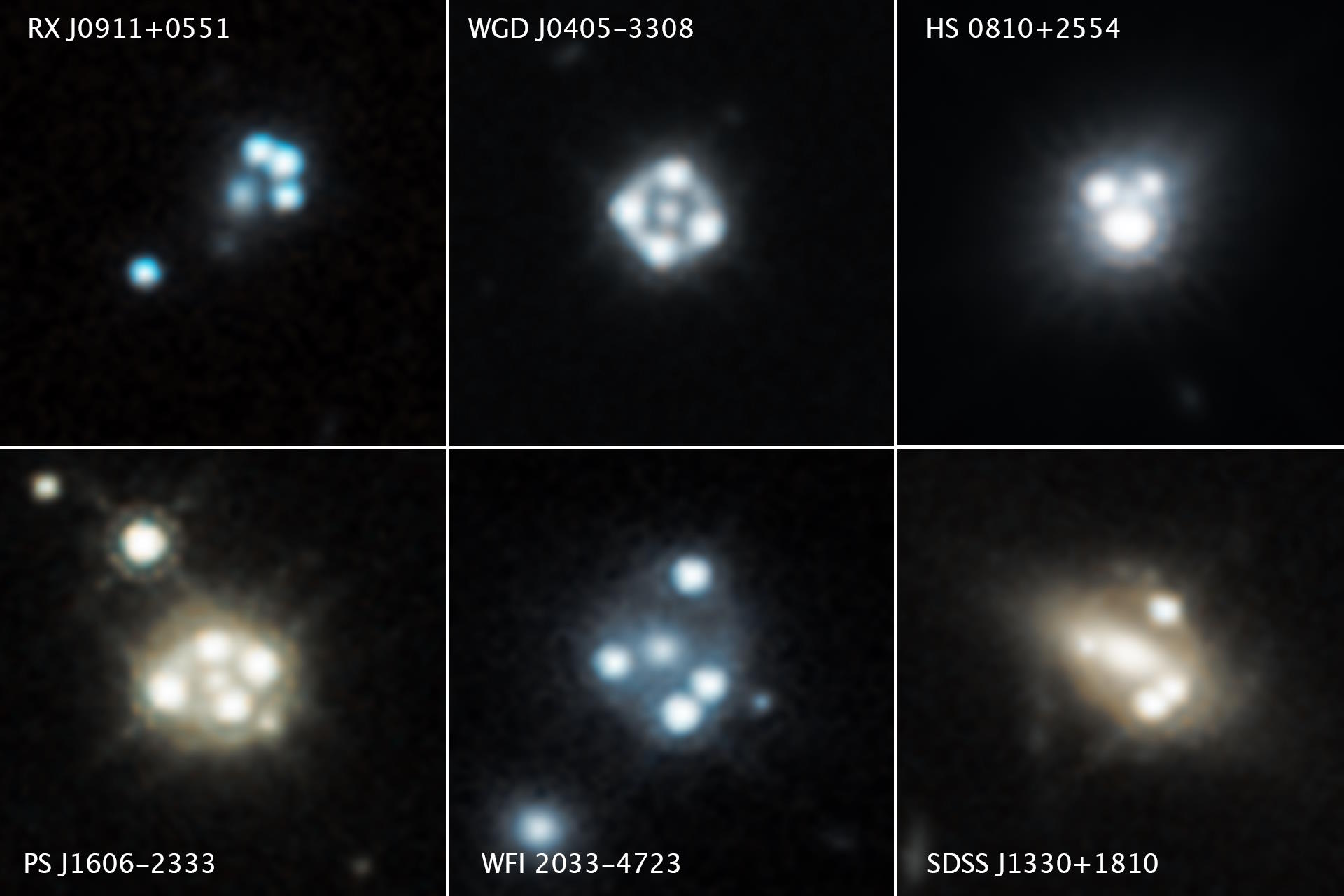
You can also look to strong lensing systems, including systems lensed simply by individual galaxies, to test the nature and presence of dark matter within galaxies. In quadruply-lensed systems, in particular, the magnification and brightness and size and location of the separate multiple images of each lensed, background galaxy can tell us whether the dark matter distribution within the galaxy is smooth, or whether there’s dark matter substructure present, as predicted.
This research was performed and published back in early 2020, and observed variations among the different images at the thousandths-of-a-percent level: which teaches us about the nature of the dark matter within these objects. In particular, dark matter cannot be born hot, or moving close to the speed of light early on, and it could not have been born warm either, which would “smooth” and “fuzz” out any individual substructure clumps, albeit not as severely as hot dark matter.
Instead, the dark matter must be cold, and the indirect evidence for these small-scale substructures comes from both weak and strong lensing data. These tiny dark matter clumps act as imperfections, or even “cracks,” in the gravitational lenses themselves. The evidence for them is now overwhelming, and combined with other lines of evidence from galaxies and galaxy clusters, shows that dark matter’s presence is not so easily explained away.
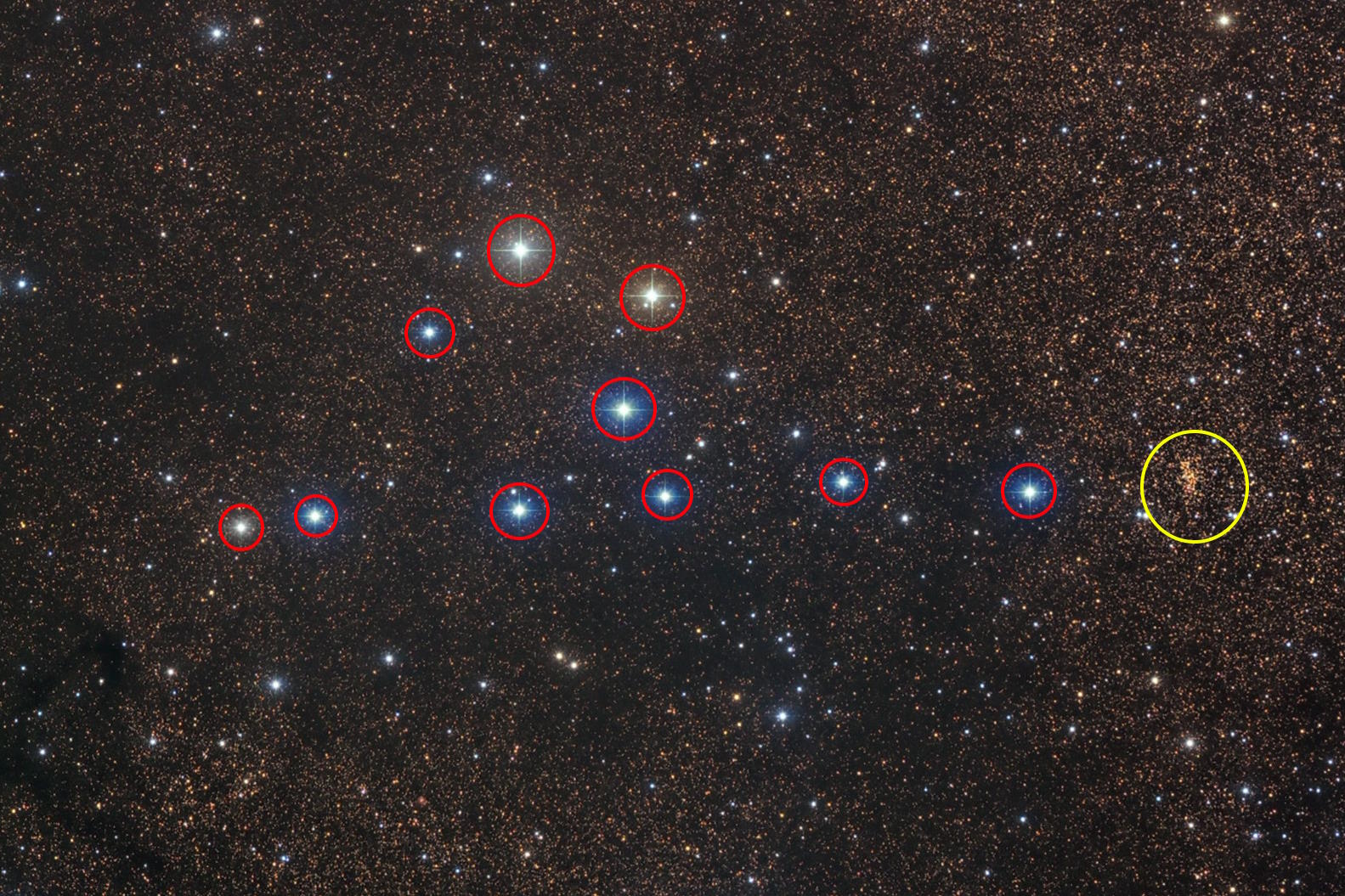
Is the Bullet Cluster an absurd cosmic rarity?
Take a look at this region of sky, shown above. Prominently visible through a telescope or binoculars in the months of July and August, this asterism, known as the Coathanger (or as Brocchi’s Cluster), consists of 10 relatively bright stars, all around magnitude 6 or 7, concentrated within one square degree of sky. Now, there are only about 40,000 stars this bright, or brighter, over the entire sky, and there are approximately 40,000 square degrees of sky altogether. You might think to ask yourself: what are the odds of finding ten stars, of this brightness, all within the same one square degree? And if these stars were randomly distributed, you’d find the odds were 1-in-10,000,000 or so. The chances that this would occur at random are small; you can rule that out at more than 5-sigma significance, for example.
But it turns out that all of these stars are unrelated; there is no “cluster” save for the one highlighted in a yellow circle: completely unrelated to the Coathanger asterism. It really is just a chance alignment of stars, however unlikely it may seem. Is the Bullet Cluster moving too fast to be consistent with our standard (ΛCDM) cosmology? Statistical arguments can be used the same way, arguing that the speed of these two clusters is too great to be consistent with our picture of the Universe.
As you might expect, different simulations give different results.
- Some say this one system is extraordinarily unlikely, and it’s likely to be disfavored at the more than 5-sigma level.
- Some say this system is somewhat unlikely, but not absurdly so; there should be a handful like it all throughout the Universe, and we happened to find it as our first colliding galaxy clusters.
- And others say this system is fairly run-of-the-mill, and only slightly faster, in collision, than you’d expect, and there’s no conflict with what we expect at all.
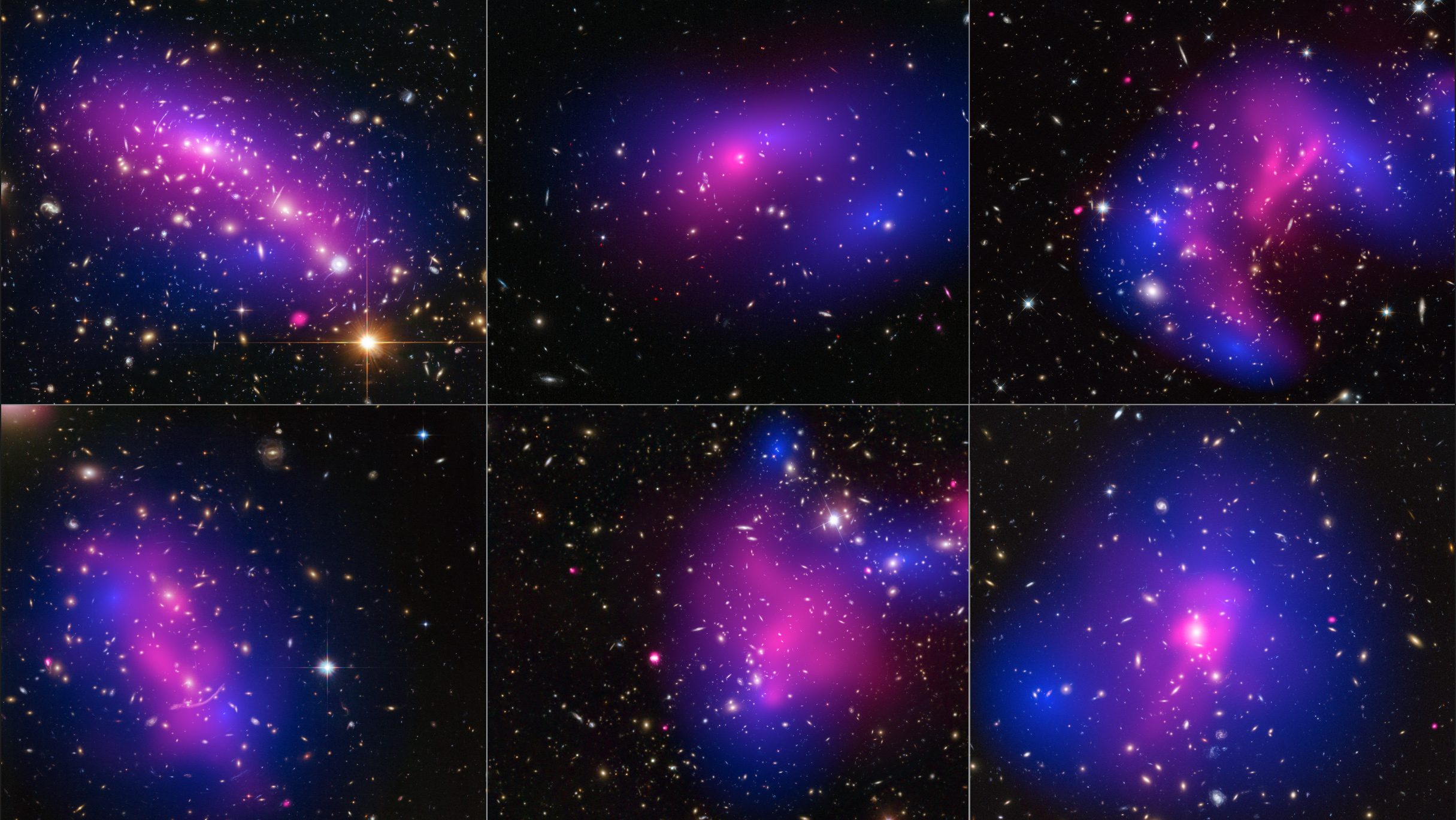
But we can do better than simulations; we now have dozens of these systems under our belt, with both gravitational lensing data (in blue) and X-ray emissions data (in pink) highlighting the differences between where the normal matter is and where the total mass is. In all systems, if you have evidence for heated gas (which arises from a cluster collision), then you are going to have mass offset from the distribution of normal matter. Speeds of these collisions are typically in the low thousands of km/s, but a few are faster; the Musket Ball Cluster, for instance, is comparable to the Bullet Cluster in speed. All display evidence for dark matter, and while some are fast, like the Bullet Cluster, the majority are of more modest speeds: more like the “standard” prediction of ΛCDM cosmology.
It remains true that on scales that are greater than that of a stellar and planetary system, of a few thousand times the Earth-Sun distance, up to scales of around a million or even a few million light-years, there are theories of modified gravity — including MOND — that may rival dark matter for how well they describe the Universe. But on larger cosmic scales, including (and, perhaps, especially) on the scales of galaxy clusters, all of these modified theories of gravity fail spectacularly unless they also include something that behaves identically to dark matter. We may not have directly detected it, and we may truly be in the dark when it comes to the question of what the Universe is made of, but the evidence against dark matter in galaxy clusters is flimsy, at best. The greatest objections to it, as the data overwhelmingly shows, simply don’t hold any water at all.