Could Right-Handed Neutrinos Solve The Dark Matter Puzzle?
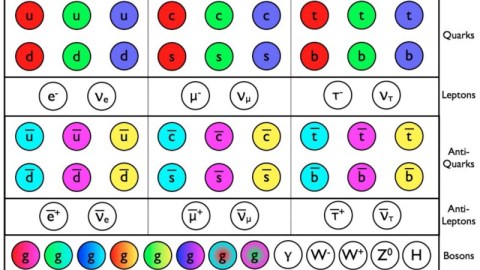
Forget those WIMPy WIMPs, and move on up to WIMPzillas!
“Eight solid light-years of lead…is the thickness of that metal in which you would need to encase yourself if you wanted to keep from being touched by neutrinos.” –Michael Chabon
The Standard Model of elementary particles is complete. Every particle predicted within it:
- the six quarks and antiquarks in three different colors each,
- the three charged leptons (electron, muon and tau) and their corresponding neutrinos,
- the six anti-lepton counterparts,
- and the eight gluons, three heavy weak bosons (W+, W-, and Z), the photon and the Higgs boson,
has already been directly detected. But despite the Standard Model’s success, and the overwhelming success of experimental particle physics, there are mysteries of the Universe that haven’t yet been solved, and two of them might be related.
One of them is dark matter. All the “known” matter in the Universe — the radiation from photons, the normal matter from quarks, gluons and electrons, and a tiny bit of hot dark matter from neutrinos — comes from the Standard Model. But if you add all of it up, and you run a simulation of what you’d get out, you don’t get a Universe like ours. Instead, you’d get one where galaxy formation is suppressed, where star formation tears young galaxies apart, where heavy elements get blasted into intergalactic space and never forms rocky planets, and where the large-scale structure of the Universe looks vastly different.
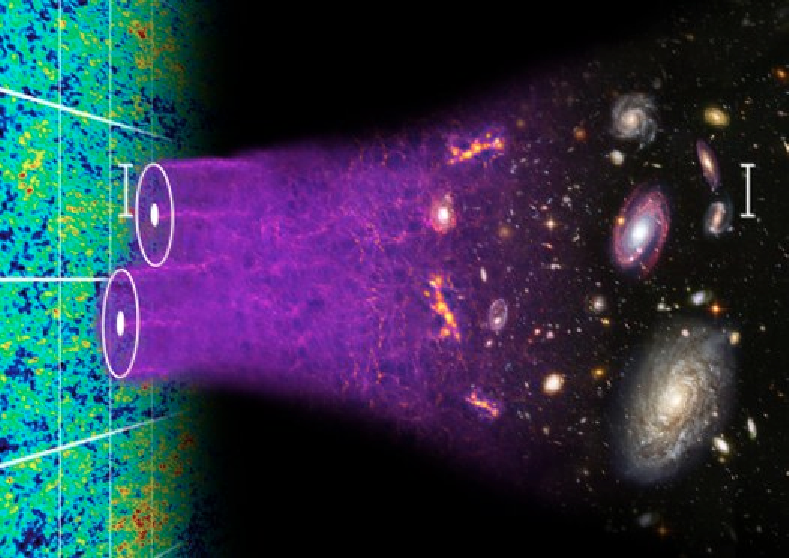
In order to get the Universe we see and know today, including to give you:
- the observed fluctuations in the cosmic microwave background,
- the small-and-large-scale clustering features of galaxies,
- the rotation profiles of spiral and elliptical galaxies,
- the gravitational lensing effects of galaxy clusters, along with many more observations,
you need an extra type of matter in addition to what the Standard Model predicts: some type of dark matter. This dark matter must be about five times as abundant as all the normal (Standard Model) stuff combined, it has to be massive, it has to clump and cluster together, and it has to be moving slowly compared to the speed of light. All sorts of indirect evidence exists for dark matter, but we’ve never directly detected it. In order to find out what its nature actually is, we’ll have to do just that.
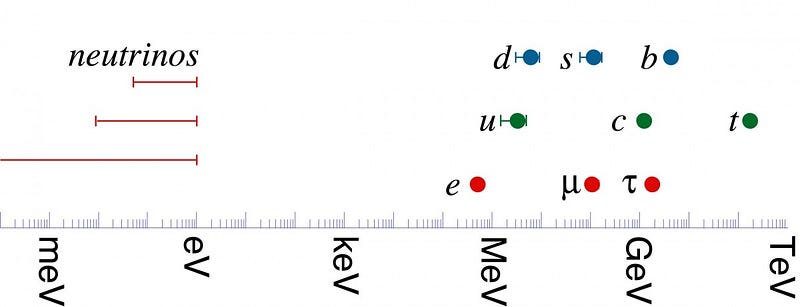
A second mystery is the masses of neutrinos. All the other particles in the Standard Model either are completely massless (like the photon or gluon), or have a substantial mass that falls somewhere within a relatively large but well-defined range. The lightest particle, the electron, is about 511,000 electron-Volts in mass, while the heaviest, the top quark, is somewhere around 175,000,000,000 eV. This might seem like a big range, but a factor of less than 400,000 to cover all the particles is a pretty good deal.
For a long time, the neutrino was thought to be massless, too. But recent experiments have found that all three types — the electron, the mu and the tau — all have very small but non-zero masses, weighing in somewhere around the milli-electron-Volt range, or at least a factor of ten million times lighter than the electron!
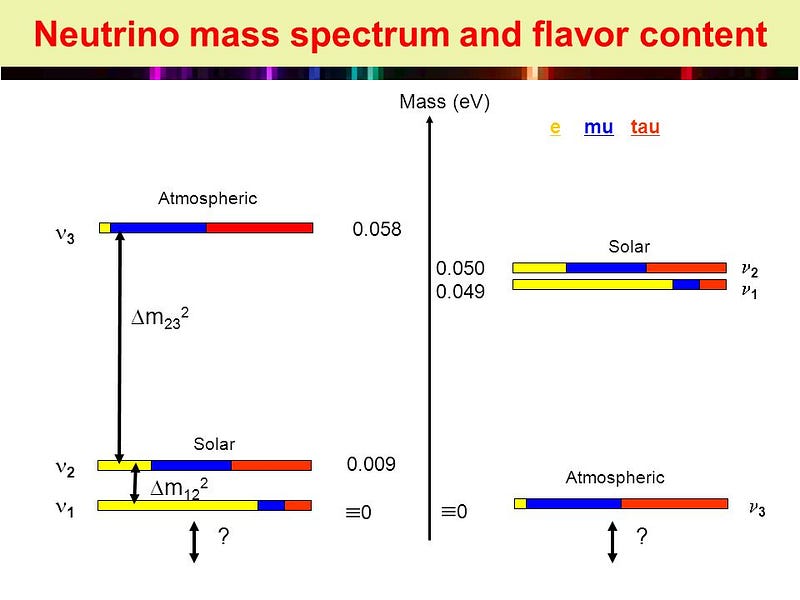
For particles that were predicted to be massless, this is a problem! Why would they not only have mass, but why would their masses be so remarkably tiny? One of the leading ideas — first put forth by a number of scientists in the late 1970s — is that neutrino masses could work like a see-saw! You see, the neutrinos that we see are all left-handed, meaning that if you orient yourselves in their direction of motion, they’re all seen to spin in the same fashion. Similarly, all the anti-neutrinos are right handed.
But if you assume that there’s a very large mass scale in nature, like a grand unification scale, then the neutrinos (both left and right-handed) could have had a “normal” mass like the other Standard Model particles, where they were sort-of balanced on a see-saw. But then that heavy mass comes along, sits on one side of the see-saw, and splits them: the left-handed neutrinos become very light, while the right-handed neutrinos become extremely heavy.
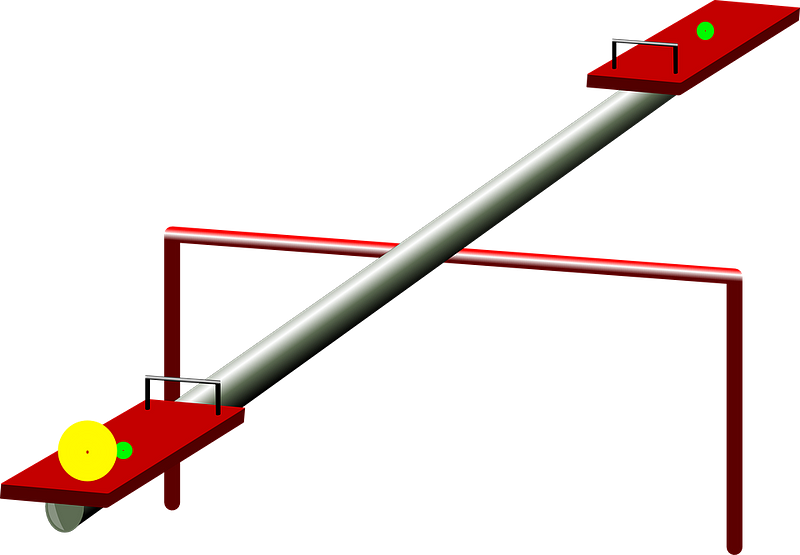
This is the leading explanation for how neutrinos oscillate, and also how they acquire such tiny (but non-zero) masses. But rather than hypothesizing supersymmetry, extra dimensions, axions or some other exotic solution to dark matter, here’s a fun possibility: the ultra-heavy, right-handed neutrinos could actually be the dark matter! Instead of being in the same range as the neutrino masses (like axions) or in the same range as the other Standard Model particles (like in SUSY or extra dimensions), they might be super-heavy: billions or even trillions of times heavier than the other Standard Model particles. This new class of dark matter candidate has a fantastic name (coined by Rocky Kolb): WIMPzillas!
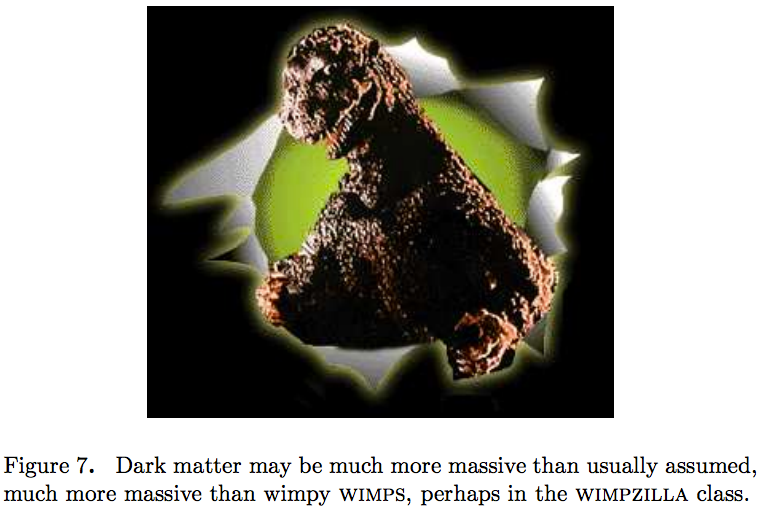
What’s remarkable about this possibility is that it comes about from already known physics, and explains a problem — neutrino masses — that has no other good, known alternative explanation. In theoretical physics, one of the great motivators to any unsolved problem is a possible solution to a completely different unsolved problem, and WIMPzillas are an underappreciated possibility for dark matter. If true, they could explain everything that’s missing in the Universe — all of the missing mass — and give us the galaxies, clusters, and large-and-small-scale structure that we see today.
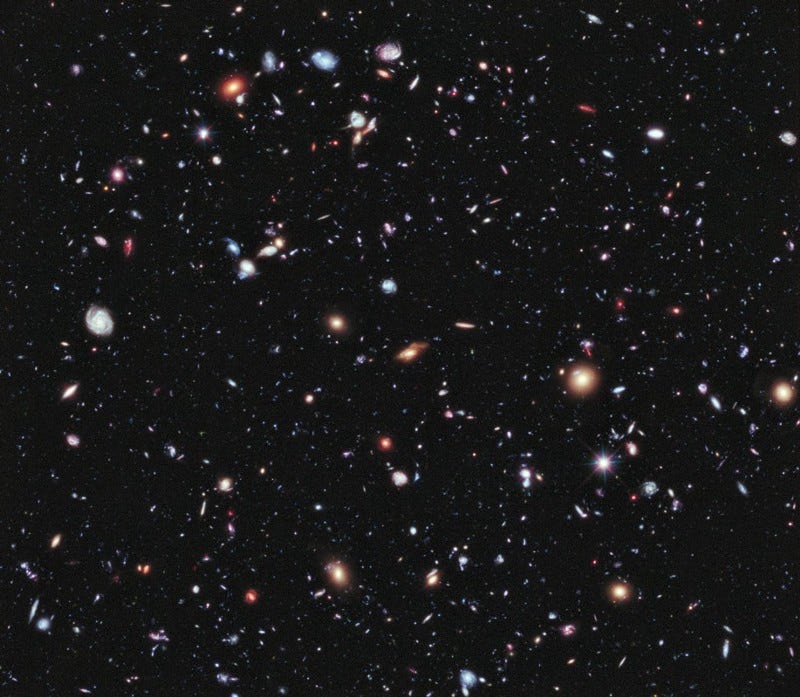
In the quest for dark matter, it’s important not just to think small, but to think very, very large as well!
This post first appeared at Forbes. Leave your comments on our forum, check out our first book: Beyond The Galaxy, and support our Patreon campaign!