Astronomers near a complete picture for how planets form
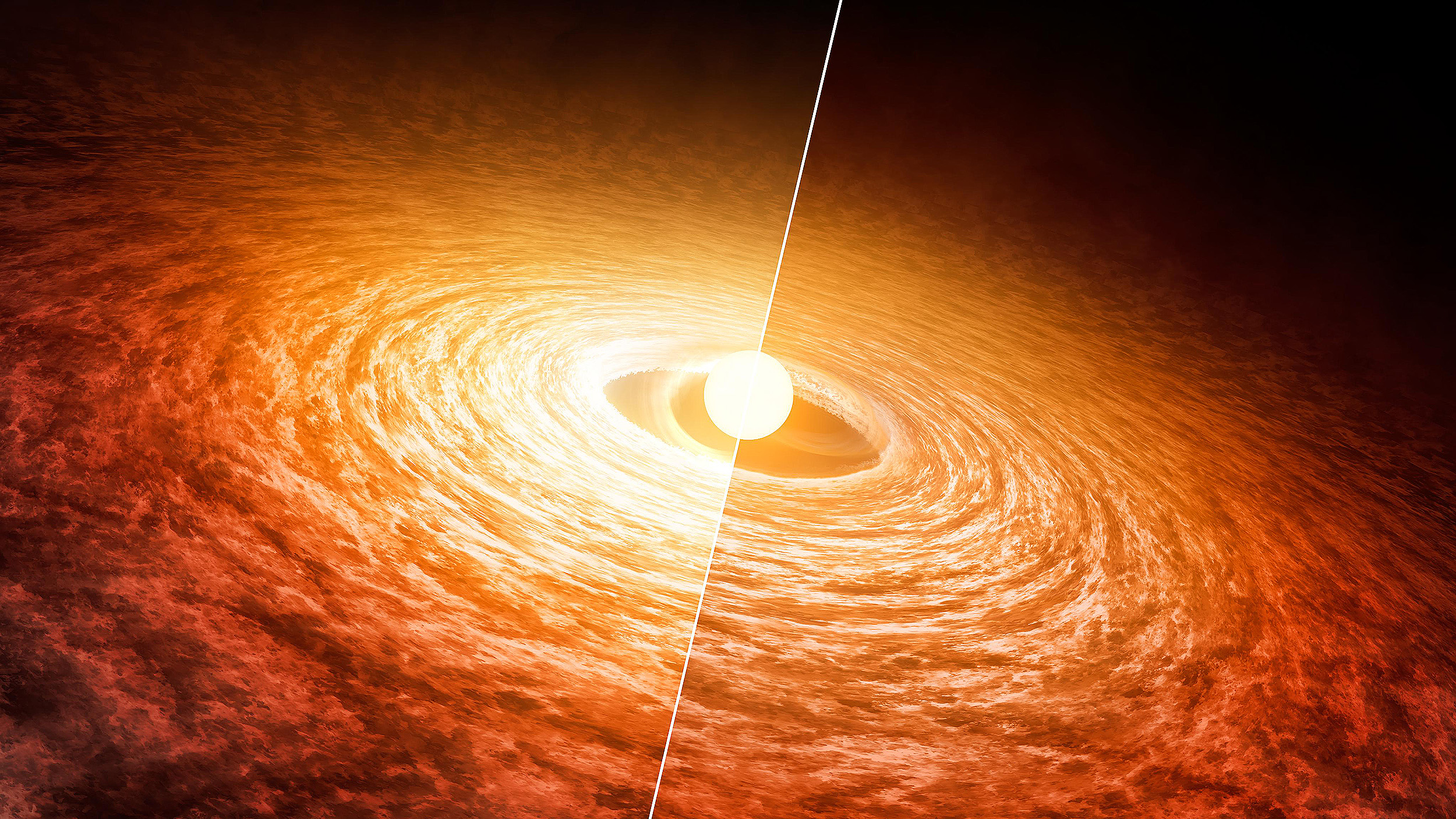
- When we look at the planets in our Solar System, including our own planet Earth, we find that they formed nearly at the same time as the Sun: all are ~4.5 billion years old.
- But around newborn stars, the situation evolves rapidly: from protostars to stars with circumstellar disks to planet-rich systems with debris disks to mature planetary systems.
- With so much data about circumstellar and debris disks now collected, a complete picture for the formation of planets is finally starting to emerge. Here’s what the cosmic story of planet birth looks like today.
For a species that grew up on life-giving planet Earth, it’s a wonder that we still don’t have an end-to-end scientific picture for how planets actually form in this Universe. Even with our most advanced observatories, like NASA’s Hubble and JWST, we’ve only ever obtained “snapshots” of stellar systems at a variety of ages and stages, including:
- the protostar stage, before nuclear fusion has ignited in the star’s core,
- the initial fusion stage, where stars have ignited but their circumstellar disks remain featureless,
- the initial planet-forming stage, where circumstellar disks start to display evidence that planet-formation has started,
- the end of the circumstellar disk phase, where the gas-to-planet mass ratio drops from ~100-to-1 down to a more modest ~1-to-1 ratio,
- the debris disk phase, where dust-and-gas-rich material, as well as numerous smaller bodies, persist for several hundred million years,
- and finally, to the mature planet phase, where planets are no longer growing and remain in a relatively stable (or quasi-stable) long-term configuration.
It’s only in very recent years, since the advent of the infrared-sensitive JWST and the radio capabilities of ALMA combined, that we’ve been able to piece together a complete picture of these various stages, including what happens and when, with extraordinary implications for our own Solar System. Here’s the most complete picture we’ve been able to assemble as of today.

A cloudy beginning
It might seem like it’s an unlikely story, but every star — as far as we know, at any rate — began its life initially as a cloud of gas. (Notwithstanding the stars that are born from the merger of two pre-existing stars or brown dwarfs.) Clouds of gas are often both massive and warm, where the kinetic energy of the gas is great enough to counteract the gravitational potential energy binding the gas together: preventing further collapse. However, over time, based on the heavy elements and molecules (both simple and complex) inside of them, heat gets radiated away, diminishing the kinetic energy of the gas and leading to a star-forming scenario: where the gas cloud undergoes fragmentation and gravitational collapse.
Over a relatively short time of a few million years to perhaps a few hundred million years, gravitational collapse proceeds in a variety of locations, where the various regions where collapse occurs can contain as little as one or two solar masses, or as much as tens of millions of solar masses. Stars can form in isolation, in small groups, or in clusters with anywhere from hundreds to tens of millions of newborn stars. In each stellar system — i.e., where each new star is born — an initially asymmetric clump of matter contracts down to a massive core, where one or more stars will form, surrounded by a two-dimensional disk of material: material that typically touches, and contacts, the massive core at the center of it all.

From protostar to full-fledged star
One of the paradoxes of star-formation is this: in order to gravitationally collapse, gas needs to cool, but in order for nuclear fusion to ignite within a star’s core, temperatures need to be remarkably high, at 4,000,000 K or above. How can you go from a cool, even cold cloud of gas to a dense, collapsed ball of that same material at extreme temperatures?
Remarkably, the answer is simple and straightforward from an astrophysics perspective: from gravitational collapse. Remember, in order for a gas cloud to cool, it has to radiate heat away: radiation that can only propagate away from a region of space through the surface area that encloses it. As gravitational collapse occurs, the volume that the material occupies, as well as the surface area that encloses that volume, both shrink, making it more difficult for that heat to radiate away. As a result, that heat becomes trapped: a consequence of adiabatic contraction.
Even though there’s nothing driving away the surrounding gaseous matter, the central core of the protostar continues to see its temperature rise and rise, while the density increases as well. Eventually, the core temperature crosses over the critical ~4,000,000 K threshold, and nuclear fusion ignites, fusing protons and protons together in a chain reaction to trigger the official birth of the star.

The undifferentiated circumstellar disk phase
At last, the star has ignited, and now the radiation and pressure emerging from it increases spectacularly. At the start of this phase, the circumstellar disk is completely attached to the star, meaning that matter continues to accrete onto the protostar, and that the particles and photons emitted by the star are insufficient to stop the continued growth of the star itself. Meanwhile, the disk surrounding the star remains featureless and undifferentiated: hot, swirling, and without any massive perturbations — i.e., protoplanets — that are detectable even through direct observation with the greatest tools at our disposal.
But depending on the particulars of the system in question, this phase will only persist for somewhere between half-a-million and two million years. At some point, a protoplanetesimal will grow large and massive enough that it will begin carving features, like a spiral pattern, a gap, or even a ring within the disk itself. Additionally, a “gap” will first appear between the young star and the disk itself: separating the star from the circumstellar disk that enables the formation of planets, with the interior edge of the gap normally falling well inside the “soot line,” or close enough to the star that even heavy chemical compounds are dissociated by the energy and particles emitted by the star. The emergence of gaps and features within the disk marks the end of the undifferentiated phase.

The chaotic planet-forming phase
These circumstellar disks, or disks that surround the star, are also known as protoplanetary disks: the locations where planets are formed. Once the star ignites, the material within the disk is caught up in a race against time, as in just a few million years (generally thought to last about ten million years), the majority of the gas within the disk will be blown away from the central star’s radiation. This means we have:
- initial imperfections,
- which seek to grow by gravitational attraction,
- drawing on matter from within their orbit, as well as exterior and interior orbits,
- to produce protoplanets and eventually full-fledged planets,
- while surviving gravitational encounters with the other objects that are racing to do the same,
- for as long as the gaseous matter persists in great abundance where it is.
In the chaos of these early environments, many interactions will occur. Small protoplanetesimals will collide: sometimes binding together to form larger protoplanets, sometimes smashing into one another and blasting their bound material apart. Young planets and protoplanets will gravitationally interact, where they can combine, be ejected, collide, or get hurled into the central star. The largest initial planets gobble up the material around them, becoming the largest worlds in their stellar systems, and the migration of planets perturbs the orbit of everything nearby, often destroying these lower-mass worlds and preventing them from becoming surviving planets.
And yet, despite this initial chaos, once enough time goes by, a more stable-looking system emerges. After approximately 10 million years have gone by, the gas-to-planet mass ratio will have dropped substantially: from an initial ratio of around 100-to-1 (or greater) down to a more modest 1-to-1, indicating that the planets within this stellar system have now largely taken shape.
In our own Solar System, we typically assume that the four gas giant planets we know today — Jupiter, Saturn, Uranus, and Neptune, as well as possibly one or more additional large planets — all formed during this very early phase, with Jupiter initially being much closer to the Sun than it is today. Whatever the initial planetary configuration was in the inner Solar System, it was likely destroyed, absorbed, ejected, or hurled into the Sun by Jupiter.
But even with relatively low amounts of gas remaining, the outward migration of Jupiter paved the way for smaller bodies to form and survive within the inner Solar System: bodies such as the ones that would become the modern-day Mercury, Venus, Earth, and Mars. That’s because planet-formation doesn’t end abruptly or all-at-once, but simply transitions to a different stage once the gas has mostly disappeared.
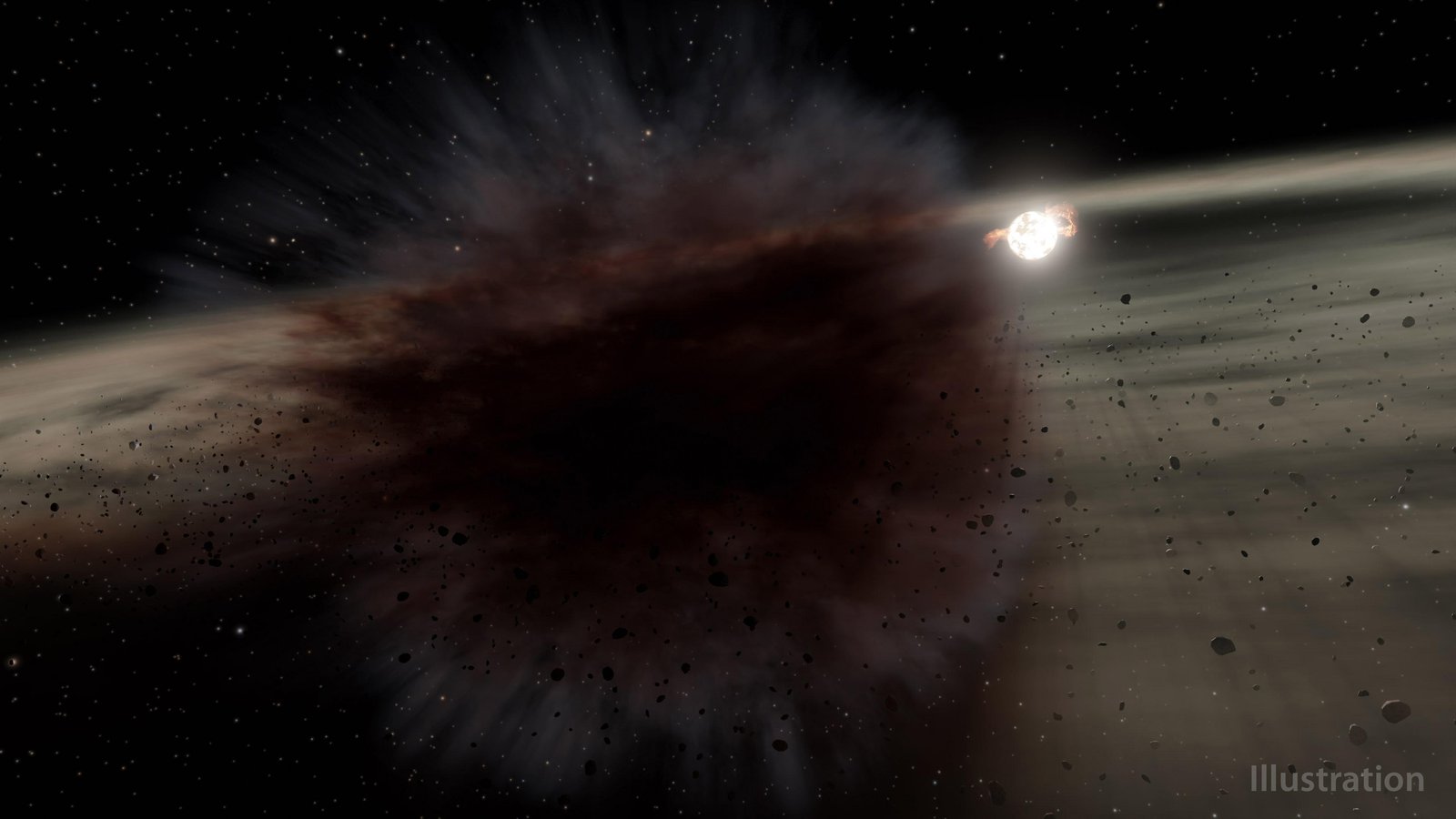
The debris disk phase
Once the free gas from the initial disk that surrounded the newly-formed star has almost completely blown away, planet-formation is over, right?
That naive picture was, in many ways, the leading idea in planet formation for several decades, so if this is what you thought, too, know that you weren’t alone. In fact, this is probably true for the majority of large, massive planets that formed in the Universe. (Although, over the next tens to even hundreds of millions of years, gas giant worlds that have already formed can continue to grow much more massive: a scenario that likely applies to our own Solar System’s Jupiter and maybe even Saturn, but not so much to Uranus or Neptune.)
Instead, we now know that the material that remains in a young planetary system includes:
- some gas,
- young planets and planetesimals at a variety of distances from the star,
- an enormous number of solid bodies at various “frost lines,” or when different compounds transition from solid-to-ice or vice versa,
- and a great number of solid bodies in the outer system, at Kuiper belt-like distances and beyond.
(It’s also possible that intermediate belts are common; more needs to be learned about this.)
This stage in the evolution of stellar systems represents a time of heavy bombardment of planetary bodies by comet-like and asteroid-like bodies, as well as a time where there’s great potential for giant collisions.
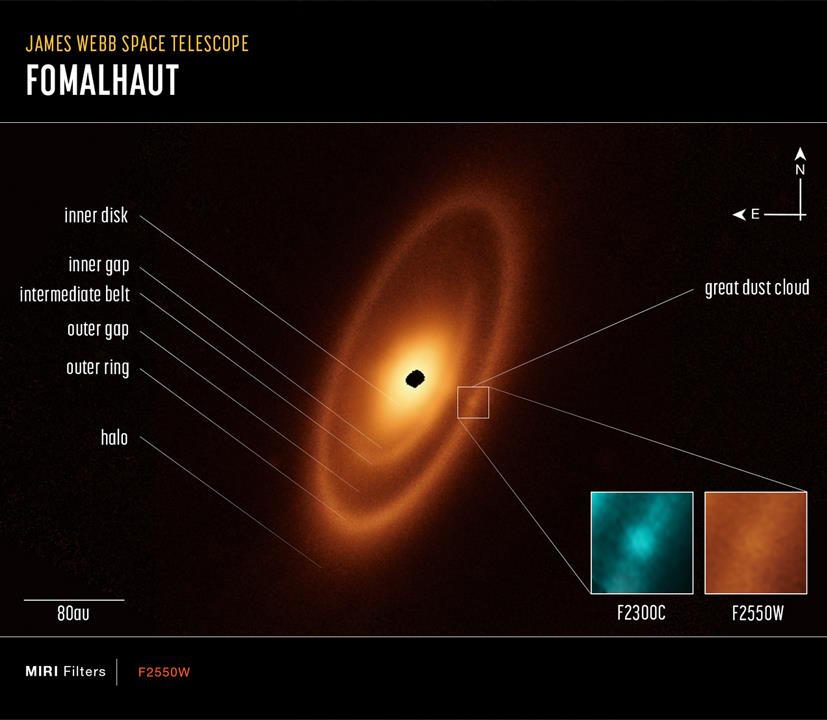
Bombardment and its gradual end
If you grew up in the late-20th or early-21st centuries, you probably learned about a time in our own Solar System’s history known as the “late heavy bombardment,” which lasted until about 700 million years after the formation of the Sun and planets. Evidence for this period exists across the ancient surfaces of many well-known bodies, from the Moon to Mercury to Mars and more. However, it’s kind of a misnomer in a sense: nothing happened that was all that special to increase the bombardment rate that late in the game. Instead, there was a massive bombardment period that persisted for the entirety of the first ~700 million years of our Solar System’s history, and it’s more like the extreme violence ceased about 3.8 billion years ago: right around the time that the first smoking-gun evidence for life on Earth appears in the fossil record.
Many violent events occurred during this bombardment period. About 50 million years after the circumstellar disk phase ended, a giant collision between young Earth and the protoplanet Theia occurred, giving rise to our Moon. Mars experienced a giant impact, giving rise to Phobos, Deimos, and a now-destroyed, massive third moon that fell back onto Mars. Pluto received a great impact, creating its system of five moons. Triton, once the largest and most massive body in the Kuiper belt, migrated inward and became gravitationally captured by Neptune. Only after all of these events occurred did bombardment end, signaling the end of the debris disk phase of our Solar System’s history.
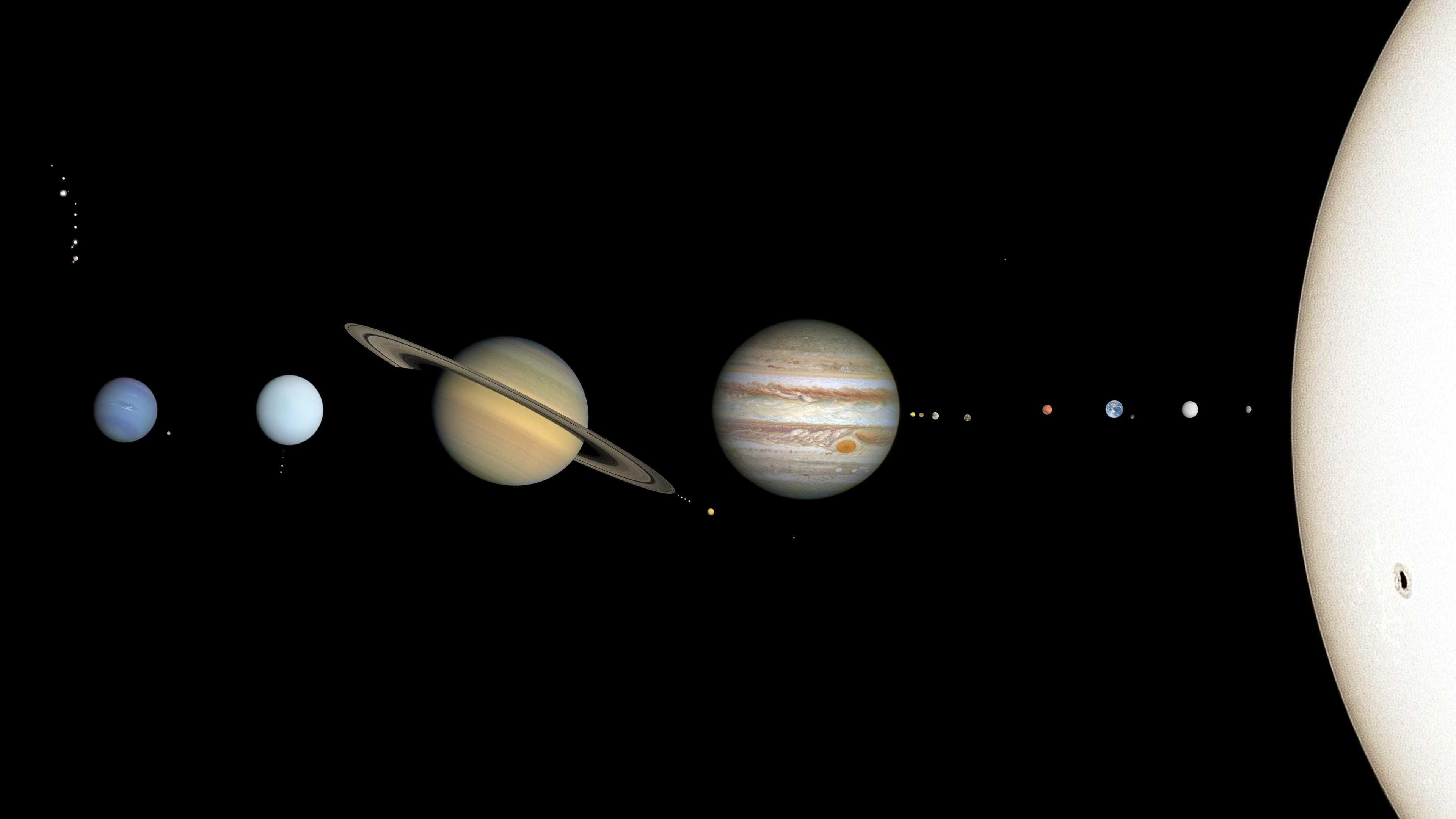
Mature planetary systems
Just as humans go through a variety of phases as we grow up — from infancy to toddlerhood to childhood-and-adolescence to adulthood — so, it appears, do stellar and planetary systems. During the protostar and undifferentiated circumstellar disk phase, planets are not yet conceived: the seeds for them are destroyed just as easily as they are created. As the circumstellar disk begins to differentiate, and it is not yet known whether the earliest differentiations that occur lead to planets or get completely destroyed, planetesimals, or the seeds of planets, get created. They grow rapidly, like a gestating human fetus in the womb. During this phase, planetary miscarriages are common: getting devoured by other, larger baby planets, getting ejected, or even getting hurled into the parent star.
Then, when the circumstellar disk phase gives way to the debris disk phase, these baby planets start to grow up, going through a long childhood and adolescence, experiencing many violent episodes (where moons around rocky planets are primarily formed), and where a few lucky, tiny, leftover planetesimals can persist as full-fledged planets, much the way Mercury and Mars likely did.
And then, finally, when the debris completely clears out, which can occur quickly (within a couple of hundred million years) or which can take a long time (nearly a billion years), the planets that are left are fully mature, with only the rare gravitational interaction upsetting the orbits of the planets over the subsequent billions of years.
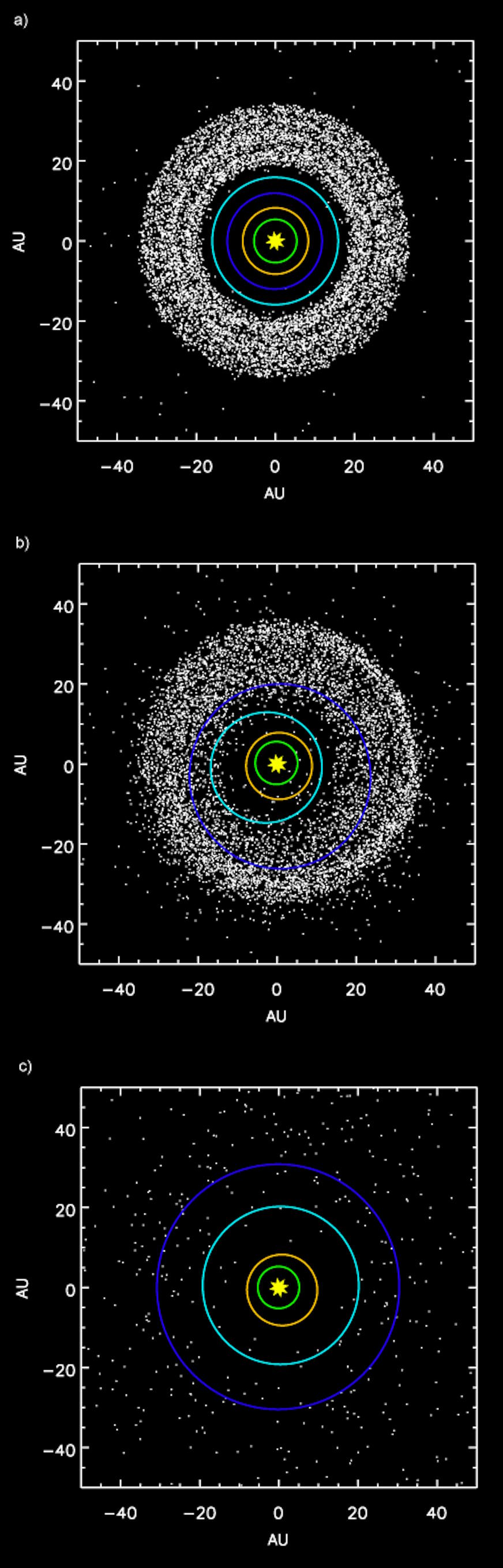
There are still a great many details that need to be investigated about how planetary systems form and grow up, but at this point in time — in mid-2024 — we have now assembled a more comprehensive and detail-rich picture about the formation of planets than ever before. Yes, very high-mass and very low-mass stellar systems are likely to deviate from this standard story, and there’s likely to be a lot of variation among individual systems even within this story, just as there are always going to be a few extremely tall, or short, or heavy, or light humans within a large enough population at any given stage of development. However, for the first time, we can assemble a detail-rich story of planetary evolution that’s driven by a wealth of data that was unavailable to astronomers prior to observatories like ALMA and JWST.
The story, quite simply, is one of the evolution of matter from a uniform, undifferentiated disk around a protostar to one that’s virtually gas-free that simply contains mature planets and planetary systems orbiting around one or more stars in a stable fashion. The transition from a circumstellar disk phase to a debris disk phase, as well as the persistence of a debris disk over long periods of time, remains poorly understood, and it’s hoped that future observations of these young systems will help us fill in the gaps we presently have. Nevertheless, we have a compelling story for planet formation across the Universe that is, at last, consistent with our own Solar System’s history. Now that we have an outline for how planets and planetary systems form and evolve, it’s time to find out just how rich the tapestry of existence can truly be.