Ask Ethan: Can gravitational waves let us peek inside a black hole?
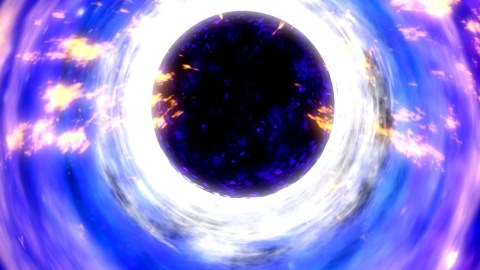
If they change and distort spacetime as the ripples pass through, could what was once inside actually get out?
“Find a place inside where there’s joy, and the joy will burn out the pain.”
–Joseph Campbell
Since LIGO first directly detected gravitational waves from merging black holes, scientists have taken a renewed interest in learning all about them. After all, with new data, a new technique and a new way of looking at the Universe, perhaps there are a whole slew of new discoveries that are now possible. One of a black hole’s fundamental properties, of course, is that nothing can exit its event horizon from inside, since the escape velocity in a black hole’s interior is greater than the speed of light. But perhaps that can be overcome? Patreon supporter Robert J. Hansen wants to know if there’s a way of viewing what’s inside:
“If spacetime distortion can in effect boost the speed of light, is it possible for a passing gravitational wave to alter the event horizon of a black hole, giving us a way to observe the contents due to a temporary boosting of c?”
Let’s take a look at the physics and find out!
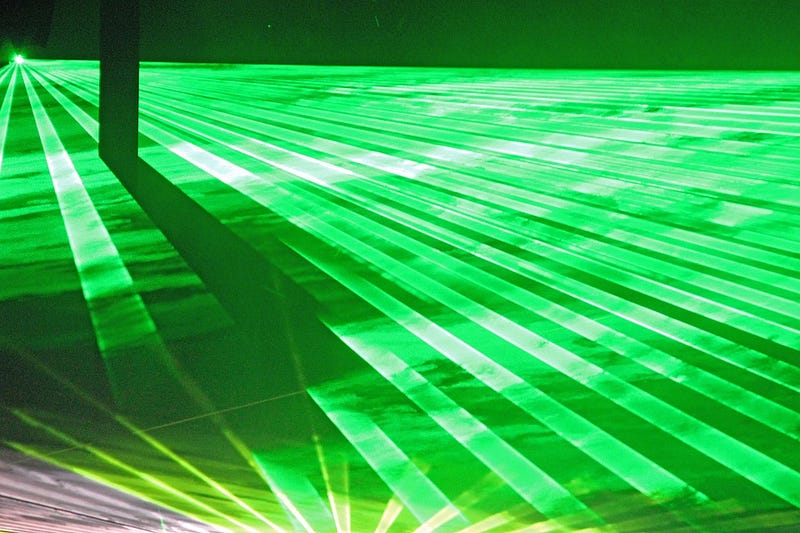
You’ve no doubt heard that the speed of light in a vacuum, the universal constant of c, is a constant no matter what. In special relativity, this is strictly true; if your space is completely flat, there’s no way around this. You can set up, theoretically, an infinite series of observers, spaced a fixed distance apart, at rest with respect to one another. As a light wave goes by, the amount of time it takes each observer to see that light signal is fixed: the time it takes the light to go from 1 to 2 is the same as from 2 to 3, as 3 to 4, and from 99 to 100. There’s no disagreement and no ambiguity, and so everybody’s happy.
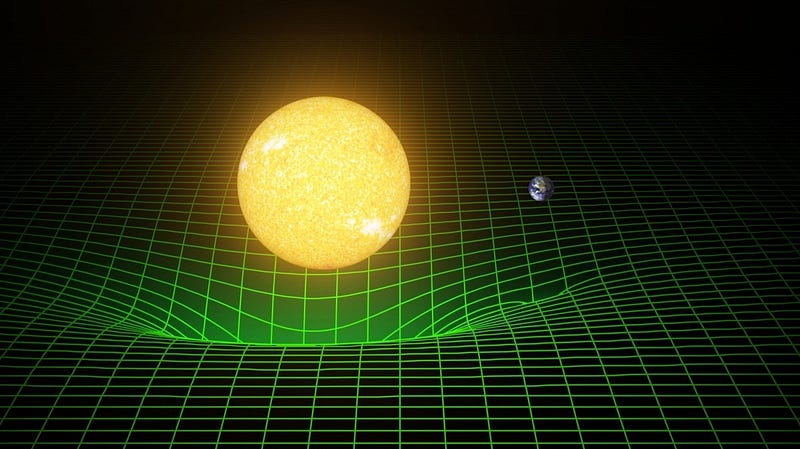
But things get hairier once you allow space to be curved, which is the big difference between special and general relativity. If you try and place that same infinite series of observers a fixed distance apart and at rest with respect to one another, they’re going to fight. Not because of any interpersonal differences, but because their observations won’t agree with one another as to what constitutes a “fixed distance” or what “at rest” means. When the light signal passes by each observer, they each measure that signal’s speed to be c, just like you’d expect, but they can’t agree with one another as to what happens at the locations that aren’t their own. There’s no common standard of rulers and clocks that apply equally to all observers once you allow space to be curved.
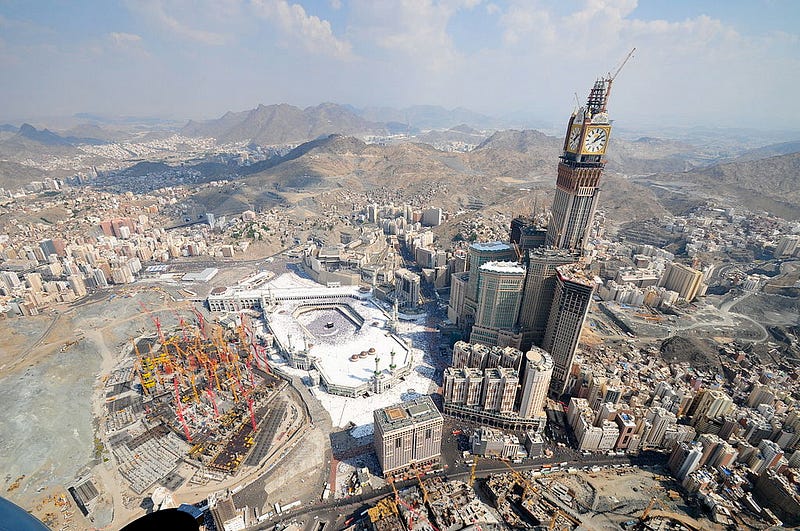
This is the same reason why, if you place an atomic clock at the bottom of a building and an identical atomic clock at the top, you’ll find that they run at slightly different rates. It’s not that one clock is flawed; it’s that the non-zero curvature of space makes it so that different observers disagree as to what makes a good “clock” at any location other than their own!
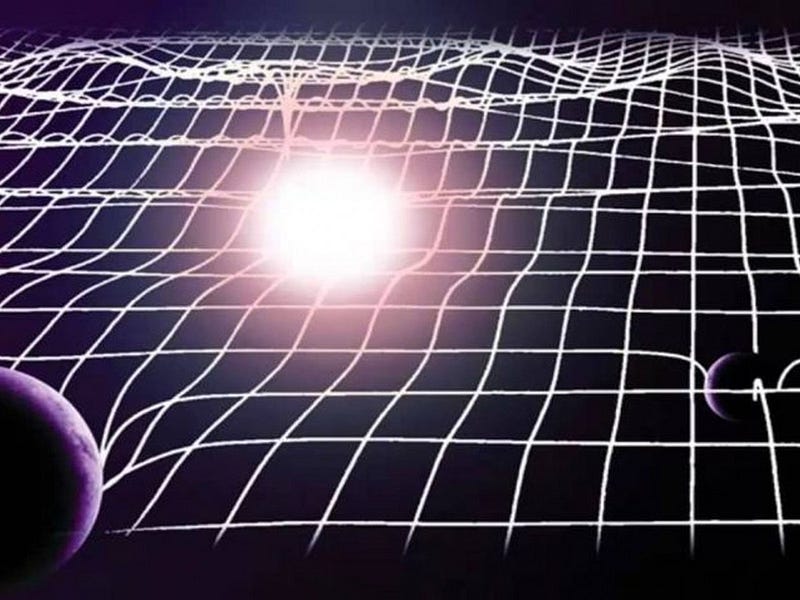
When a light signal passes through a region of curved space, a distant observer might see that signal as moving faster than c or slower than c, depending on how curved-or-flat the region they’re in and the region they’re observing are relative to one another. But is anything really moving faster or slower than c? No; what’s happening is we are often unable to measure what the speed of something at any location other than our own actually is. Einstein himself noted this in his 1920 book, Relativity: the special and general theory, where a translation (from German) reads:
according to the general theory of relativity, the law of the constancy of the velocity of light in vacuo, which constitutes one of the two fundamental assumptions in the special theory of relativity […] cannot claim any unlimited validity. A curvature of rays of light can only take place when the velocity of propagation of light varies with position.
Now, what about a passing gravitational wave? As it turns out, it will have an effect on all the space it passes through. Just as gravitational waves compress space in one direction while simultaneously stretching the perpendicular direction in an oscillatory fashion — a feature LIGO took advantage of to directly detect them — they will stretch and compress the event horizon of a black hole as well.
The waves that pass inside the black holes event horizon will, in fact, have their energy absorbed into the black hole itself; just as any light that fell in would add to the black hole’s mass (converting energy to mass via a less familiar form of Einstein’s famous equation, m = E/c2), so does gravitational radiation. But those that aren’t absorbed might distort the space around it, and the curved space itself — plus the changes in the curved space — would certainly affect the light-travel-time of anything surrounding it. Particles experience time differently in these gravitational fields; space looks longer-or-shorter depending on the physics of the waves passing through them; the shapes of both physical objects and non-physical geometric constructs indeed get distorted.
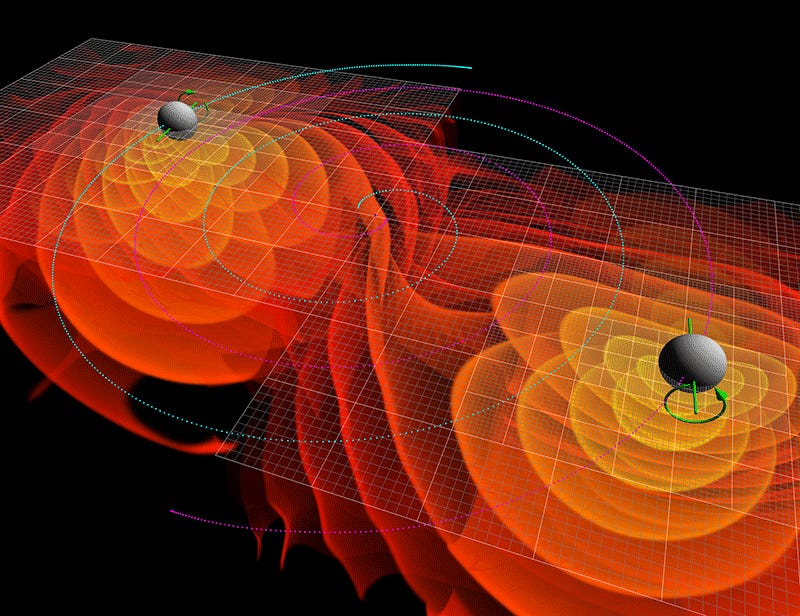
But at no point does this mean that the space that was inside the event horizon ever travel to the outside. At no point would a particle from the interior ever find its way to the exterior. And at no instant could anyone receive information outside of the black hole about what was going on in the interior. The escape velocity of something right at the edge of the event horizon would still be c, and what you would call a “change in the speed of light” as an external observer (incapable of measuring speeds at any location other than your own) is more accurately described by a distortion in the curvature of space itself.
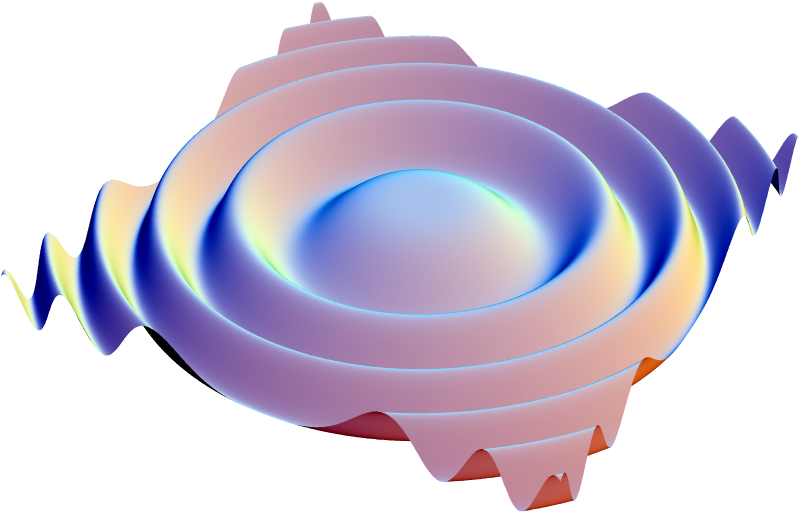
The space inside the event horizon might expand and/or contract as a gravitational wave passes through, but the best you could hope for is that a photon that otherwise would have fallen in might have a chance to not fall in. Gravitational waves don’t change the most fundamental, inescapable fact about black holes of all: nothing that’s already inside will ever get out.
This post first appeared at Forbes, and is brought to you ad-free by our Patreon supporters. Comment on our forum, & buy our first book: Beyond The Galaxy!