Ask Ethan #98: When Will The Stars Go Dark?
Even the dead stars still shine today, and will for a long time. But they, too, will fade to black.
“As the blackness of the night recedes so does the nadir of yesterday. The child I am forgets so quickly.” –Sylvia Ashton-Warner
Each and every week, you send in your questions and suggestions for Ask Ethan, and we set a new record with over 100 column ideas new for this week. There were a lot of great candidates, but the one I wound up choosing was one of the shortest and sweetest, yet one of the most profound, courtesy of a submitter who simply goes by Steve:
How long would it take for stars to cool down after they have exhausted their nuclear fuel? Will there be any ‘black’ dwarfs? Are there any today?
Let’s start by talking about the lives of stars, and take you through to the very, very end to explore this fully.
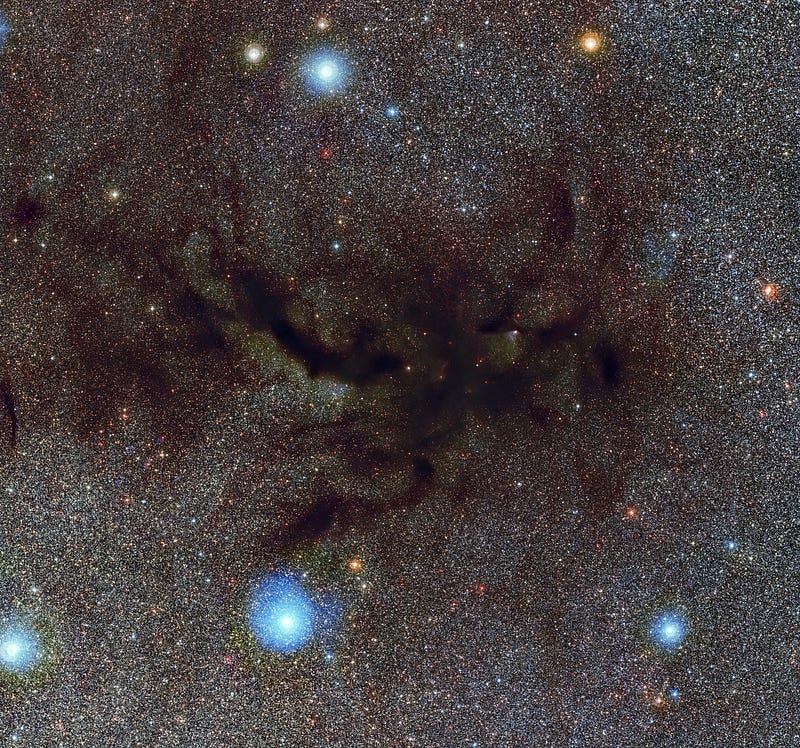
When a cloud of molecular gas collapses under its own gravity, there are always a few regions that start off just a little bit denser than others. Every location with matter in it does its best to attract more and more matter towards it, but these overdense regions attract matter more efficiently than all the others.
Because gravitational collapse is a runaway process, the more matter you attract to your vicinity, the faster additional matter accelerates to join you. While it can take millions to tens of millions of years for a molecular cloud to go from a large, diffuse state to a relatively collapsed one, the process of going from a collapsed state of dense gas to a new cluster of stars — where the densest regions ignite fusion in their cores — takes only a few hundred thousand years.

When you create a new cluster of stars, the easiest ones to notice are the brightest ones, which also happen to be the most massive. These are the brightest, bluest, hottest stars in existence, with up to hundreds of times the mass of our Sun and with millions of times the luminosity. But despite the fact that these are the stars that appear the most spectacular, these are also the rarest stars, making up far less than 1% of all the known, total stars, and also the shortest-lived stars, as they burn through all the nuclear fuel (in all the various stages) in their cores in as little as 1–2 million years.
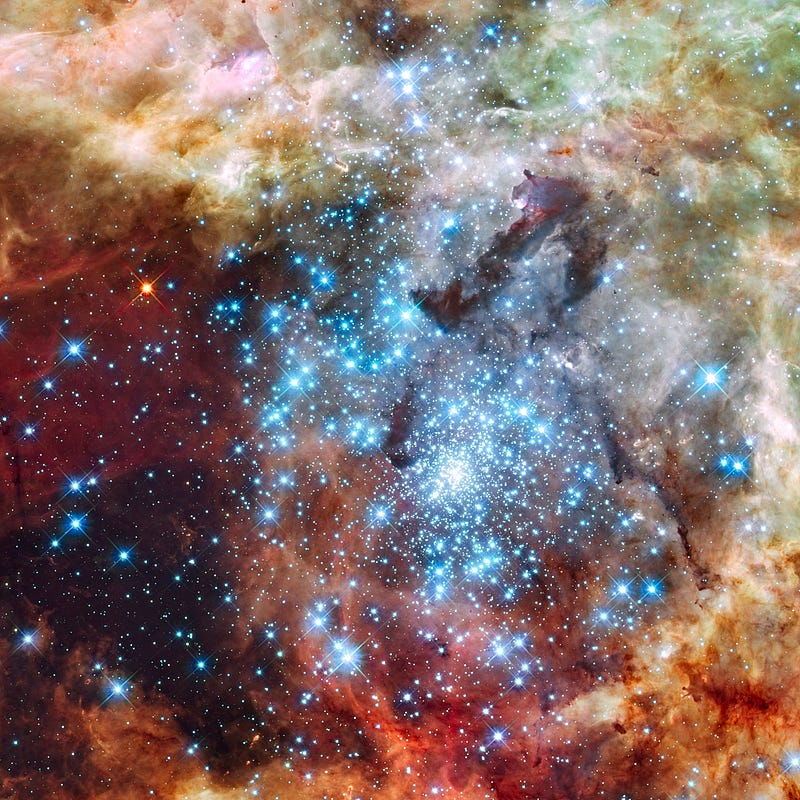
Acknowledgment: R. O’Connell (University of Virginia) and the Wide Field Camera 3 Science Oversight Committee.
When these brightest stars run out of fuel, they die in a spectacular type II supernova explosion. As this occurs, the inner core implodes, collapsing all the way down to a neutron star (for the low-mass cores) or even to a black hole (for the high-mass cores), while expelling the outer layers back into the interstellar medium. There, these enriches gases will contribute to future generations of stars, providing them with the heavy elements necessary to create rocky planets, organic molecules, and in rare, wonderful cases, life.
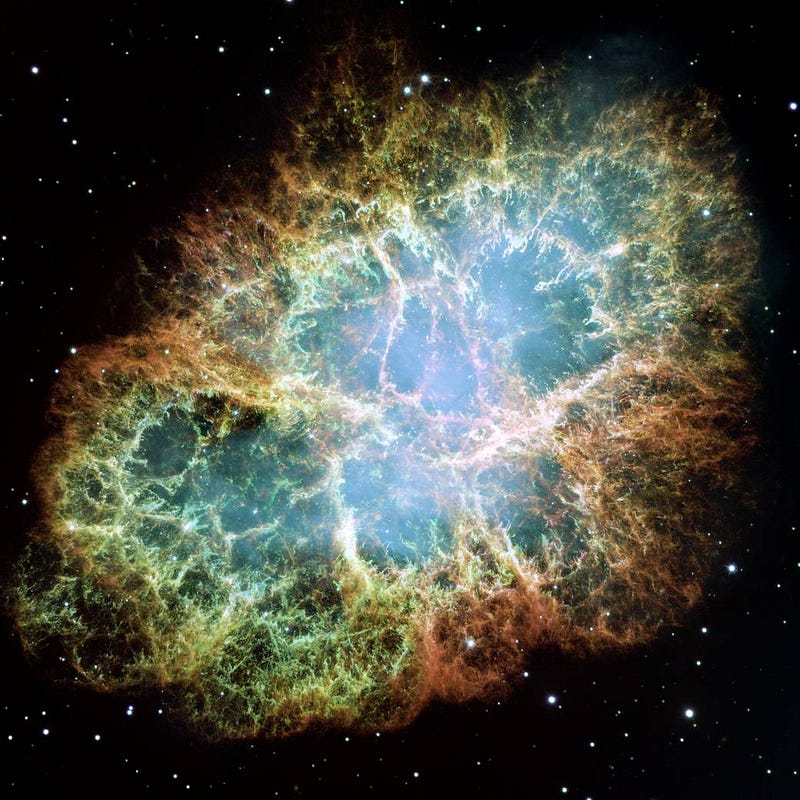
Black holes… well, by definition go black immediately. Other than the accretion disks surrounding them and the extraordinary low-temperature Hawking radiation emanating from their event horizons, black holes go black practically instantaneously following the core’s collapse.
But neutron stars are a different story.
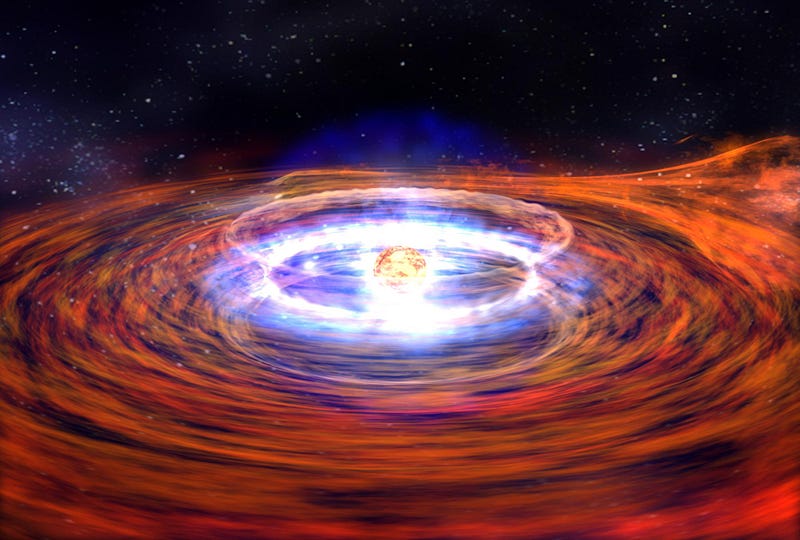
You see, a neutron star takes all the energy in a star’s core and collapses incredibly rapidly. When you take anything and compress it quickly, you cause the temperature within it to rise: this is how a piston works in a diesel engine. Well, collapsing from a stellar core all the way down to a neutron star is maybe the ultimate example of rapid compression. In the span of seconds-to-minutes, a core of iron, nickel, cobalt, silicon and sulphur many hundreds-of-thousands of miles (kilometers) in diameter has collapsed down to a ball just around 10 miles (16 km) in size or smaller. Its density has increased by around a factor of a quadrillion (10^15), and its temperature has grown tremendously: to some 10^12 K in the core and all the way up to around 10^6 K at the surface.
And herein lies the problem.
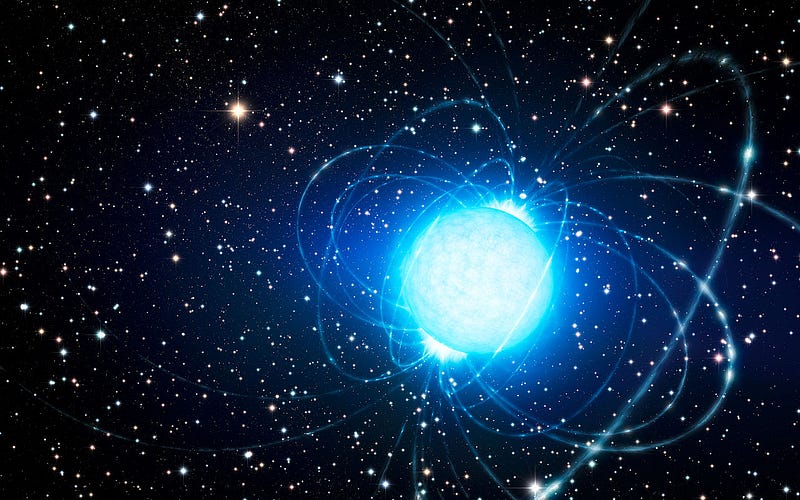
You have all this energy stored within a collapsed star like this, and its surface is so tremendously hot that it not only glows bluish-white in the visible portion of the spectrum, but most of the energy isn’t visible or even ultraviolet: it’s X-ray energy! There is an insanely large amount of energy stored within this object, but the only way it can release it out into the Universe is through its surface, and its surface area is very small.
The big question, of course, is how long will it take a neutron star to cool? The answer depends on a piece of physics that practically isn’t well-understood for neutron stars: neutrino cooling! You see, while photons (radiation) are soundly trapped by the normal, baryonic matter, neutrinos, when generated, can pass right through the entire neutron star unimpeded. On the fast end, neutron stars might cool down, out of the visible portion of the spectrum, after as little as 10^16 years, or “only” a million times the age of the Universe. But if things are slower, it might take 10^20-to-10^22 years, which means you’ll be waiting for some time.
But there are other stars that go black faster.
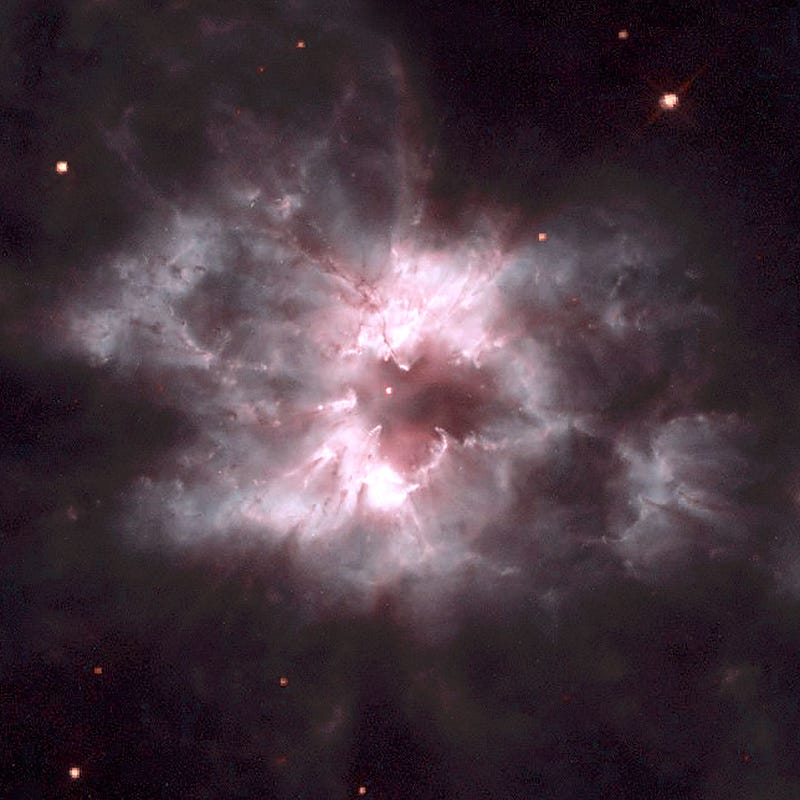
You see, the vast majority of stars — the other 99% and change — don’t go supernova, but rather, at the end of their lives, contract (slowly) down into a white dwarf star. The “slow” timescale is only slow compared to a supernova: it takes tens-to-hundreds of thousands of years rather than mere seconds-to-minutes, but that’s still fast enough to trap almost all the heat from the star’s core inside. The big difference is that instead of trapping it inside of a sphere with a diameter of only 10 miles or so, the heat is trapped in an object “only” about the size of Earth, or around a thousand times larger than a neutron star.
This means that while the temperatures of these white dwarfs can be very high — over 20,000 K, or more than three times hotter than our Sun — they cool down much faster than neutron stars.
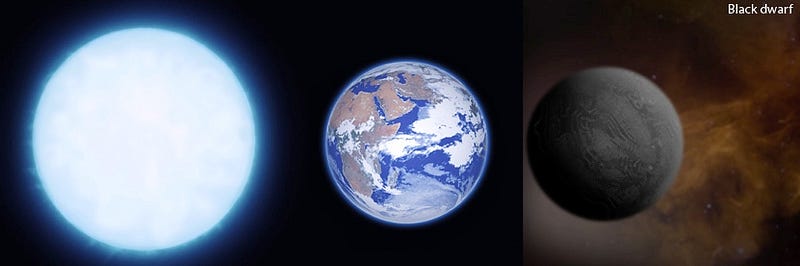
Neutrino escape is negligible in white dwarfs, meaning that radiation through the surface is the only effect that matters. When we calculate how quickly heat can escape by radiating away, it leads to a cooling timescale for a white dwarf (like the kind the Sun will produce) of around 10^14-to-10^15 years. And that’s to get all the way down to just a few degrees above absolute zero!
This means that after around 10 trillion years, or “only” around 1,000 times the present age of the Universe, the surface of a white dwarf will have dropped in temperature so that it’s out of the visible light regime. When this much time has passed, the Universe will possess a brand new type of object: a black dwarf star.
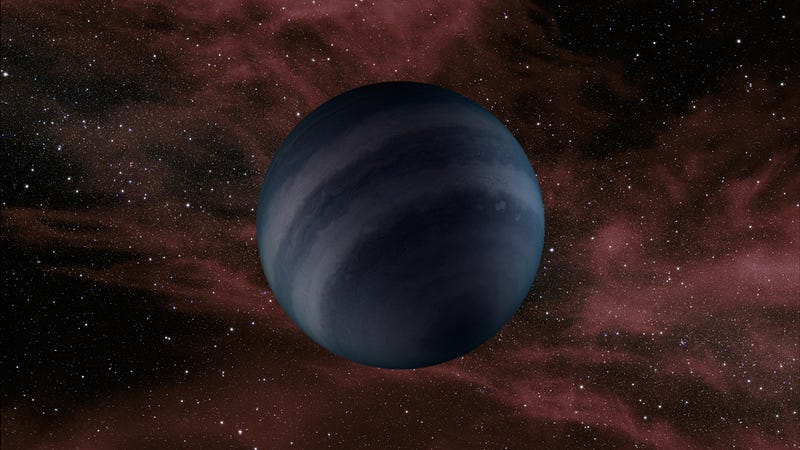
So no, Steve, I’m sorry to disappoint you, but there aren’t any black dwarfs around today. The Universe is simply far too young for it. In fact, the coolest white dwarfs have, to the best of our estimates, lost less than 0.2% of their total heat since the very first ones were created in this Universe. For a white dwarf created at 20,000 K, that means its temperature is still at least 19,960 K, telling us we’ve got a terribly long way to go, if we’re waiting for a true dark star.
It’s funny how we think of our Universe as littered with stars, clustered together into galaxies, separated by vast distances. By time the first black dwarf comes to be, our local group will have merged into a single galaxy (Milkdromeda), most of the stars that will ever live will have long since burned out, with the surviving ones being exclusively the lowest-mass, reddest and dimmest stars of all.
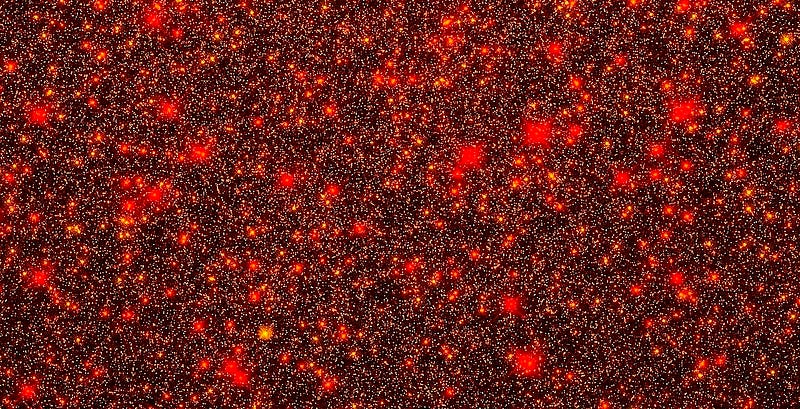
In addition, every other galaxy beyond our own will have forever disappeared from our reach, thanks to dark energy. The chances for life in the Universe will be at the very tail end, and stars (and stellar corpses) will begin to be thrown out of our galaxy due to gravitational interactions faster than new stars form.
And yet, amidst it all, a new type of object will come to be for the very first time. Even though we’ll never see or experience one, we know enough of nature to know not only that they’ll exist, but how and when they’ll come to be. And that, in itself, is one of the most amazing parts of science of all!
Have a question or suggestion for Ask Ethan? Submit it here for our consideration.
Leave your comments at our forum, and support Starts With A Bang on Patreon!