Ask Ethan #93: Newton’s Random Apple
If all the random motions of the molecules inside aligned, how far and fast would it go?
“Millions saw the apple fall, but Newton was the one who asked why.”
-Bernard Baruch
One of the great joys of being a scientist writing about what they love for the world is that every once in a while, you run across someone who’s been curious about a particular question for their entire lives, and has never gotten a satisfactory answer. If you’ve got that going for you, perhaps you’d like to submit your question or suggestion for Ask Ethan, and maybe you’ll get lucky just like Mike did, who asked:
This question has bothered me since I was a kid. If all the random molecular heat motion in an apple picked the same direction, how far would the apple go? And then what?
When you think about large objects down on a microscopic level, what is the picture in your head?
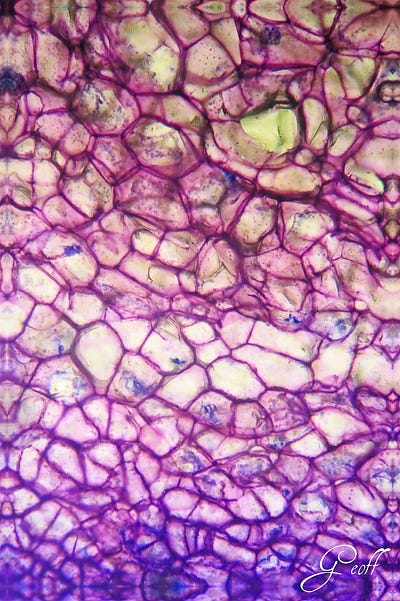
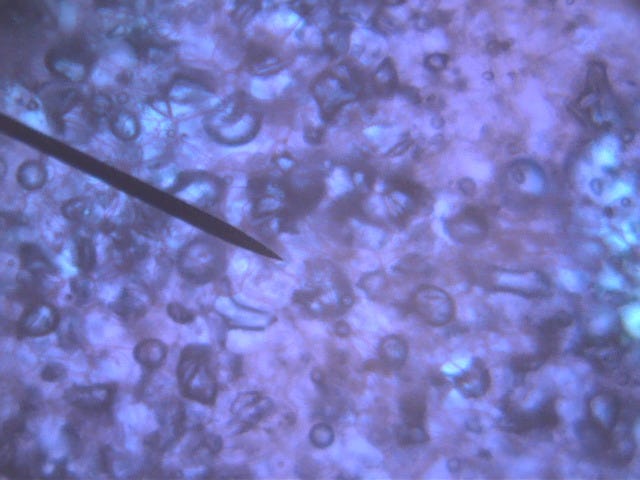
Perhaps you think down to the cellular level, magnified hundreds of times more powerfully than what we macroscopically can view. But we can go far, far deeper than this.
You see, each cell is made of organelles, each organelle has its own unique set of molecular configurations that give it a structure and function, and each molecule itself is made up of still smaller particles: atoms, electrons, nuclei and even smaller fundamental particles like quarks and gluons.
Perhaps you think down to the smallest constituents of matter when you think about apples, and how these particles must be bouncing around.

If this were an accurate picture of an apple, all you’d need to do to answer Mike’s question would be to measure the temperature of your apple — say room temperature, or ~298 K — figure out the masses of the particles bouncing around, like a sugar molecule of 342.3 amu, and use the math of kinetic molecular theory to figure out how fast these molecules were moving on average.
You’d get a big number: around 147 m/s, or 329 miles-per-hour (529 kilometers-per-hour). That’s about three times as fast as the apple launched from this apple gun, below.
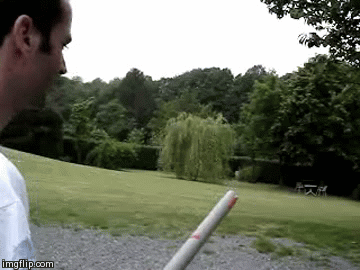
If you could somehow capture all the thermal/heat energy from the motions of these atoms within an apple and transfer it, 100% efficiently, into kinetic energy of the apple itself, this is exactly what you’d get.
But there are two problems with this line of reasoning, which is to say there are two really good reasons why your apple will never actually do this.
1.) We have the pesky law of momentum conservation. Thermal motions are random motions, which means that for every atom-or-molecule that moves in one direction, there’s another atom-or-molecule that moves with the same speed in the opposite direction. Sure, the individual components of your apple might be moving quickly, but overall, the net momentum of the apple is zero, just like the apple itself might be made out of some 10^27 protons and 10^27 electrons, but there aren’t tremendous electrical forces at play overall, since the total charge balances, and is zero. Similarly, you can’t take that random energy configuration and convert it into directional kinetic energy without in some way compensating, and having an equal-and-opposite amount of momentum moving in the opposite direction from the one you want to send the apple.
If that were the only constraint, however, there would be a very clever way around it.
You could send a very small amount of the apple’s mass flying in one direction by taking advantage of the properties of recoil: having a small mass bounce off a larger one, which bounces off a larger one, which in turn bounces off a larger one, and so on.
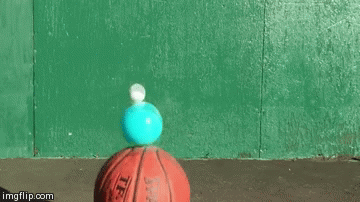
This actually comes into play in a hugely important way in nuclear physics, by a phenomenon known as the Mössbauer effect, which effectively immobilizes nuclei in a crystal, allowing for a tiny change in the momentum of the overall crystal to cause individual particles (or photons) to be emitted with tremendous energies/speeds. A reverse Mössbauer effect could enable the apple itself to fly off “slowly” (at 147 m/s) while only a tiny fraction of it headed off in the opposite direction with a tremendous amount of momentum.
But the second reason why this won’t happen is a real killer.
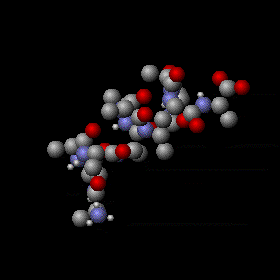
2.) These atoms aren’t actually free, but are bound in molecules, which are mostly bound together in a large-scale, solid structure. The picture we put together earlier — of atoms bouncing around off of one another — is pretty good for liquids and even better for gases and plasmas. But for solids? We can’t apply the same physics at all. We get vibrational and/or rotational motion, but not free, rapidly-moving kinetic motions like we talked about earlier.
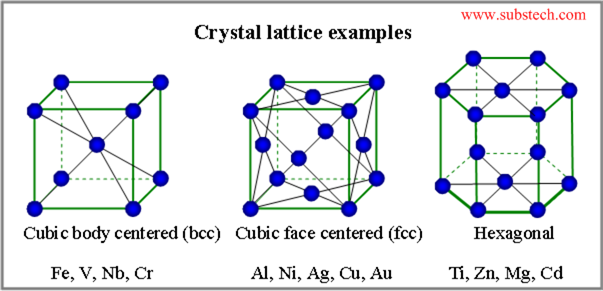
There’s a large amount of energy stored in the bonds of a solid object, but the thermal energy present that causes these atoms to vibrate is insufficient to break these bonds, and hence the apple remains bound in its solid state.
It takes an incredible amount of thermal energy to break those bonds, something that you’re not really going to be able to do unless you dehydrate the apple, since anything above about 373 K is going to boil off the water inside the apple itself.
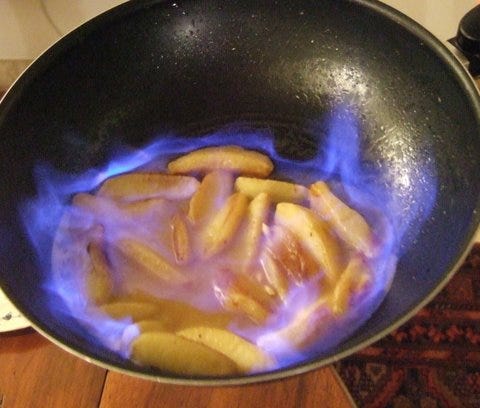
If we force ourselves to recognize the fact that we don’t have individual, free water molecules, sugar molecules, and other small molecules in an apple, but rather very large, massive structures (like cells) instead, we find that the individual “random motions” are much, much smaller than we had previously considered. Even if we pretended (and this is a great exaggeration) that the apple was divided into nanogram-mass particles that were unbound and free to move around, we’d find that the thermal motions were minuscule: at speeds of about 100 microns-per-second.
In other words, because your apple is a solid, and the molecules within are bound together, these thermal motions aren’t going to enable you to reach a very substantial speed at all. Even if you’re willing to contrive the configuration you’re seeking, the fact of the matter is — at the end of the day — you’ve simply got a somewhat warm apple that isn’t going anywhere.
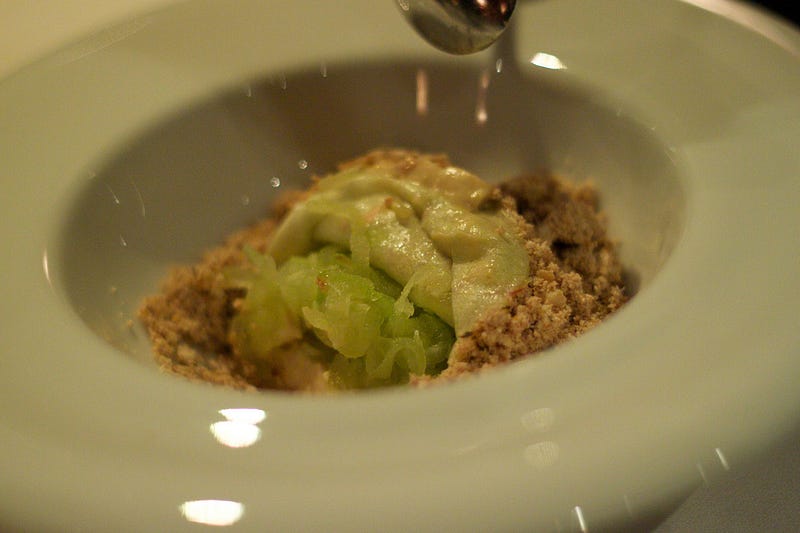
But even though it might not be the answer you wanted, considering the laws of physics allows us to explore the nature of matter and learn a little more about how our Universe works. For me, that’s what Ask Ethan is all about! So if you’ve got a question or suggestion to send in, let me have it. The next column could be all yours.
Leave your comments at our forum, and support Starts With A Bang on Patreon!