Ask Ethan #71: Heavy planets, light Sun?
The Sun is made almost entirely out of hydrogen and helium; the Earth has hardly any of either. How’d that happen?
“The single most powerful element of youth is our inability to know what’s impossible.” –Adam Braun
Each week, you send in your questions and suggestions, and I pick my favorite to tackle in our weekly Ask Ethan column. But sometimes, the simplest questions are the most challenging to answer. Take a look, for example, and the Sun and the stars, and then take a look at the planets. You might think that mass is the only difference — that if you made a planet massive enough, it would become a star — but then how do you explain the simple observation that Greg Rogers makes:
If the Sun (and all stars) are mostly Hydrogen and Helium, why don’t planets have about the same distribution of stuff?
Not only don’t planets have about the same distribution of stuff, it’s not even close.
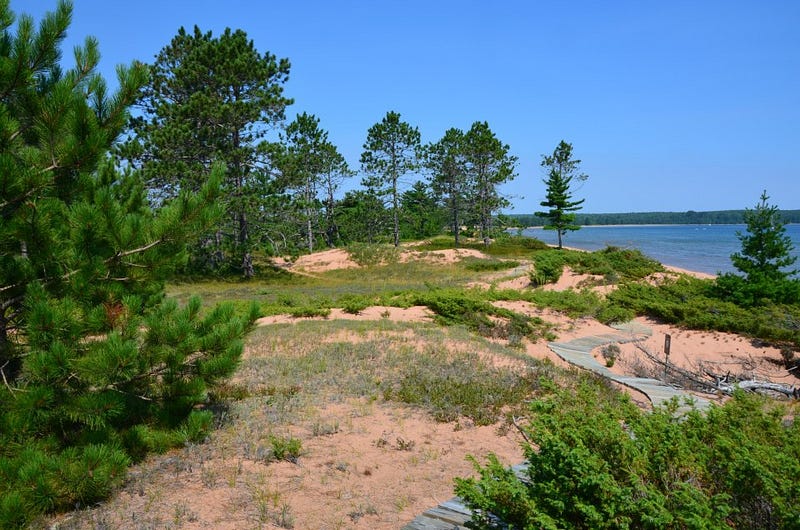
If we take a look around at say, the surface of our planet, we find that there are all sorts of elements around: some 90-or-so naturally occurring ones on our surface. Hydrogen is plentiful, but not dominant, especially not if we’re looking by mass. The air we breathe is mostly nitrogen and oxygen; the oceans that cover our world are only about 11% hydrogen by mass (because each oxygen atom is 16 times as massive as each hydrogen); the solid matter of both living and non-living things from rocks to dirt to plants and animals certainly contains hydrogen in significant amounts, but it’s vastly outnumbered (and outmassed) by things like sodium, oxygen, silicon, aluminum and a whole slew of other elements.
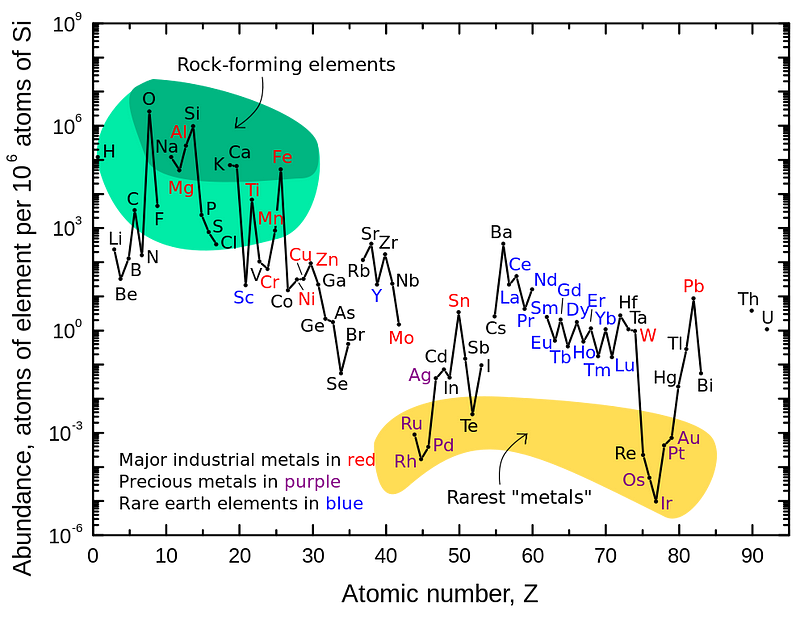
If we take a dive inside our planet, the situation gets even worse. Sure, we can find additional repositories of helium stored in underground chambers, but those were produced by radioactive decays of ultra-heavy elements over billions of years. There are small amounts of hydrogen in there as well, by far and away we come to heavier and heavier elements: metals like iron, nickel and cobalt, as well as elements that transcend the limit of stability on the periodic table.
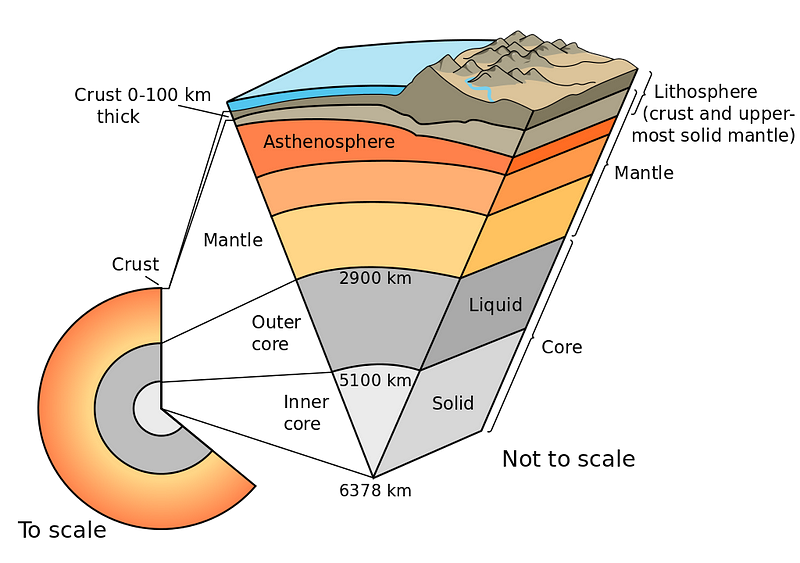
We know this because the different layers of Earth get denser and denser as we go deeper and deeper. It isn’t exclusively due to gravitational contraction and compression, either; the heavier elements sink to the bottom.
This last point is incredibly important so I’ll say it again: when the Earth is very young, a huge variety of elements are present, but the heavier elements sink to the bottom and the lighter elements “float” on the top, the same way that less dense liquids will float atop denser ones.
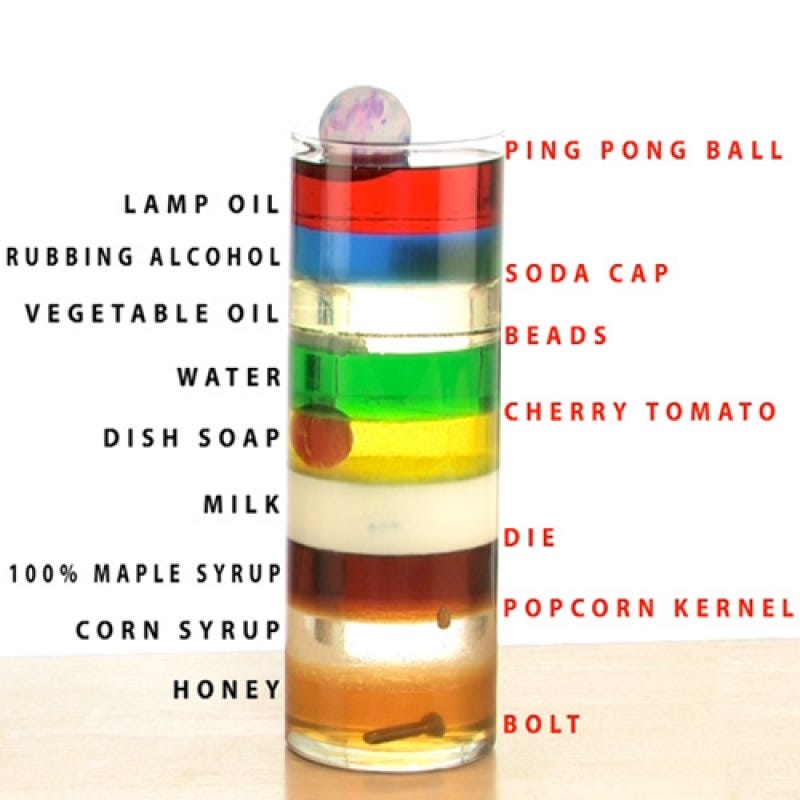
So when we’re looking at the Earth, we’re actually seeing the lightest elements that our planet is made of preferentially represented on the surface; the majority of what we have is even heavier and denser. So when it comes to hydrogen and helium, we truly have very little of it.
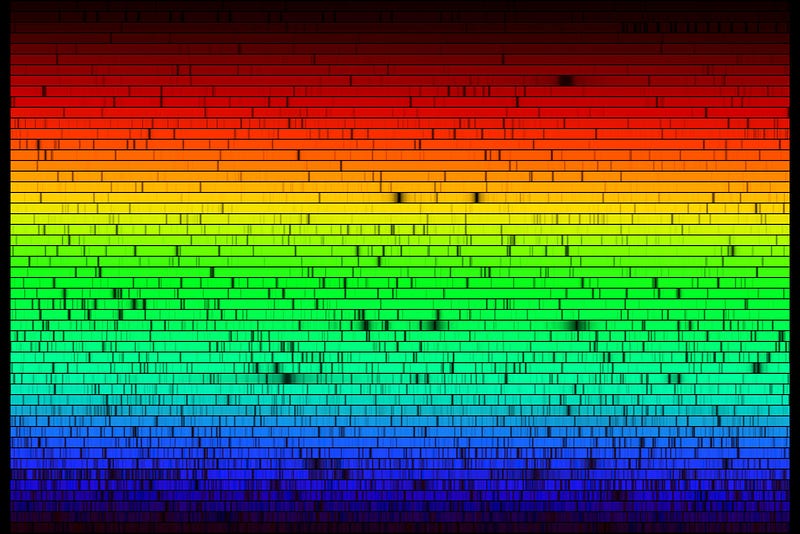
And then, we come to the Sun and the stars. Have a look at the solar spectrum: you can see that there are all sorts of absorption lines there, representing the full gamut of elements found on Earth, as well as a few that aren’t naturally occurring here at all.
But one thing really stands out: two sets of absorption features — those from hydrogen and helium — are incredibly strong. Once we came to understand how stars worked, and how temperature, ionization and elemental abundance were related, we discovered that the Sun was made of roughly 70% hydrogen, 28% helium and only about 1-to-2% “other” stuff.
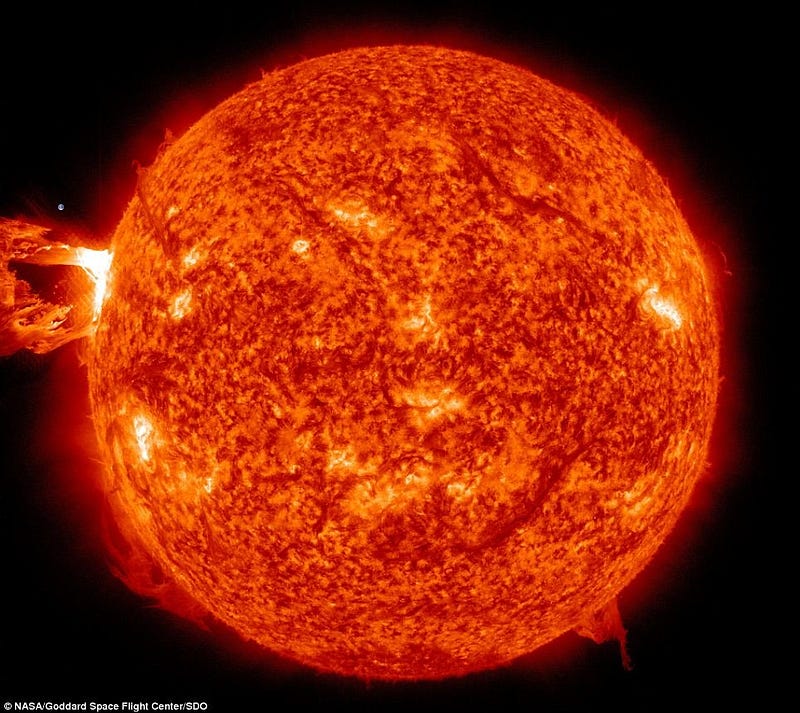
Yet the Earth is 99%+ “other stuff”! So why is this the case? To understand it, let’s go all the way back to our birthing grounds: the star forming nebulae. These are molecular clouds of gas — mostly hydrogen, lots of helium, and small amounts of other stuff — that have begun to collapse under their own gravity.
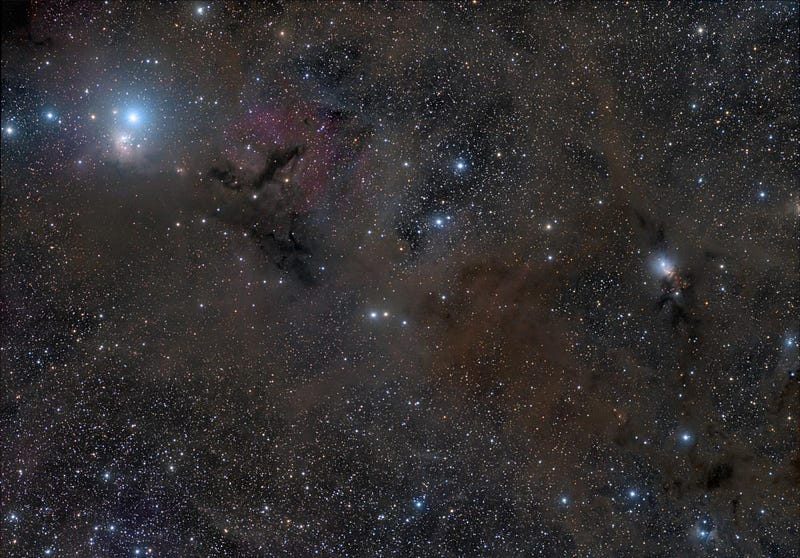
In the earliest stages that lead to star formation, gravitation is the only thing that matters. The gas cloud inevitably develops clumps, and these clumps grow denser and denser in places, with these overdensities attracting more and more matter to them. Because gravitational collapse is relatively rapid and there’s not a very efficient way for these gas clouds to radiate their energy away, the collapse causes the interior of these clumps to heat up. Before long, the hydrogen at the core has reached sufficient temperatures and densities to begin nuclear fusion.
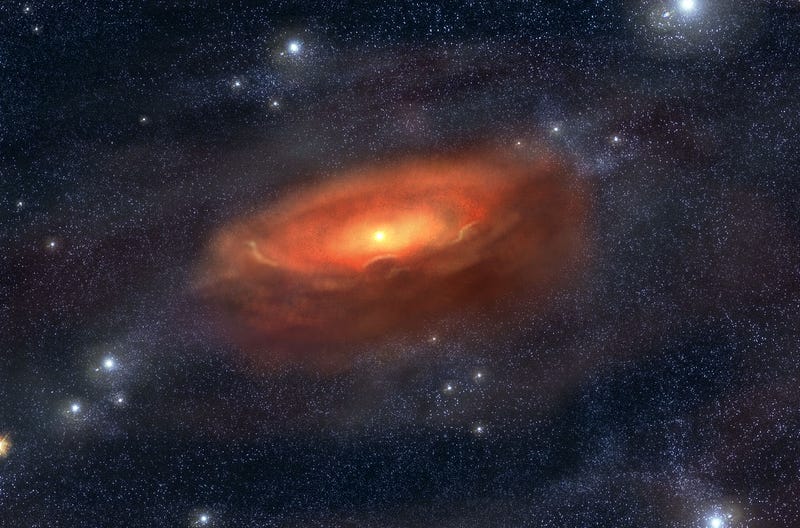
These infant stars come in many varieties: different colors, temperatures and masses. But one thing that most of them have in common is that they don’t form in isolation, but rather with other, smaller “clumps” of matter around them. The largest ones — and the ones that got the biggest head starts — will eventually grow into rocky planets, gas giants, or in the most extreme cases, other stars.
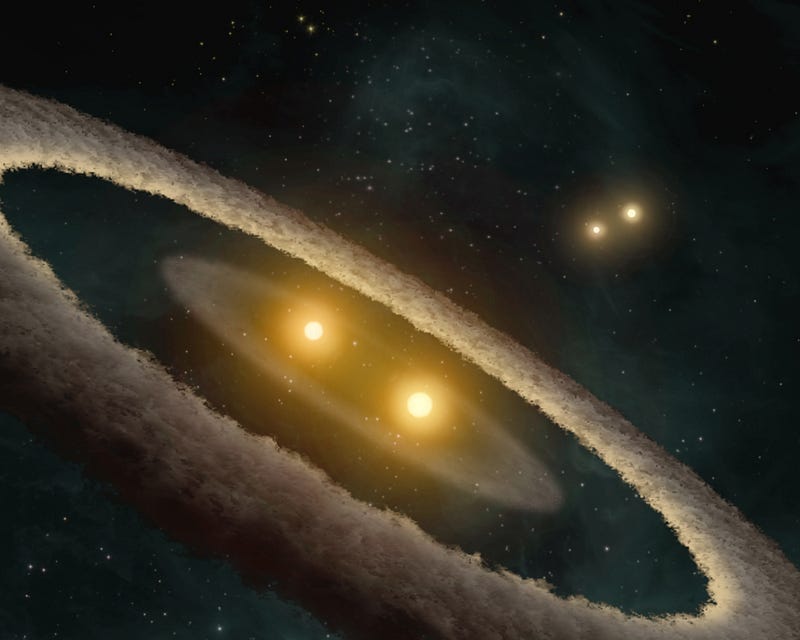
At the same time, the energy being put out by the parent star in the system is being flung outwards at everything it can interact with in the solar system. This includes the solar wind, ions, electrons and — of course — photons. The thing is, what are these energetic particles going to be running into?
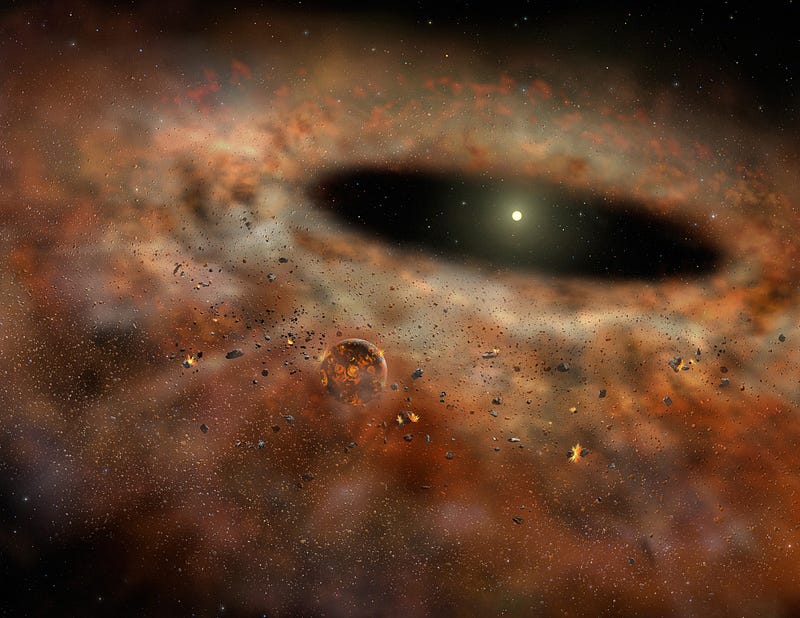
For every planet or planetoid they encounter, they’re running into the outermost, lightest elements, because those are the ones that “float” on top of the heaviest ones, which have mostly sunk towards the center. Think about what happens if you run up to and kick a soccer ball as hard as you can, versus what happens if you kick a bowling ball as hard as you can. Don’t think about your foot: think about the ball! The soccer ball will get an incredible velocity imparted to it, and will likely fly fast and far, while the bowling ball will hardly go anywhere at all.
Why? Because when you give things with different masses the same energetic kick, the lighter ones wind up moving faster.
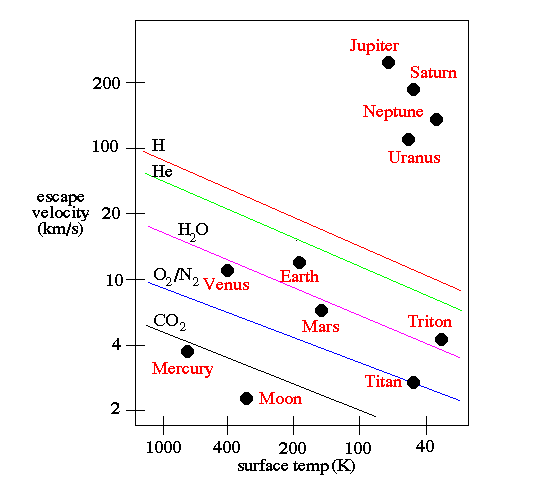
This is enough — on nearly all worlds — to expel practically all of the hydrogen and helium into interstellar space: the energy emitted from the star is enough to give those atoms sufficient speed so that they reach escape velocity, and are no longer gravitationally bound to the world they began bound to.
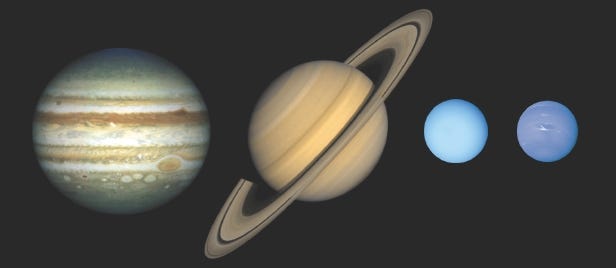
It’s only the gas giant worlds — worlds about two times the mass of Earth or more — that have enough gravity to hang on to a hydrogen/helium envelope. And the more massive your world is, the “thicker” the envelope it can hang on to! The gas giants are expected to have a dense, heavy-element filled solid core, but you’d only find it after descending through many layers that are dominated by hydrogen.
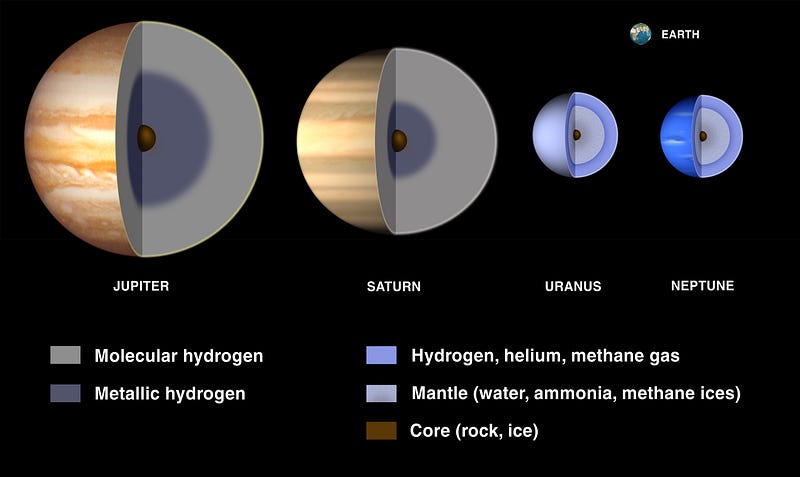
So to answer your question, Greg, planets are all born out of the same materials, and if it weren’t for the radiation emitted by stars, every single planet would be dominated by hydrogen and helium, just like our Sun and the stars. But being so close to an energetic source means that every element gets an energetic “kick” applied to it, and in the case of all the rocky planets we know of, that kick is enough to rid the world of practically all the free hydrogen and helium in it. It’s only when you accrue enough mass — and/or are also far enough away from your parent star — that you can begin to hang on to the lightest of all the elements in the face of all that incoming radiation. And then the more massive you are, the more you can hang onto! This goes all the way up to a limit of about 8% the mass of the Sun, where once you reach that, you begin fusing hydrogen into helium, and become a star yourself!
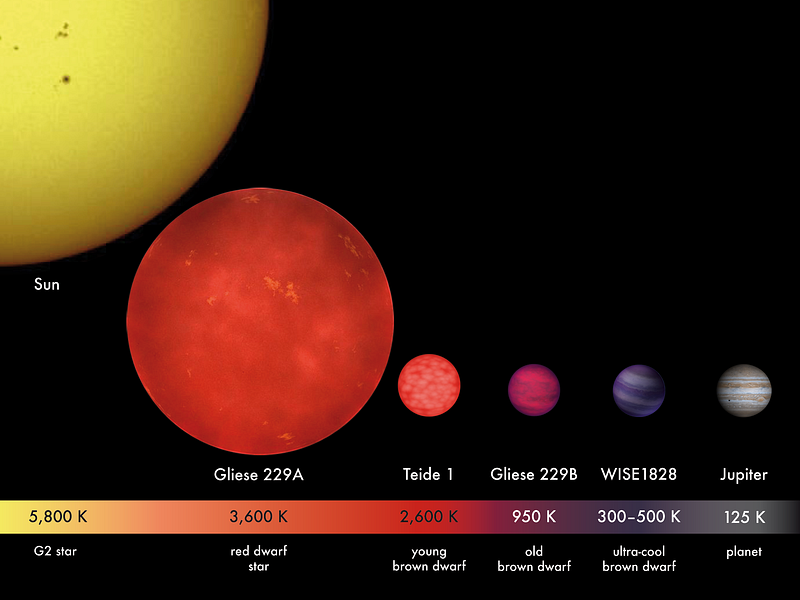
And that’s why the elements are where they are! Thanks for a great question, Greg, and if you’ve got questions or suggestions for the next Ask Ethan column, send them in. You might be surprised at what we know!
Leave your comments at the Starts With A Bang forum on Scienceblogs!