Ask Ethan #53: What is the Big Rip?
The scariest of all possible fates results in the ultimate destruction of everything that ever was or will be.
Image credit: Boren-Simon 2.8–8 ED POWERNEWT Astrograph Image Gallery.
“Why do people have to be this lonely? What’s the point of it all? Millions of people in this world, all of them yearning, looking to others to satisfy them, yet isolating themselves. Why? Was the earth put here just to nourish human loneliness?” –Haruki Murakami
There’s a vast Universe out there, stretching for hundreds of billions of light-years and containing close to a trillion galaxies at minimum. In truth, the Universe — going well beyond the part that’s observable to us — may well be infinite. But what’s going to happen to it all in the future? You’ve sent in a slew of great questions and suggestions this week, but this edition of Ask Ethan has the honor go to Jeff Harris, who asks:
A long time ago I read a New York Times article on something they called the Big Rip. They pointed out that an accelerating expansion of the universe would eventually lead to the galaxies being unperceivable to each other, then the stars being unperceivable to each other, and then atoms would be unable to form, and matter would ‘evaporate’. Given current estimates of the acceleration rate, is any of this possible? What would be the major observable benchmarks of this process of the ‘Big Rip’, and how far in the future from our current temporal origin point would each of these observable effects take place?
If you’re interested in the fate of the Universe, you’ll want to go back to the Big Bang and look at how the Universe has come forward to where we are today.
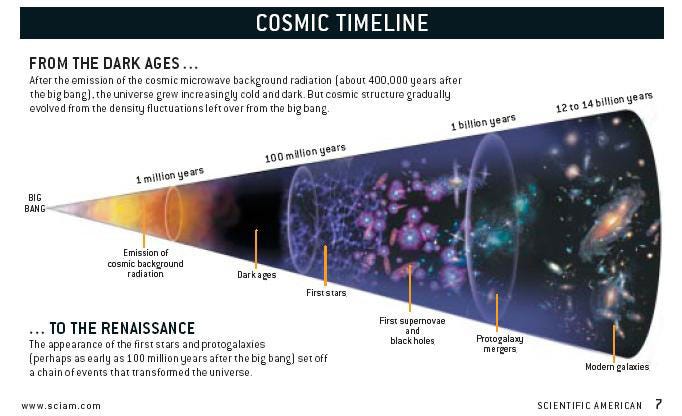
Back in the early stages, some 13.8 billion years ago, our Universe was hot, dense, almost perfectly uniform, and expanding very, very rapidly. At the same time, because the Universe is so massive, we’ve got the force of gravitational attraction working to slow that expansion down, counteract it, and — if possible — eventually reverse it. Whether it can or not should depend only on three things: the initial expansion rate, the total amount of matter-and-energy in the Universe, and what types of energy are present (and in what ratios) in our Universe.
For a long time, we thought there would be three possible fates to the Universe:
- A fate where there was enough matter-and-energy to overcome the initial expansion, slowing it, causing it to pause at some maximum scale, and reverse. The Universe would recollapse and end in a Big Crunch.
- A fate where there wasn’t enough matter-and-energy, and where the expansion continued forever into the future. Gravity would be sufficient to slow this rate continuously, but it would always remain positive, and distant galaxies would continue to get farther and farther apart forever.
- The “just right” case balanced in between the two above, where one more proton in the Universe would cause it to recollapse, but we simply don’t have it. In this case, the Universe’s expansion rate asymptotes to zero, but never reverses.
Of course, the actual Universe does none of these three things.
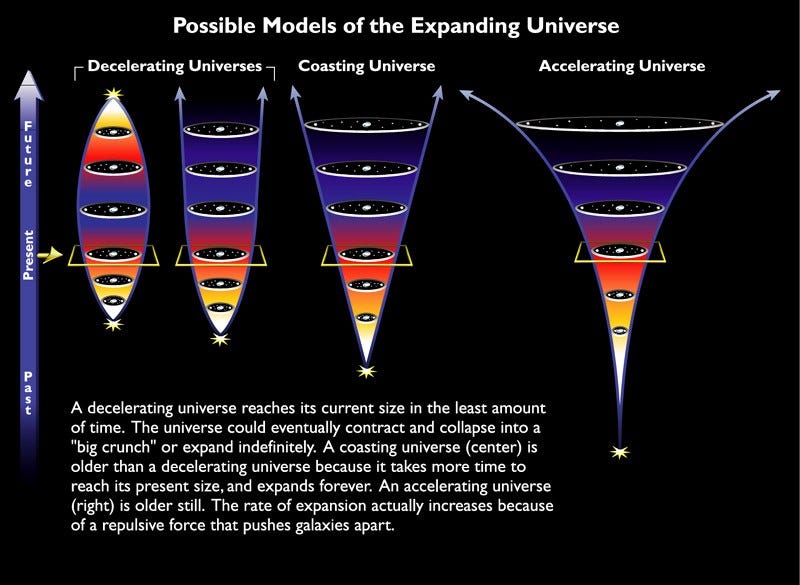
It expanded and slowed for a long time, as matter-and-radiation diluted, and then — about six billion years ago — the rate at which distant galaxies were receding from us stopped decreasing, and the Universe started accelerating. This acceleration has continued to the present day, and shows no sign of letting up.
Even as the matter and radiation densities continue to drop, the continued acceleration tells us that there’s a new form of energy in addition to these more common types: something we call dark energy.
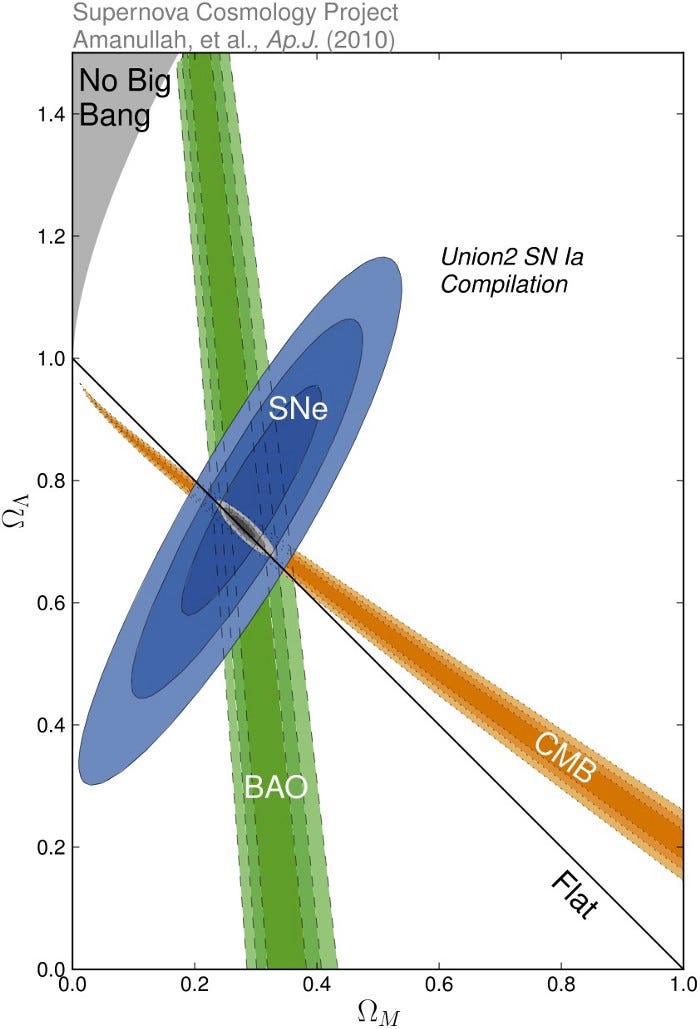
Dark energy shows itself in a number of different observations, including large-scale structure, the cosmic microwave background, and in observations of very distant objects, such as gamma-ray bursts, quasars and type Ia supernovae. We’ve measured it very precisely in recent years, and it’s gone just in the past decade from having uncertainties of around 100% to 50% to 30% to 12% and finally now down to about 8%.
To the best of our present limitations, we see that dark energy is consistent with being a cosmological constant, meaning that its energy density remains constant throughout time.
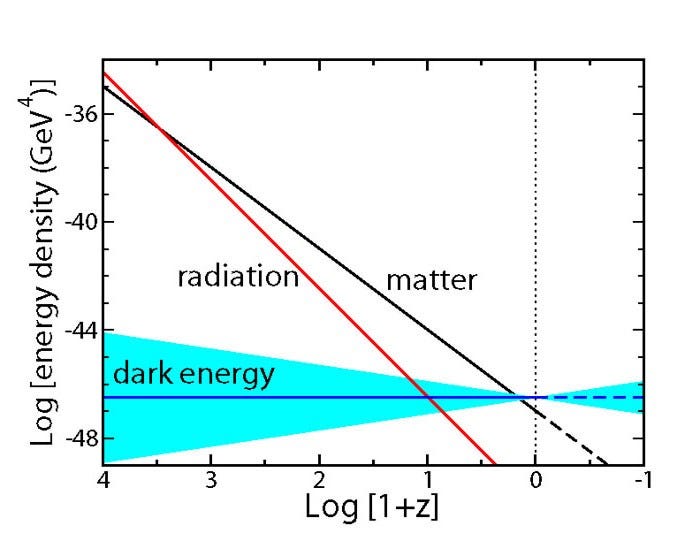
It doesn’t have to be exactly a constant. Theoretically, the best and most compelling argument (i.e., with the fewest assumptions and fewest free parameters) would be for dark energy to be a cosmological constant, and that’s what the data favors. Of all the possibilities, a cosmological constant would be the least surprising outcome.
But there are other possibilities: dark energy could be almost a constant, having decayed from something larger in the past and still decaying further today, albeit slowly. If this were the case, the acceleration phenomenon would eventually decay away, too, leaving us with a Universe that stopped expanding entirely.
It’s also conceivable that dark energy could reverse itself, going from a positive cosmological constant to a negative one, resulting in a Big Crunch after all.
But there’s one more possibility to consider — and this is what Jeff wants us to consider — that dark energy will actually get stronger as time goes on. This is the possibility that results in the Big Rip.
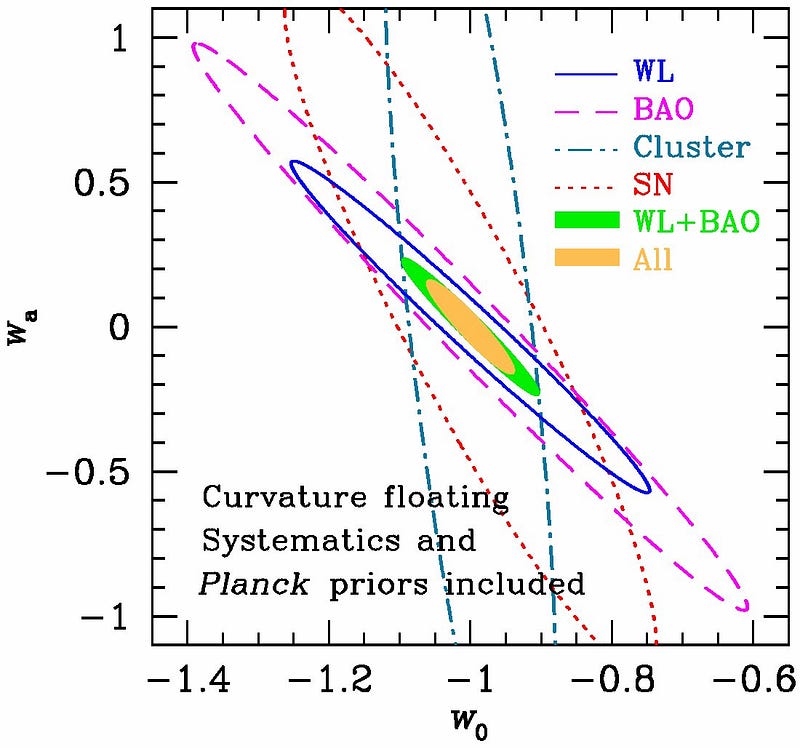
If dark energy were a constant, it would mean that an object that’s about 10 million light-years distant from us now should be receding from us at a rate of around 150-to-200 km/s. By time it’s 20 million light-years distant, however, it’ll be moving at 300 km/s. By time it’s 100 million light-years distant, it’ll be receding at 1,500 km/s, by time it’s a billion light-years away, 15,000 km/s and at 20 billion light-years distance, it’ll appear to recede from us at the speed of light, or 300,000 km/s!
The fact that our Universe is already accelerating and that there are objects receding faster than 300,000 km/s from us right now means that 97% of the Universe observable to us — all the stars, galaxies and planets more distant than that recession speed — are forever inaccessible to us. Even if we got into a spaceship today with an infinite amount of power at our disposal, we could never reach these distant destinations.
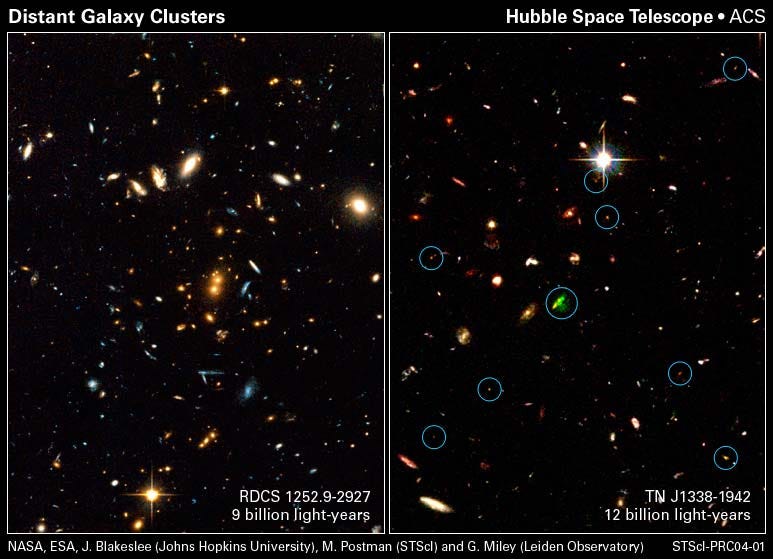
If dark energy is only a constant, than things like our Solar System, our galaxy, and even our local group of galaxies — consisting of the Milky Way, Andromeda, the Triangulum Galaxy, the Magellanic Clouds and a few dozen small, dwarf galaxies — will remain gravitationally bound together for trillions upon trillions of years into the future. But if dark energy is increasing, or getting stronger over time, then that acceleration rate will not only drive distant galaxies away from us, but will cause these structures to become gravitationally unbound as time goes on!
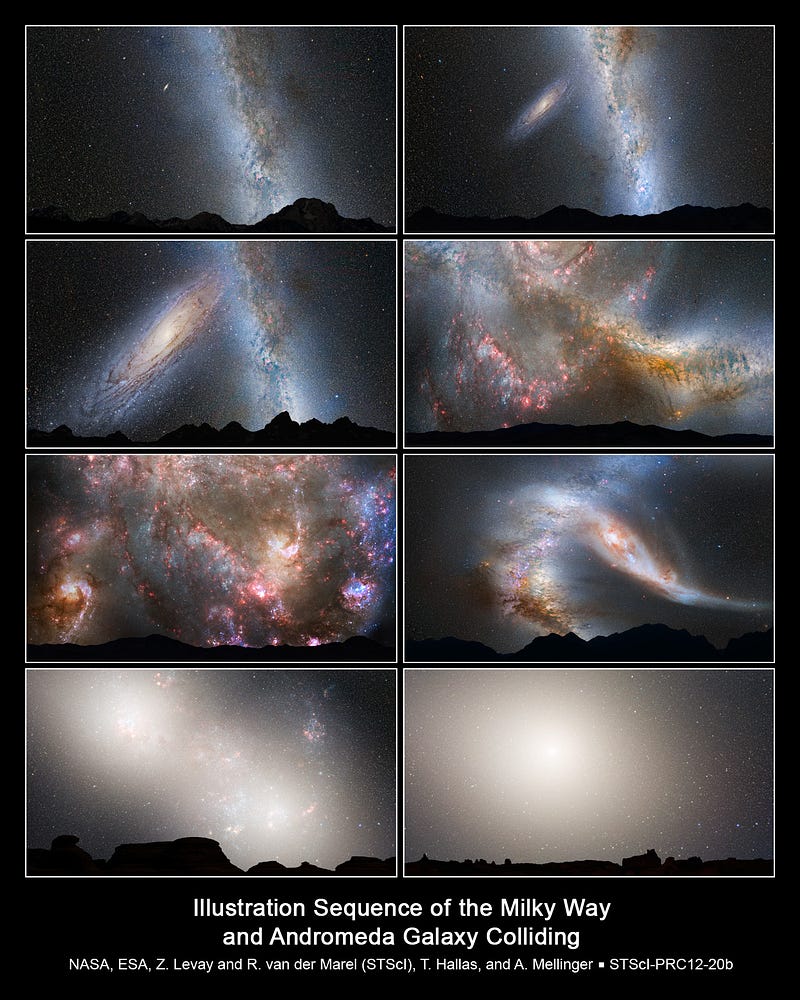
If the energy density of dark energy increased to about ten times what it is today, it would be enough to prevent the Milky Way from merging with Andromeda, and would instead drive our neighboring galaxy away from us, like all the other distant galaxies in the Universe. Also gone would be the Triangulum Galaxy and most of the other ones as well.
Increase the energy density of dark energy to about a hundred times its current value, and the stars on the Milky Way’s outskirts would begin flying off from our galaxy, as the metric expansion of space would even overcome the gravitational pull of all the matter in our local neighborhood. Get up to two or three hundred times its present value, and our Sun will join those outer stars in being torn apart from our galaxy.
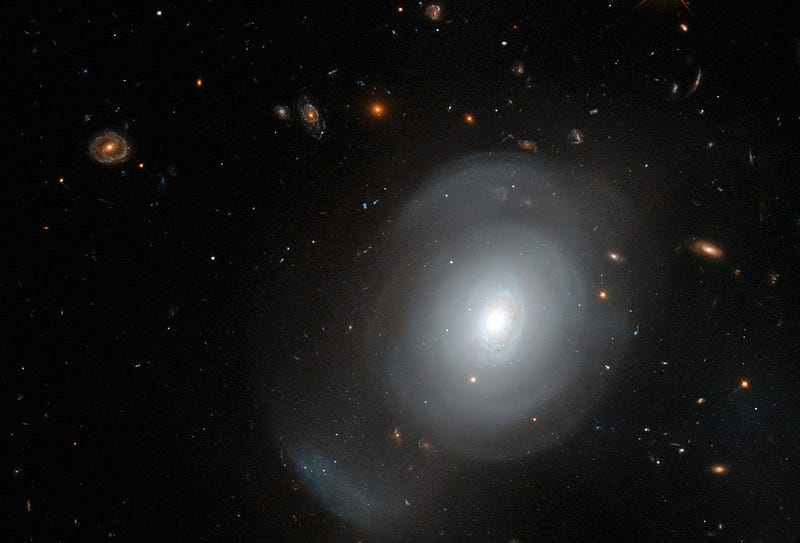
And if the energy density of dark energy continued to rise, what would happen to our Solar System? Eventually, the planets themselves would become unbound from our Sun, with Earth getting thrown out of orbit when dark energy reaches a density — are you ready? — of 100 billion times its present value. Finally, humans would be separated from Earth’s gravitational pull, individual cells, molecules, atoms, and nuclei would be torn apart, as the dark energy density continued to increase to an infinite amount. Presumably, even spacetime itself would be torn apart at the very end.
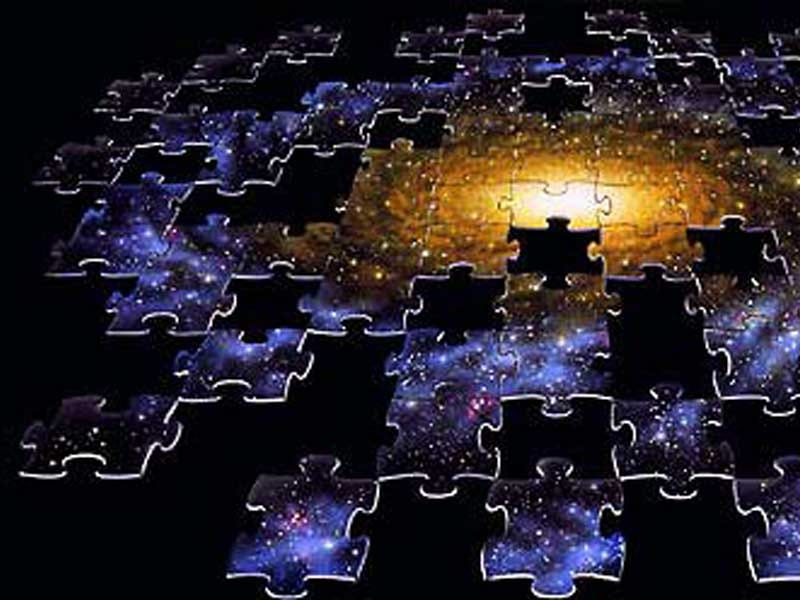
A horrifying fate, no doubt. This was first put forth in a paper in 2003 by Robert Caldwell, Marc Kamionkowski and Nevin Weinberg, and the way it worked was very simple. You see, all forms of energy density in the Universe have a pressure associated with them, and that pressure (with some unit conversions) can be expressed as a fraction of the energy density. Motionless dust has a pressure of zero, radiation has a pressure that’s 1/3 its energy density, and cosmological constants have a pressure that’s equal to the negative of the energy density.
In physics, we call that constant that goes out in front — +1/3 for radiation, 0 for matter, -1 for cosmological constant — the parameter w, and refer to it as an equation of state. The people who coined the term “Big Rip” originally considered w = -1.5, and found that the Universe would end in 22 billion years in that scenario. Almost every event outlined above occurs very close to the end, as the dark energy density would have to increase very slowly for a long time, only spiking towards infinity at the very end-of-the-Universe.
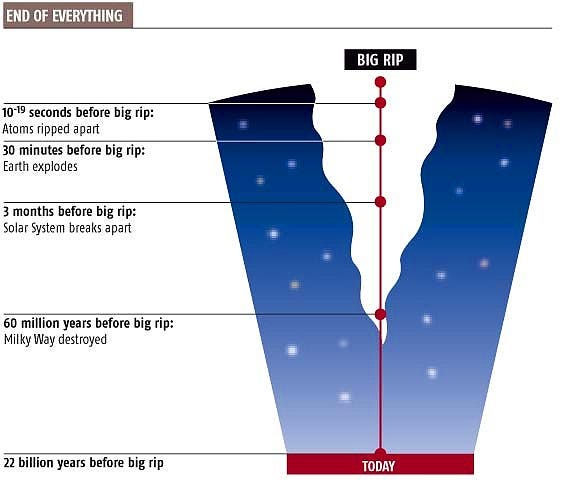
To the best of our measurements, we can now state that w = -1.0, with an uncertainty of about ±0.08, pushing any “Big Rip” scenarios out to at least 80 billion years from the present. If you want to calculate the lifetime left in the Universe for any equation of state, you can put in the following formula, and make sure you convert your units appropriately:

Although do be warned that, to the best of our knowledge, w = -1, exactly, and if that turns out to be the case, there will be no Big Rip ever. And that’s where I’d place my money if I were a betting man, although it’s important to remember that this is science, and we do not rule out any possibilities, no matter how distateful we may find them, until the evidence allows us to do so.
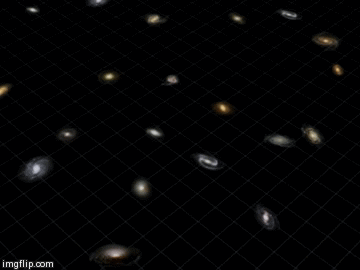
Thanks for a great question, Jeff, and if you want your question featured on Ask Ethan, send in your questions and suggestions. After all, the Universe is certainly full of puzzles great and small worth thinking about!
Leave your comments at the Starts With A Bang forum on Scienceblogs!