Antimatter Mystery Likely Due To Pulsars, Not Dark Matter
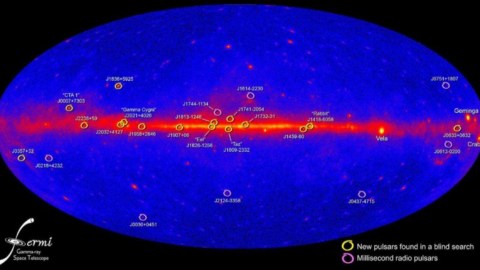
For years, astronomers have been puzzled by an excess of antimatter particles. Unfortunately, dark matter is probably not the solution.
When you look out at the Universe, what you see is only a tiny portion of what’s actually out there. If you examine the Universe solely with what’s perceptible to your eyes, you’ll miss out on a whole slew of information that exists in wavelengths of light that are invisible to us. From the highest-energy gamma rays to the lowest-energy radio waves, the electromagnetic spectrum is enormous, with visible light representing just a tiny sliver of what’s out there.
However, there’s an entirely different method to measure the Universe: to collect actual particles and antiparticles, a science known as cosmic ray astronomy. For more than a decade, astronomers have seen a signal of cosmic ray positrons — the antimatter counterpart of the electron — that they’ve struggled to explain. Could it be humanity’s best clue towards solving the dark matter mystery? A new study says no, it’s probably just pulsars. Here’s why.
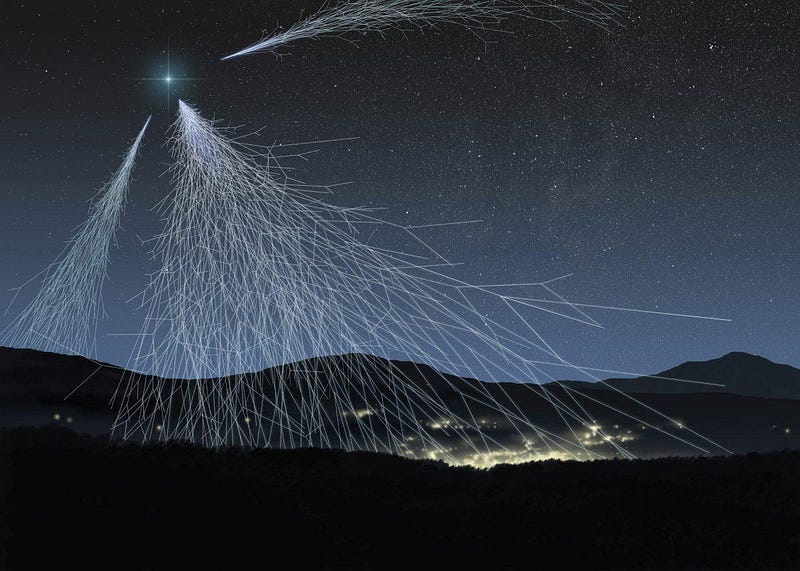
Cosmic rays produced by high-energy astrophysics sources can reach any object in the Solar System, and appear to permeate our local region of space omnidirectionally. When they collide with Earth, they strike atoms in the atmosphere, creating particle and radiation showers at the surface, while direct detectors in space, above the atmosphere, can measure the original particles directly. (ASPERA COLLABORATION / ASTROPARTICLE ERANET)
There are a great many things in the Universe that are known to create positrons, the antimatter counterpart of electrons. Whenever you have a high-enough energy collision between two particles, there’s a certain amount of energy that will be available with the potential to create new particle-antiparticle pairs. If that available energy is greater than the equivalent mass of the new particle(s) you want to create, as defined by Einstein’s E = mc2, there’s a finite probability of generating those new particles.
There are all sorts of high-energy processes that can lead to this type of energy becoming available, including particles accelerated by black holes, high-energy protons colliding with the galactic disk, or particles accelerated in the vicinity of neutron stars. Based on the known physics and astrophysics of the Universe, we know that a certain amount of positrons must be generated irrespective of any new physics.
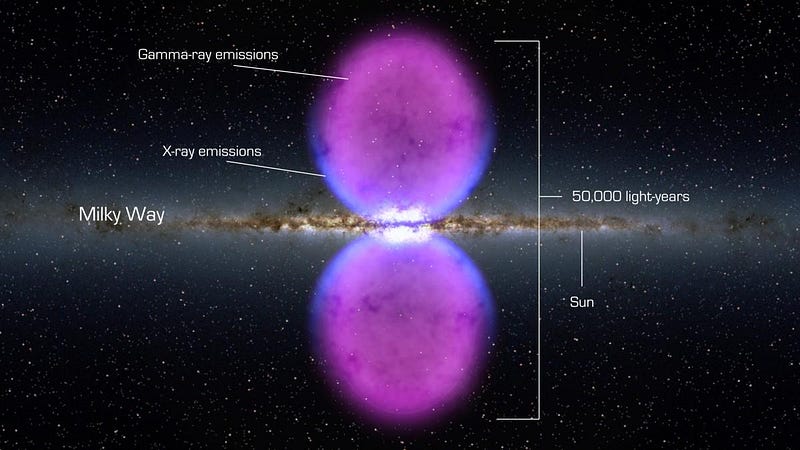
Two bubbles of high-energy signatures are evidence that electron/positron annihilation is occurring, likely powered by processes at the galactic center. Here on Earth, more positrons than can be explained by conventional physics are seen via direct cosmic ray experiments, putting forth the exciting possibility that dark matter might be the cause of both that excess and the galactic center gamma rays. (NASA’S GODDARD SPACE FLIGHT CENTER)
However, we also expect that there is some new physics out there, because of the overwhelming astrophysical evidence for dark matter. While the true nature of dark matter will remain a mystery until the particle (or at least one of the particles) responsible for it is detected directly, many dark matter scenarios exist where not only is dark matter its own antiparticle, but that dark matter annihilations will also produce electron-positron pairs.
Whenever you have multiple possible physical explanations for what could cause an observable phenomenon, the key to telling which one matches reality is to tease out differences between the explanations. In particular, positrons due to dark matter should experience a cutoff at specific energies (corresponding to the mass of the dark matter particles), while positrons generated by conventional astrophysics should fall off more gradually.
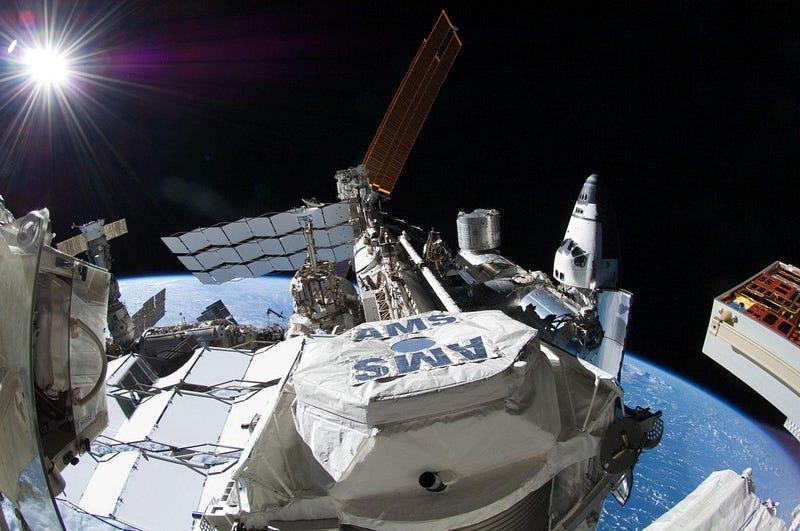
Exterior view of the ISS with the AMS-02 visible in the foreground. The AMS-02 experiment was installed in 2011, and has provided our best measurements of cosmic rays by type and energy of any experiment to date. (NASA)
In 2011, the Alpha Magnetic Spectrometer experiment (AMS-02) was launched with the goal of further investigating this mystery. After arriving at the International Space Station aboard the final mission of the Space Shuttle Endeavor, it was quickly set up and began sending data back to Earth within 3 days. During its operational phase, it collected and measured more than ten billion cosmic ray particles per year.
What’s remarkable about AMS-02 is that it didn’t just measure cosmic ray particles, but was able to sort them both by type and by energy, providing us with an unprecedented set of data to evaluate whether the positrons appeared to be due to dark matter or not. At low energies, the data matched the predictions of cosmic rays colliding with the interstellar medium, but at higher energies, something else was clearly at play.
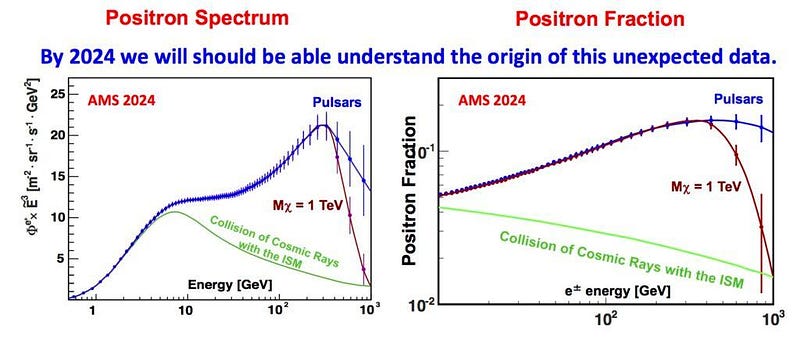
If the AMS-02 experiment had not experienced any failures or required any repairs, it would have collected sufficient data to distinguish between pulsars (blue) or annihilating dark matter (red) as the source of the excess positrons. Either way, collisions of cosmic rays with the interstellar medium can only explain the low-energy signature, with another explanation required for the high-energy signatures. (AMS COLLABORATION)
However, that’s not a slam dunk for dark matter by any means. At higher energies, it’s also possible that pulsars, which accelerate matter particles to incredible energies through a combination of their gravitational and electromagnetic forces, could produce a peaked excess of positrons at high energies.
Although AMS-02 sees evidence (at 4-sigma, or 99.99% confidence) that there’s a peak and then a falloff in the observed energies of positrons, its sensitivity and event rate peters out at exactly the types of energies that would enable us to differentiate between a positron signal arising from pulsars versus one arising from annihilating dark matter. With spacewalks currently ongoing to attempt to repair AMS-02 and bring it back online to continue its observations, it may eventually collect enough data to discern, on its own, whether pulsars or dark matter provide the best fit to the data.
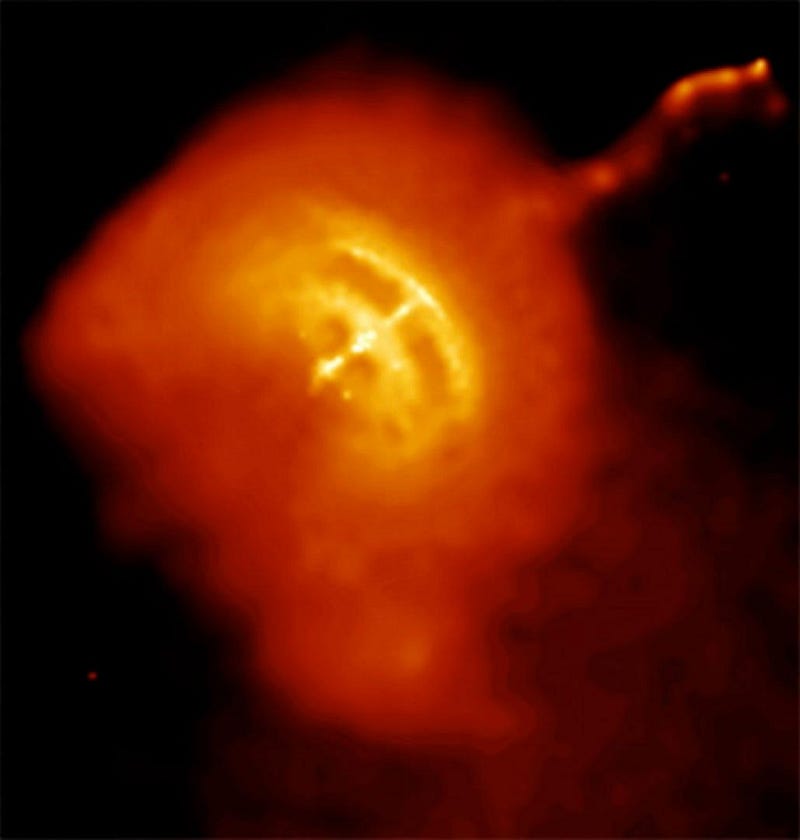
The Vela pulsar, like all pulsars, is an example of a neutron star corpse. The gas and matter surrounding it is quite common, and is capable of providing fuel for the pulsing behavior of these neutron stars. Matter-antimatter pairs, as well as high-energy particles, are produced in copious amounts by neutron stars, offering up the possibility that they, and not dark matter, are responsible for the excess signals observed by AMS-02. (NASA/CXC/PSU/G.PAVLOV ET AL.)
However, there’s more than one way to tell these two scenarios apart, as positrons produced by pulsars should also generate an additional signal that falls well outside the measurements that AMS-02 or any cosmic ray experiment could detect: gamma rays.
If pulsars truly generate the positrons that could be responsible for the signal that cosmic ray experiments are seeing, then a significant fraction of those positrons will have the misfortune of colliding with electrons in the interstellar medium long before they arrive at our cosmic ray detectors. When positrons collide with electrons, they annihilate, with each reaction producing two gamma rays with a very specific energy signature: 511 keV of energy, the rest-energy equivalent of an electron’s (or positron’s) mass, also obtained from Einstein’s E = mc2.
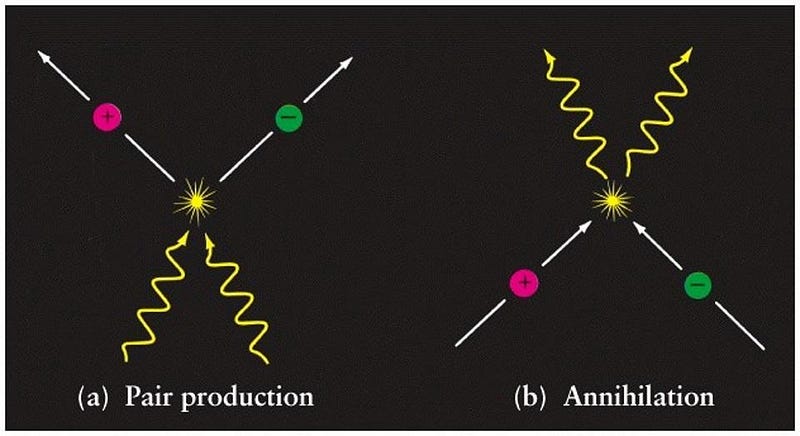
The production of matter/antimatter pairs (left) from pure energy is a completely reversible reaction (right), with matter/antimatter annihilating back to pure energy. When a photon is created and then destroyed, it experiences those events simultaneously, while being incapable of experiencing anything else at all. If you operate in the center-of-momentum (or center-of-mass) rest frame, particle/antiparticle pairs (including two photons) will zip off at 180 degree angles to one another, with energies equal to the rest-mass equivalent of each of the particles, as defined by Einstein’s E = mc². (DMITRI POGOSYAN / UNIVERSITY OF ALBERTA)
However, pulsars should theoretically be able to accelerate these electrons and positrons up to extraordinarily high energies: energies that even the world’s most powerful terrestrial particle accelerator, the Large Hadron Collider, struggles to reach. When photons — even normal-energy starlight — interact with these ultra-relativistic (near light-speed) particles, they can get boosted to extraordinary energies through a process known as inverse Compton scattering.
Based on physical parameters like the properties of the pulsar, the matter in the pulsar’s vicinity, the electrons and positrons generated, and the amount of starlight present nearby, a specific energy spectrum will be created for the photons generated from this process. Sum them all up for all of the nearby, relevant pulsars, and your gamma ray signature might indicate that pulsars, and not dark matter, cause this positron excess.
Particles traveling near light speed can interact with starlight and boost it to gamma-ray energies. This animation shows the process, known as inverse Compton scattering. When light ranging from microwave to ultraviolet wavelengths collides with a fast-moving particle, the interaction boosts it to gamma rays, the most energetic form of light. (NASA / GSFC)
About 800 light-years away, incredibly close by astronomical standards, one of the brightest gamma-ray pulsars in the entire sky can be found: Geminga. It was only discovered in 1972, and had its nature revealed in 1991, when the ROSAT mission measured evidence for a neutron star spinning at a rate of 4.2 revolutions-per-second.
Fast-forward to the present day, where NASA’s Fermi Large Area Telescope — with enormously improved spatial and energy resolution — is now the world’s most sophisticated gamma ray observatory. By subtracting out the gamma ray signal arising from cosmic rays colliding with interstellar gas clouds, the remnant signal from starlight interacting with accelerated electrons and positrons could be revealed.
When a team of researchers led by Mattia di Mauro analyzed the Fermi data, what they saw was spectacular: an energy-dependent signal that, at its largest, spanned some 20 degrees in the sky at the exact energies that AMS-02 was most sensitive to.
This model of Geminga’s gamma-ray halo shows how the emission changes at different energies, a result of two effects. The first is the pulsar’s rapid motion through space over the decade Fermi’s Large Area Telescope has observed it. Second, lower-energy particles travel much farther from the pulsar before they interact with starlight and boost it to gamma-ray energies. This is why the gamma-ray emission covers a larger area at lower energies. (NASA’S GODDARD SPACE FLIGHT CENTER/M. DI MAURO)
Explaining this glow, which decreases in size as Fermi looks at progressively higher energies, fit the models perfectly by leveraging a combination of inverse Compton scattering with the pulsar’s motion through interstellar space. According to Fiorenza Donato, coauthor on the recent Fermi study that measured gamma rays from Geminga,
Lower-energy particles travel much farther from the pulsar before they run into starlight, transfer part of their energy to it, and boost the light to gamma rays. This is why the gamma-ray emission covers a larger area at lower energies. Also, Geminga’s halo is elongated partly because of the pulsar’s motion through space.
The measurement of the gamma rays from Geminga alone suggests that this one pulsar could be responsible for as much as 20% of the high-energy positrons seen by the AMS-02 experiment.
This animation shows a region of the sky centered on the pulsar Geminga. The first image shows the total number of gamma rays detected by Fermi’s Large Area Telescope at energies from 8 to 1,000 billion electron volts (GeV) — billions of times the energy of visible light — over the past decade. By removing all bright sources, astronomers discovered the pulsar’s faint, extended gamma-ray halo, concluding that this one pulsar could be responsible for up to 20% of the positrons detected by the AMS-02 experiment. (NASA/DOE/FERMI LAT COLLABORATION)
Whenever there’s an unexplained phenomenon that we’ve measured or observed, it presents a tantalizing possibility to scientists: that perhaps there’s something new at play beyond what’s presently known. We know there are mysteries about our Universe that require new physics at some level — mysteries like dark matter, dark energy, or the cosmic matter-antimatter asymmetry — whose ultimate solution has yet to be discovered.
However, we cannot claim evidence for a new discovery until everything that represents what’s already known is quantified and accounted for. By factoring in the effect of pulsars, the positron excess observed by the Alpha Magnetic Spectrometer collaboration may turn out to be explicable entirely by conventional high-energy astrophysics, with no need for dark matter. Right now, it appears that pulsars may be responsible for 100% of the observed excess, requiring scientists to go back to the drawing board for a direct signal that reveals our Universe’s elusive dark matter.
Ethan Siegel is the author of Beyond the Galaxy and Treknology. You can pre-order his third book, currently in development: the Encyclopaedia Cosmologica.