The fabric of space is a Cheshire Cat
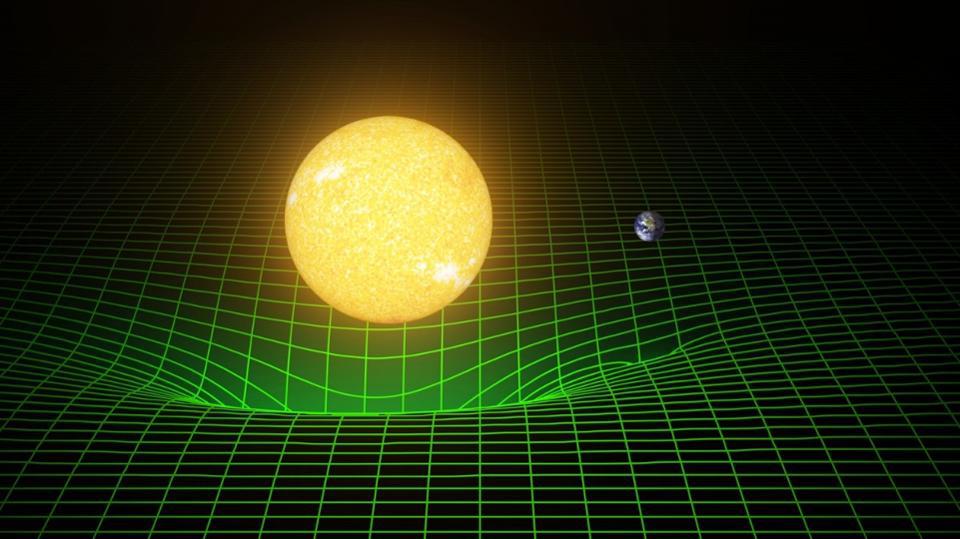
- Physicists have long understood waves — including water waves, sound waves, and waves on a string — as some type of energetic signal that travels, or propagates, through a medium.
- In the 1800s, physicists demonstrated that light is a wave, too, but the search for a medium that light propagates through came up empty: the supposed “luminiferous aether” doesn’t need to exist.
- Light, gravitational waves, and all other quantum phenomena propagate just fine, requiring no detectable medium to travel through. It’s a puzzle as curious as a Cheshire Cat.
“Well! I’ve often seen a cat without a grin,” thought Alice, “but a grin without a cat! It’s the most curious thing I ever saw in my life.” In Lewis Carroll’s imagination, the now-common picture of a Cheshire Cat took shape: where a cat’s grin could persist independent of the cat itself. It sounds like a complete absurdity, but in the world of physics, intuitively absurd notions are sometimes the only explanation that fits what the Universe presents to us.
For a long time, we understood waves as entities that propagate through some sort of medium. Water waves require a body of water to travel through. Seismic waves require a planetary object to travel through. Sound waves require something (normally air, but rock, bone, and other media work too) to travel through. If you take the medium away — no water, no planet, no air or other material, etc. — there can be no wave at all.
This held true up until the 1800s, when scientists first demonstrated the wave-like nature of light. While most assumed there was a medium that light traveled through, even naming it the luminiferous aether, experiment after experiment failed to reveal any sort of medium at all. As absurd as it may seem, what we think of as “empty space” doesn’t have the properties fundamental to all media that waves travel through, making the fabric of space the Cheshire Cat of the very Universe itself.

When it comes to how waves work, one of the simplest examples you can experiment with for yourself is to simply drop a stone into an otherwise still body of water, such as a pond or a puddle. As the stone strikes the water, it pushes the water down and out-of-the-way. This leads to a crest (or high point) forming in a circular pattern around where the stone struck the water, followed by a trough (or low point) behind it. Most often, a series of crests-and-troughs are produced: what physicists understand as a wave or a wave pattern. These waves are disturbances in the water itself: the water serves as a medium for this wave to travel through, and the wave propagates at a particular speed dependent on the properties of the water itself.
What if, instead of a dropped stone, you took a long, straight stick and dropped that into the water instead?
It would produce what physicists know as a plane wave, where instead of waves emerging from a single point (and spreading out circularly in two dimensions across the water’s surface), those waves would propagate linearly: with crests-and-troughs propagating parallel to the dropped stick itself. Remarkably, this was understood all the way back in the 1600s, when Christiaan Huygens demonstrated the equivalence between a large number of circular waves (imagine replacing your stick with 1000 small rocks, all lined up in a row) and one linear plane wave. So long as you had a medium that non-uniform disturbances could propagate through, waves seemed like an inevitable consequence.
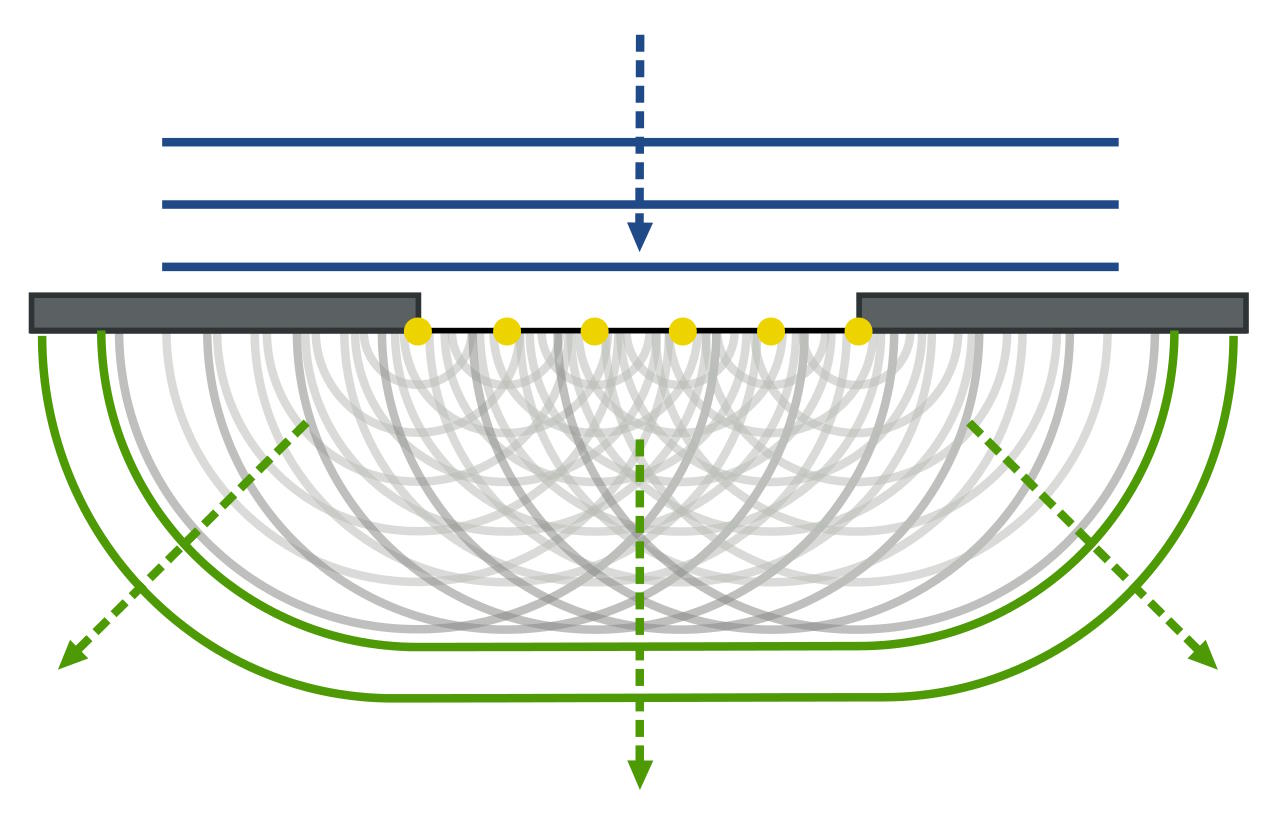
Although there are many types of waves we encounter in our daily lives — sound waves, water waves, seismic waves, etc. — these are all examples of the same class of wave: traveling waves, which propagate across or through a medium, as opposed to standing waves. Water waves travel through the body of water itself; seismic waves on our planet travel through the Earth; sound waves typically travel through the air. The sensation of hearing arises when that air, full of compressions (peaks) and rarefactions (troughs) in density, then pushes against our eardrums, causing the hairs within our cochlea to vibrate, stimulating the nerves that detect sound.
You might protest this interpretation of waves, noting that standing waves — like the waves generated by a plucked guitar string — also exist. They do, of course, but only when the waving or oscillating object (the string, in this case) is stiff enough: in the case of a guitar string, is held under sufficient tension between two fixed points. The string oscillates back-and-forth, but that isn’t the sound you hear. Instead, it’s the fact that the oscillation of the string then gets amplified (by an acoustic guitar’s resonance, or by an electric guitar’s pickups and the speaker/amp that’s attached), thereby creating traveling waves that move through the air. There needs to be a medium for these traveling waves to travel through, or the wave will simply cease to be when it reaches the end boundary of the medium itself.
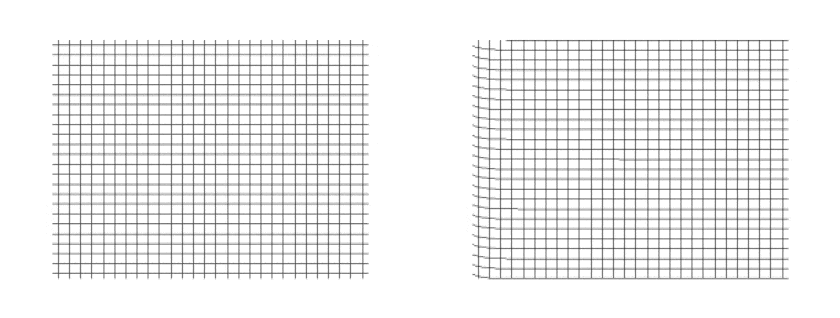
There’s one more caveat when it comes to these types of traditional — or classical — waves that we need to consider: motion. If you, the observer, are in motion relative to the wave that you’re observing or receiving, the crests-and-troughs will pass by you at a different rate than if you were stationary: at a higher frequency (more often) if you’re moving toward the wave, and at a lower frequency (less often) if you’re moving away from the wave. If you can move as fast as, or faster than, the wave itself, then it becomes possible to outrun the wave entirely: if you move away from the wave faster than the wave itself travels through the medium, it will never catch you.
Similarly, if the medium itself is in motion, it too will change the frequency of the waves that you see. We can return to the example of the stone dropped into the water, except this time, instead of a pond or puddle, imagine the water has a current, like a flowing river. Now, the ripples still propagate circularly outward through the water, but the water is in motion. If you’re floating on the water and also moving with the current, the ripples will pass you by just like they did in the earlier, stationary example: because you are also in motion along with the medium itself. However, if you’re on the shore, or if you’re on a firmly anchored rock that pokes out above the surface, you’ll see the effect of both motions — the rippling waves plus the water’s current — imprinted on the waves as they pass you by. The waves require the medium to exist, and the medium’s properties, including its motion, affect our observations of them.
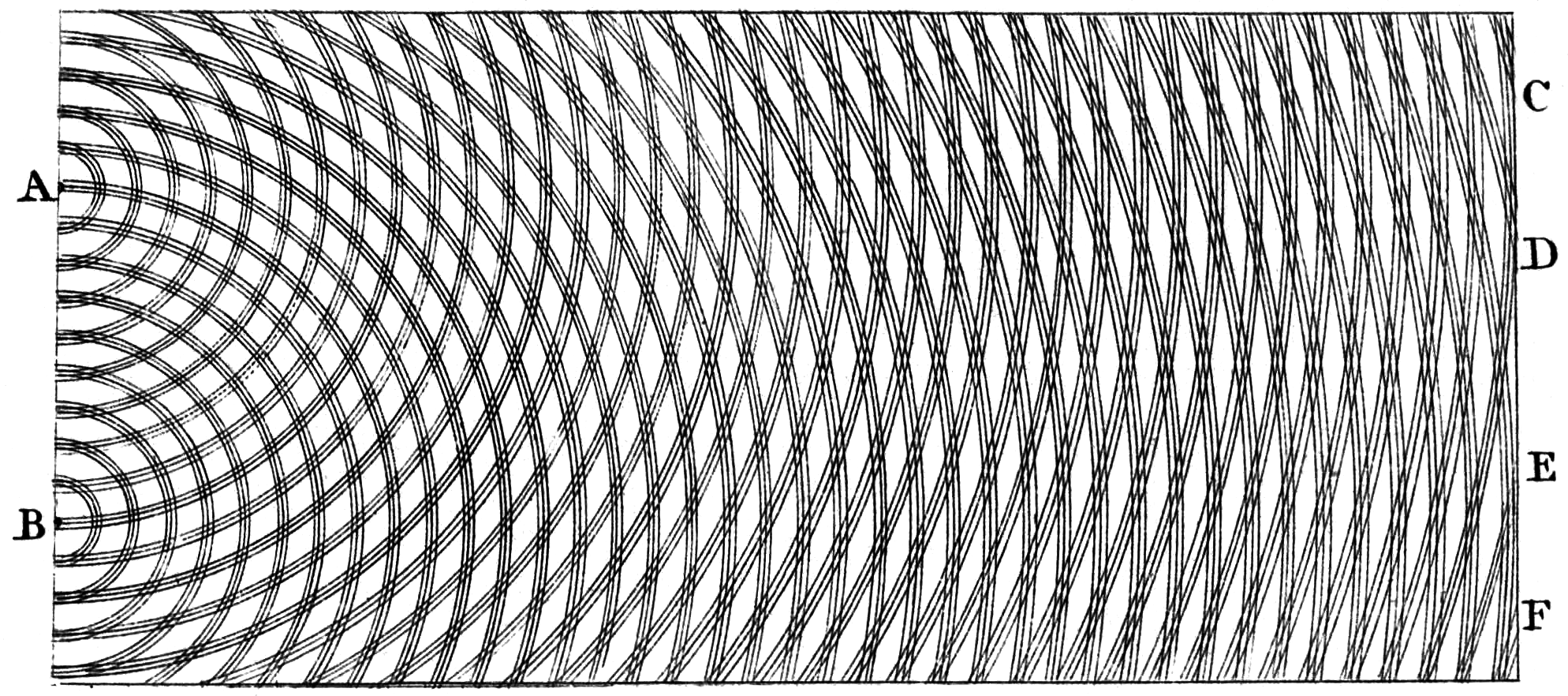
And then the first major puzzle arrived: light. As early as the 1600s, scientists were arguing over the nature of light: whether it was wave-like, as favored by Huygens, or whether it was corpuscular (i.e., particle-like), as Newton argued. It was only when experiments with light began to reveal phenomena like interference and diffraction, phenomena that would not exist if light were purely particle-like, that the wave-like nature of light was established.
The first key piece of evidence came from Thomas Young, who performed the earliest recorded double slit experiment with light. By taking light waves of a constant frequency and shining it at a barrier with two narrowly-spaced slits cut into it, Young was able to show that instead of two bright “lines” appearing on the opposite side of the barrier — which is what you’d expect if light were corpuscular, with each line corresponding to light traveling in a straight line through one slit or the other — this light produced a large number of bright-and-dark fringes in succession.
This was clear evidence for an interference pattern, just as Huygens had shown for water waves produced by stones dropped into a pond. This would only work if light were a wave, but it wasn’t enough to convince everyone.
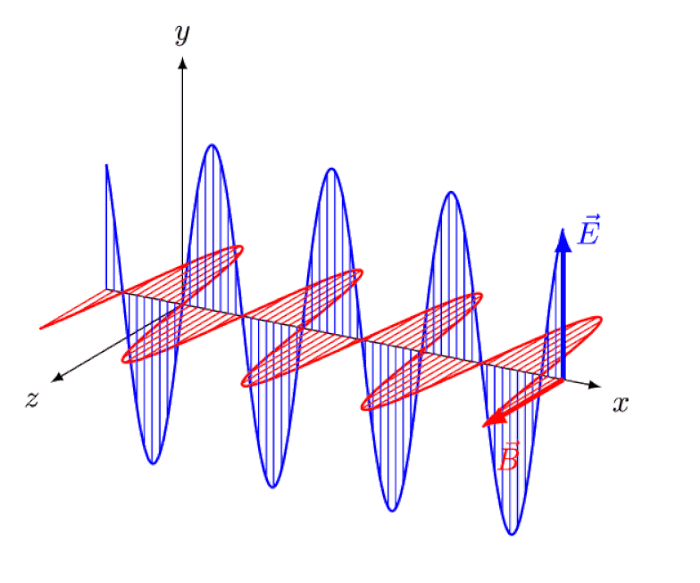
Then, in 1818, the French Academy of Sciences held an essay competition on the nature of light, where a young physicist named Fresnel decided to enter. His essay detailed the wave theory of light, using Huygens’ principle of wavefronts and Young’s principle of interference to produce a full quantitative description of light, explaining diffraction and the wave-like principle of superposition in the process.
One of the judges happened to be Simeon Poisson, a fierce adherent to a Newtonian worldview, who used Fresnel’s theory to show it led to absurd conclusions. In particular, Poisson showed that if you passed a beam of monochromatic light around a spherical obstacle, you wouldn’t get a bright ring with a solid, circular shadow within it, but instead there would be a bright, luminous point directly in the shadow’s center. Clearly, Poisson argued, this is absurd, and Fresnel’s wave theory of light should be rejected.
Fortunately for us all, François Arago was also on the judging committee, and was moved by Poisson’s argument, but not as Poisson intended. Instead of dismissing Fresnel, Arago went and performed the experiment himself using the tools available at the time. Remarkably, this spot, now known as the Spot of Arago, does in fact appear and exist! Light is a wave, after all.
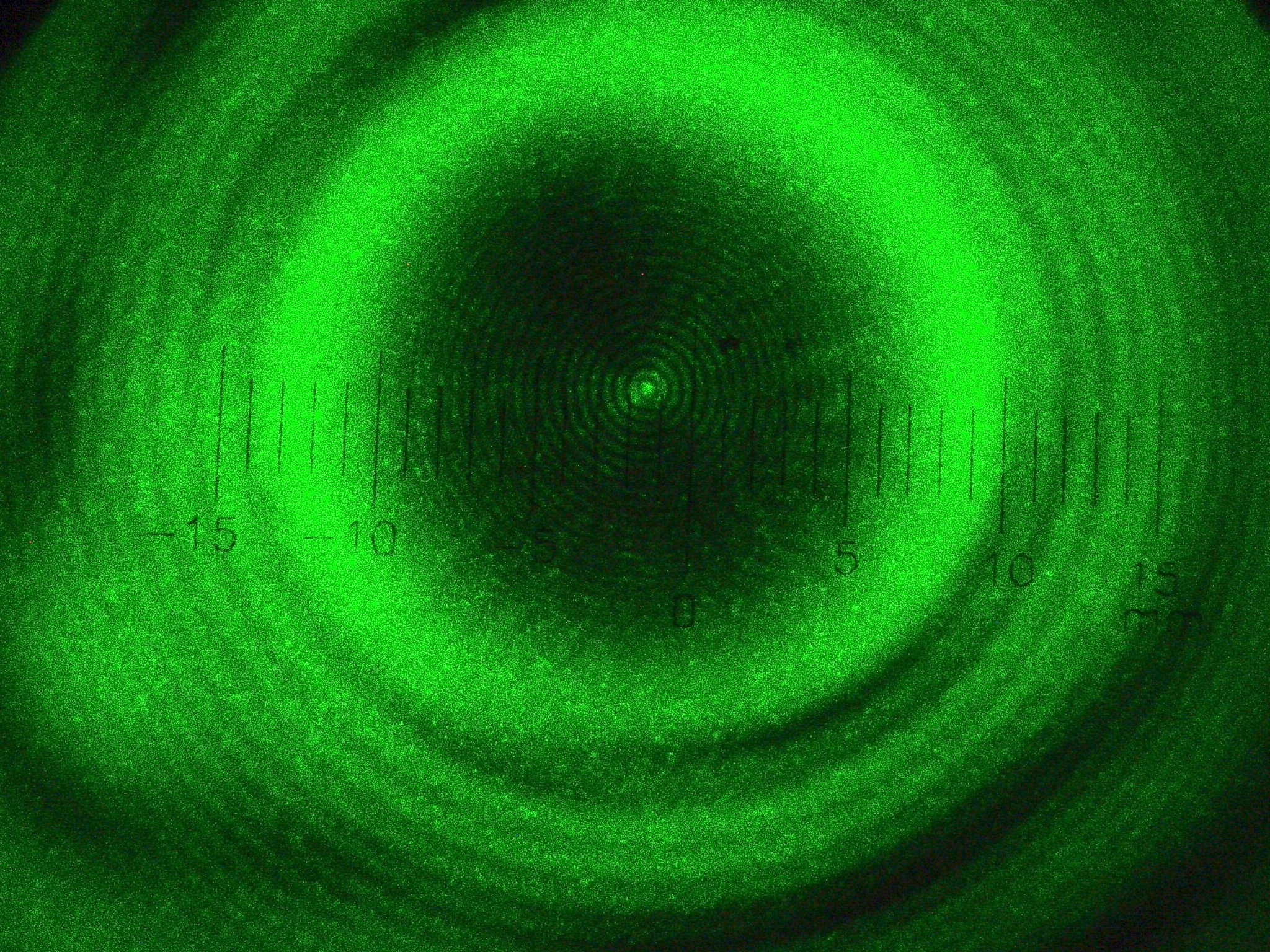
What, then, was the medium through which light traveled? Although we hadn’t detected it, most scientists knew it had to exist, and even concocted a name for it: the luminiferous aether, a medium for light to propagate through. By the 1870s, Maxwell’s equations had shown what type of wave light is: it’s an electromagnetic one, with oscillating, in-phase electric and magnetic fields. If light is a wave that travels through the luminiferous aether, then even though the speed of light is huge (around 300,000 km/s), the Earth’s motion around the Sun (at ~30 km/s) is a substantial fraction of that: around 0.01%.
If light travels through a medium, and Earth is in motion, then we should be able to detect that motion with a sensitive enough experiment. That’s precisely what the Michelson-Morely experiments did in the 1880s: the same experiment we thought about with water ripples created in a river, but with light and the moving Earth rather than with water and a flowing river.
Remarkably, the experiments found no evidence for this medium — or any medium — at all. Light traveled neither slower nor faster whether it moved with, against, or perpendicular to the Earth; it traveled at the same speed (the speed of light) no matter what experiment was performed.

What did this mean? It wasn’t until Einstein came along in 1905 that physics got our classic “Lindsay Lohan from Mean Girls” moment, only instead of “the limit does not exist,” Einstein’s great insight was to consider the Cheshire Cat-like possibility that the medium does not exist! This was the great insight of special relativity: that light didn’t travel through a medium like other waves, where the motion of the medium (or the motion of the observer relative to the light wave) would change the wave’s speed. Instead, the speed of light itself was the constant, unchanging, universal property, and while a moving observer might see the light’s crests-and-troughs passing them more or less frequently dependent on their motion, the speed of light itself never changed.
Ten years later, in 1915, Einstein incorporated gravity into the theory of relativity: giving rise to general relativity. Similar to how light is a wave in the electromagnetic field — even though the field itself can have any value, including zero, and even though there’s no medium needed for it to travel through — the gravitational field can have waves in it too: gravitational waves. Once again, as gravitational waves propagate, they can do so:
- through any gravitational field,
- including in places where the gravitational field is zero,
- and always move at the same speed — the speed of gravity — regardless of the motion of the source or the observer.
As with light, there’s no evidence for the existence of a medium that appears in any experiment or observation, even though we’ve now detected hundreds of gravitational waves directly.
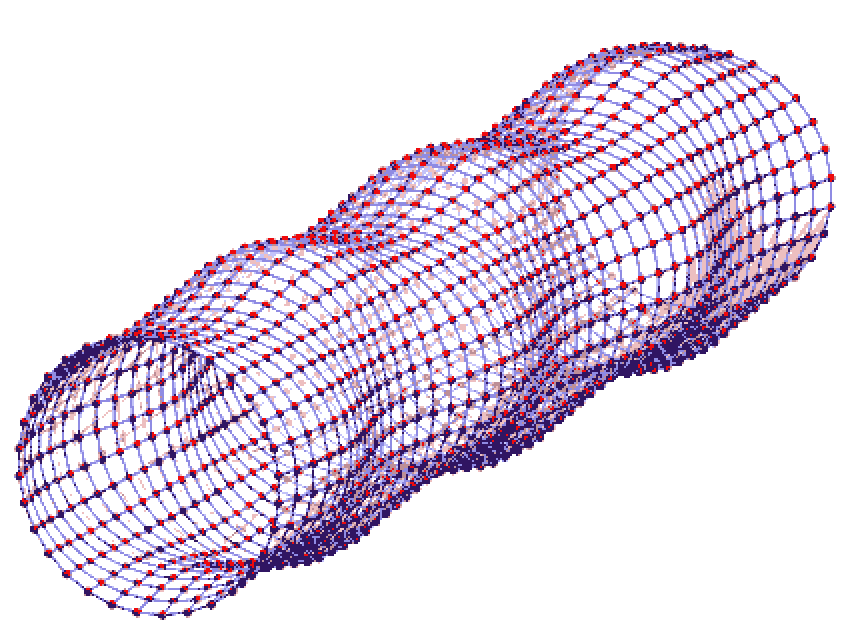
Here in the 21st century, our best picture of the Universe is based on quantum fields. In addition to the gravitational field (for which a quantum description has proven elusive so far) and the electromagnetic field, there’s also the weak nuclear field, the strong nuclear field, and the Higgs field, all of which appear to have this same Cheshire Cat-like property: a field without a medium, just like the Cheshire Cat gives a grin without a cat. Interestingly, Einstein — who first gave us this Cheshire Cat for light, whether we liked it or not — grew to hate it. After putting forth general relativity, Einstein instead began to view spacetime itself as a fabric, or in different terms, as a medium.
Was he correct?
We still don’t know. Empty space (or spacetime) is certainly “real” in the sense that it exists; it’s where everything in the Universe lives! We’re certain that a medium isn’t necessary, and that if space itself is such a medium, it doesn’t have any of the traditional properties that we associate with any other known medium. All massless waves — light waves, gravitational waves, even gluon waves — travel at the same speed, as the speed of light and the speed of gravity are equal, but whether this is a hint of an underlying medium or just a fact of reality remains undetermined.
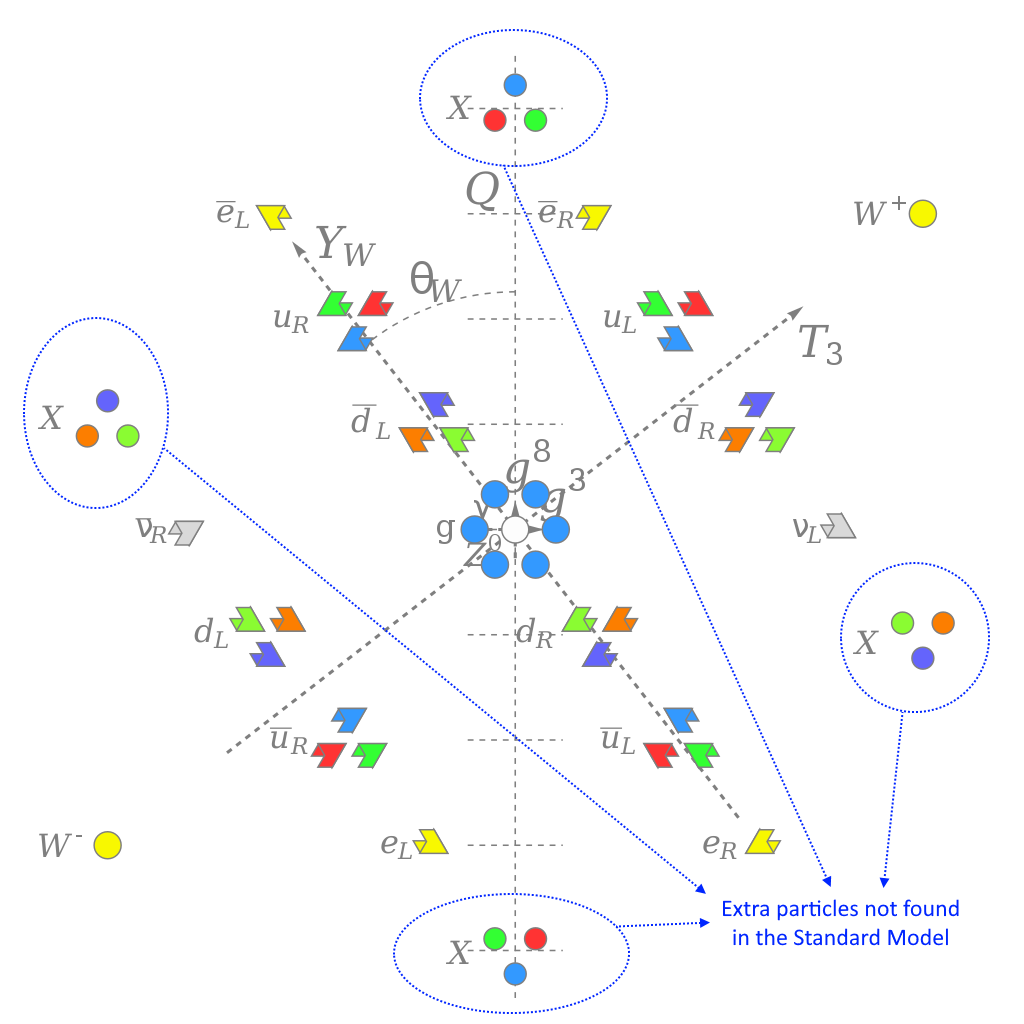
There’s a huge hint against the notion that there is an underlying medium for these waves to propagate through, however: attempts at unifying fields and forces. Back in the early 1920s, Theodr Kaluza and Oskar Klein attempted to unify gravitation and electromagnetism by adding an extra dimension of space. Lo and behold: Einstein’s general relativity and Maxwell’s equation for electromagnetism both popped out! But they were linked together by a new field: the radion. But none of the predicted properties of the radion are borne out by experiment or observation; they must all be removed, somehow, in order to recover the Universe we see.
Other extensions to theoretical physics — Brans-Dicke gravity, grand unification theories, supersymmetry, extra dimensions, string theory — suffer from the same problem. Despite how appealing the idea of a medium is for waves to travel through, the evidence for it not only doesn’t exist, but the evidence we have collected tightly constrains all of these scenarios. There are no scalars in gravity; there is no proton decay; there is only one Higgs boson; there are no relics of extra dimensions; there’s no “bleeding” of electromagnetic phenomena into gravity.
It still may be the case that there is a medium, or some type of unknown aether, that truly does underlie all of reality, but if it does, we have no evidence for it. Nature is telling us that we have waves propagating in our Universe without the need for a medium at all: the most mysterious Cheshire Cat we’ve ever encountered.
Ethan Siegel acknowledges the brilliant book Waves in an Impossible Sea, by Matt Strassler, for the concept of a “field without a medium” as a Cheshire Cat.