If photons have mass, could they explain dark matter?
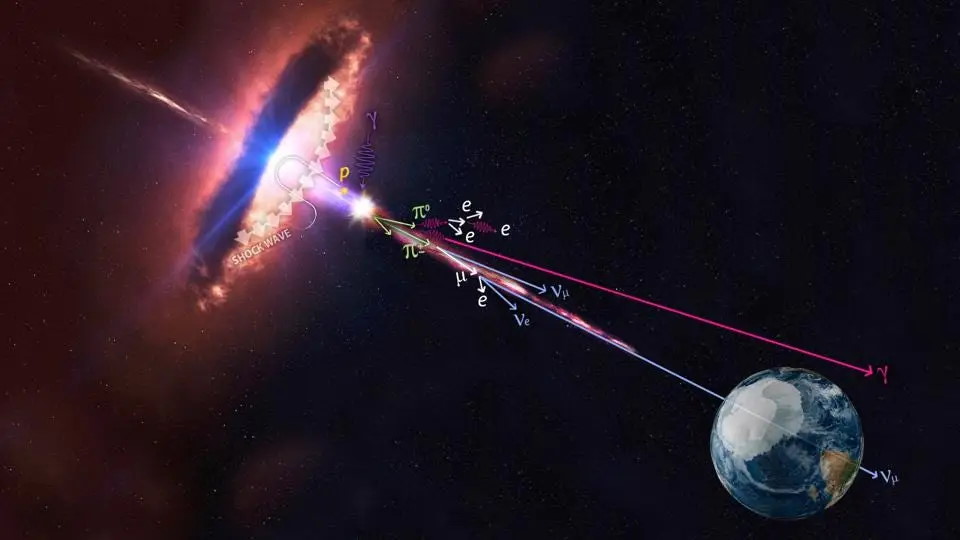
- Although there’s overwhelming evidence that dark matter exists from an astrophysical perspective, every single direct detection effort has come up empty, rendering dark matter’s nature a cosmic mystery.
- Many possible dark matter candidates abound, and while it’s easy to imagine a way in which there can be extra matter that evades detection limits in our Universe, it’s difficult to match the astrophysical observations.
- One idea that’s been floating around physics for some time is that of massive photons. Could they be the sought-after solution to this cosmic mystery?
When it comes to the Universe, there are some things we can be confident are out there based on what we observe. We know that the Universe was hotter, denser, and more uniform in the distant past. We know that the stars and galaxies in the Universe have grown up and evolved as the Universe has aged. We know that gravitation has formed the large-scale structure in the Universe, and that structure has grown more complex over time. And we also know how much normal matter, altogether, is present in the Universe, and that it isn’t sufficient to explain the full suite of the gravitational effects that we see on its own.
So if the normal matter can’t be all that there is, what else can there be? The leading idea is dark matter, but we don’t know precisely what it is. What if it’s just light? Is that possible? That’s what Enon Travis Sci wants to know, asking:
“I’d love to read an article on why light isn’t a dark matter candidate, especially in light (pun intended) of the recent articles about the 50% excess in ambient light in the universe, and the known phenomena of apparent mass photons can have on spacetime…”
It’s a completely reasonable idea to consider. Let’s take a look inside.
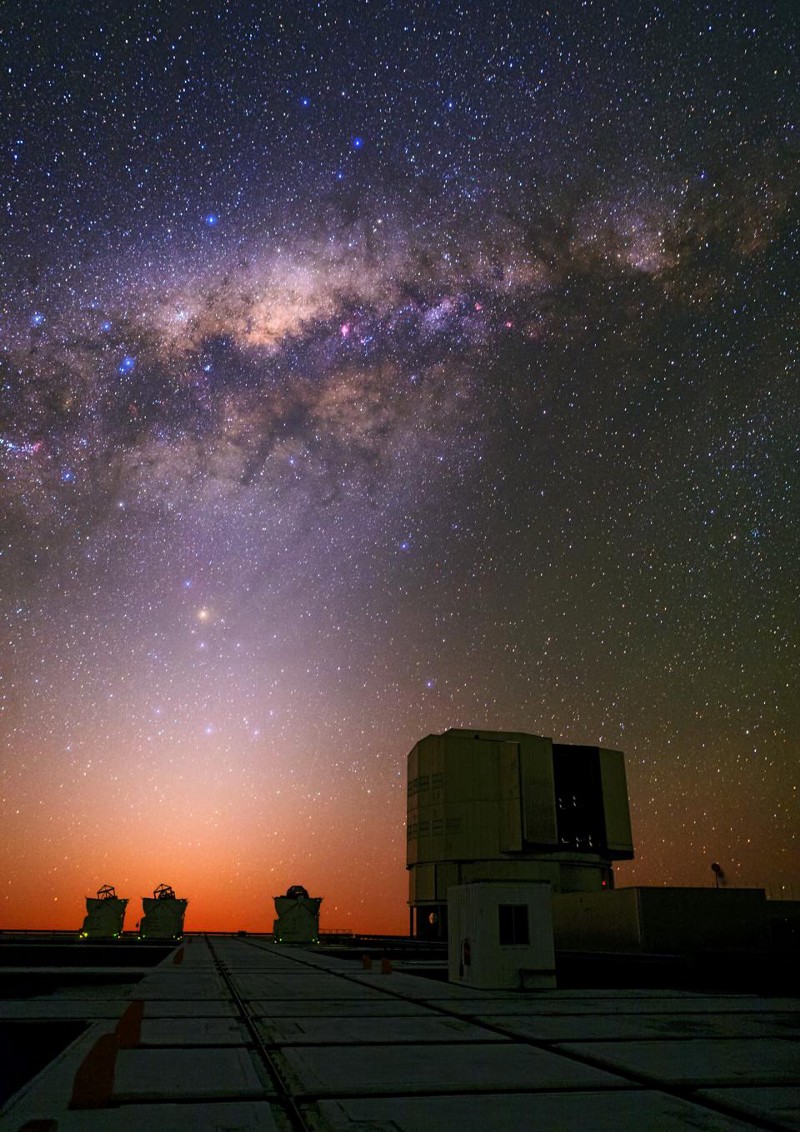
First off, there really is an excess in the ambient light we see in the Universe. In fact, I myself wrote about it back in 2020, because it’s one of the most intriguing things that the New Horizons spacecraft was able to measure from its unique location: out beyond the orbit of Pluto. Among all fully functioning spacecraft, only New Horizons is distant enough to be immune to the tiny, light-reflecting dust grains that exist all throughout our Solar System, and see what sort of light still remains.
Is deep space entirely dark?
You might think it ought to be, but that’s not what the New Horizons team found. There was excess light from expected sources: camera noise, scattered sunlight, excess off-axis starlight, crystals from the spacecraft thrust, and other instrumental effects all create an excess of light. But those effects can all be modeled, and when they are, their magnitudes and contributions can be quantified. Still, when they were subtracted out, an incontrovertible excess remained.
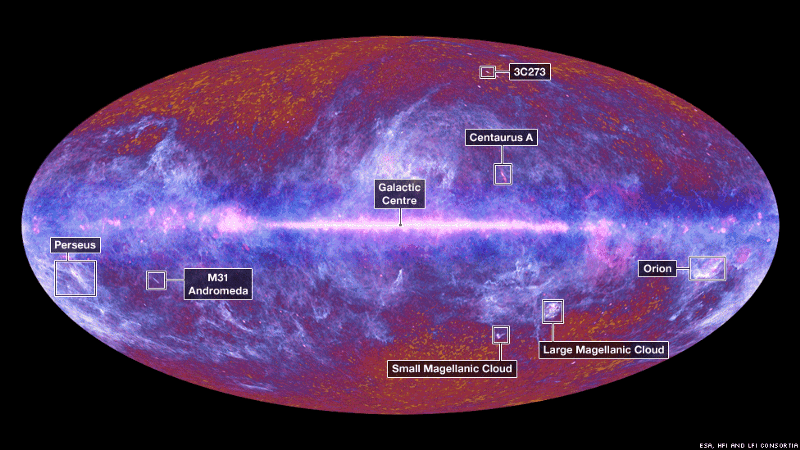
Some of that occurred in the plane of the Kuiper belt, so only observations that were out of that plane were used. Some occurred in the dust-rich plane of the Milky Way, so those observations were thrown out as well. Still, the excess persisted. What caused it? There were six theoretical contributions considered:
- stars and galaxies that we can identify,
- faint stars and galaxies that cannot (yet) be identified,
- diffuse light scattered by infrared “cirrus” clouds,
- scattered sunlight from any remaining dust in the Solar System’s outskirts,
- extra light inside the camera,
- and any diffuse cosmic optical backgrounds that aren’t associated with even hitherto unidentified sources.
After the most scrupulous accounting job they could muster, they still concluded that twice as much light was out there as the expected numbers and densities of stars and galaxies ought to produce. The light is there; there really is a diffuse, unresolved “cosmic optical background,” and we’re seeing more light in there than we would have naively expected to see.
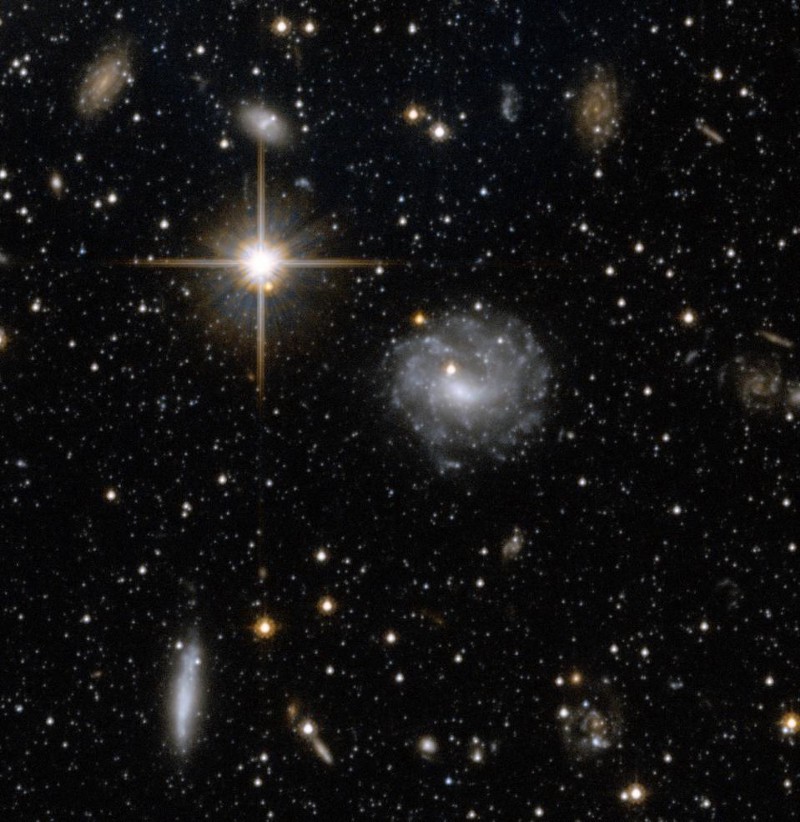
But could that excess light account for the extra gravitation we need in the Universe?
No.
There are two big reasons why. The first one is that the total amount of power in that light is minuscule: just a few dozen nanowatts per square meter of space. It’s the sum of all forms of energy that contribute to the curvature of spacetime, and it’s the clumpy forms of that energy — i.e., the ones with a non-zero rest mass — that lead to gravitational effects that are localized in space. The total fraction of energy that’s encoded in all of the light in the Universe is about 0.01% of the critical energy density, whereas the total fraction of dark matter needs to sum up to around 27% of the critical energy density. Even if we’re off by a factor of 2, or 10, or 100, we don’t come close to accounting for dark matter.
But the other reason is, perhaps, even more compelling: only things with a non-zero rest mass can behave as dark matter, and light, to the best of our knowledge, is massless. In fact, if it weren’t massless, then it wouldn’t move at the speed of light, and we have remarkable constraints on that scenario.
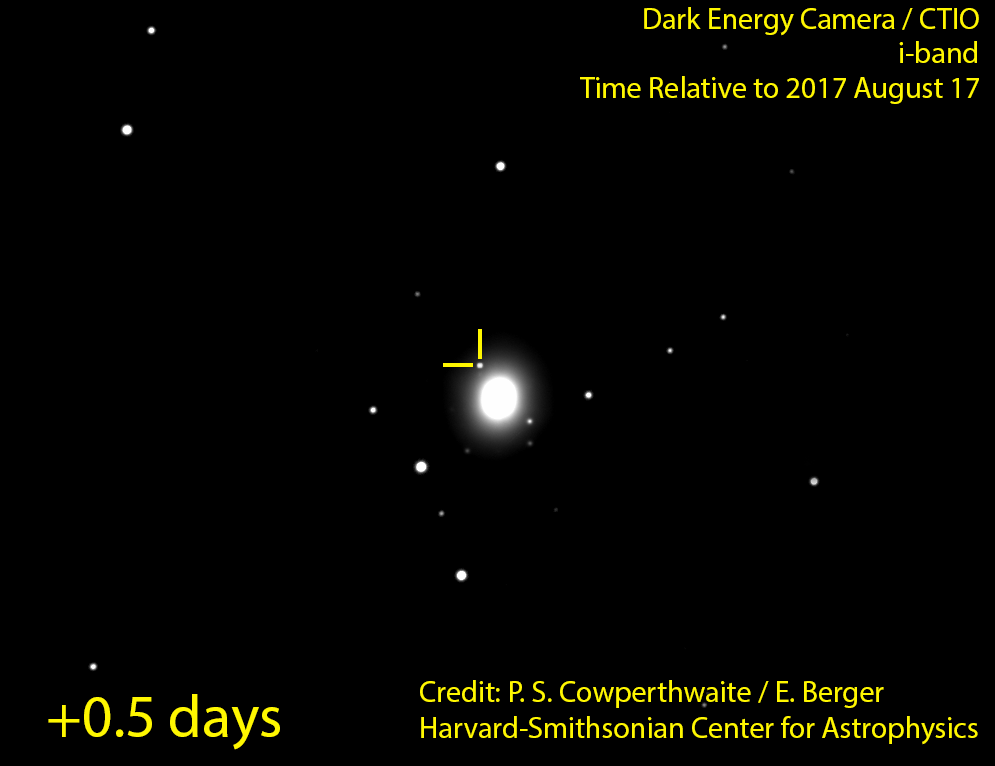
Back in 2017, an incredible observation occurred. On August 17, 2017, the Fermi Gamma-ray Burst Monitor instrument detected a localized set of high-energy photons: a classic signature of a gamma-ray burst. Gamma-ray bursts come in two varieties, a short (less than 2 second) burst, which was suspected to be caused by merging neutron stars, among other causes, and a long (more than 2 second) burst, which is thought to be from the core-collapse of massive, rapidly spinning stars. Seeing a short-period gamma-ray burst is exciting, but it’s not going to revolutionize the world of science.
At least, not on its own. What’s remarkable is that, just a few seconds earlier — as later analysis would show — the LIGO and Virgo gravitational wave detectors had also seen a signal, in gravitational waves, that was localized in the sky in precisely the same location. The signal was observable only for about 10 seconds but had all the characteristics of the final inspiral and eventual merger of two massive, compact objects. That signal abruptly came to an end at a specific moment in time, corresponding to the merger itself, which was later determined to be two neutron stars merging approximately 130 million light-years away.
And then, just 1.7 seconds later, the gamma-rays arrived in the Fermi detector, all at once.

Think about that. An event occurred: two massive, compact, astrophysical objects merged together at a specific moment in time. Those gravitational waves traveled at the speed of light across the Universe, traversing a distance of approximately 130 million light-years. (Yes, ignoring the expansion of the Universe is okay for objects this nearby; excluding it results in a distance/travel-time error of less than 1%.) At precisely the exact same moment that the merger occurs, high-energy photons are produced, and they, too, travel across the Universe, traversing a distance of 130 million light-years until they arrive at our detector.
And that’s what I want you to think about: two signals, emitted at almost exactly the same time (to within about 2 seconds of each other), traveled through space for 130 million years — about 4 × 1015 seconds — and arrived at their final destination within 2 seconds of each other. They pretty much had an ultra-ultra-ultra marathon racing head-to-head against each other, and they moved at the same speed to an accuracy that was better than one part in a quadrillion. In other words, if the photon has a mass, that mass must be so small that a racing photon and a racing gravitational wave don’t show any discernible difference in the distance they traverse over a time interval of over 100,000,000 years!

In fact, there are many lines of evidence that place similarly strong upper limits on the mass of the photon, with the magnetohydrodynamic effects of the solar wind providing the strongest robust limit that its rest mass must be less than 10-18 eV/c2, or less than one billionth-billionth-billionth of the mass of a proton. If photons do have a rest mass, that rest mass must be absolutely minuscule.
And that is enough to kill the photon as a dark matter candidate.
“Wait a minute,” I can hear you grumbling, “if it has mass, then it’s going to behave as matter. And it will clump, and it will gravitate, and if it can’t get out of the galaxy or cluster of galaxies that it’s gravitationally bound to, won’t it behave like dark matter?”
And I won’t dispute your grumbles. You’re correct. If a photon had mass, and if it had so little kinetic energy that it did fall into a galaxy or cluster of galaxies, then it would help add to the rest mass of that structure. It would gravitate, and it wouldn’t emit light of its own. It would function like dark matter.

And yet, it can’t account for the dark matter we have in our Universe. This is for one simple reason: even if photons have mass, we have to ask, “How much energy do the photons we have in the Universe actually possess?” The answer, even for the longest-wavelength, lowest-energy photons, is “much, much more energy than the maximum allowable rest mass they could have”. In fact, if you want your photons to move at speeds that are slow compared to the speed of light, they’d have to lengthen so that their wavelength was greater than about 1 billion kilometers: a little bit bigger than the approximate distance from the Sun to Jupiter.
That’s the whole problem: dark matter doesn’t just fall into massive clumps that are already present in the Universe. Instead, dark matter drives the initial formation of massive clumps in the Universe, and is the primary reason that the large-scale structure we see forms as it does. This means that dark matter needs to be moving non-relativistically, or at slow speeds compared to the speed of light, from very, very early times onward. That’s why, sometimes, you’ll hear a distinction between hot, warm, and cold dark matter. The Universe very much cares about how quickly dark matter was moving at all times compared to the speed of light.
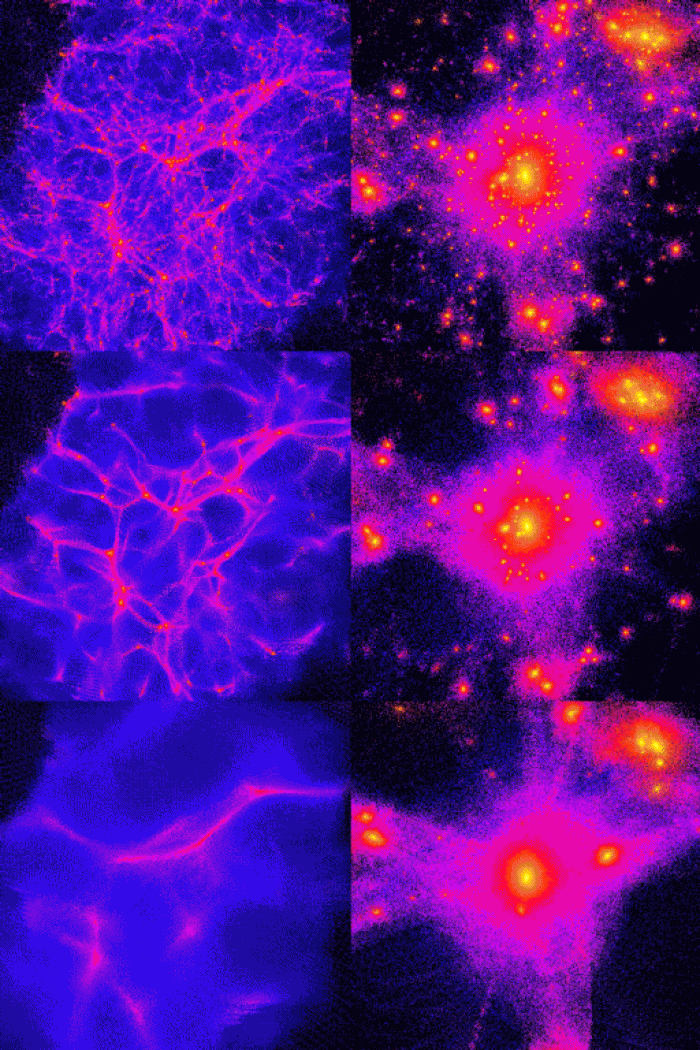
In order to agree with the observations that we have, practically a full 100% of the dark matter out there must be cold, which means it must have been moving slowly compared to the speed of light even at early times: even when the Universe was hotter, denser, and more energetic than it is today.
In fact, the Universe is actually filled with a form of hot dark matter: the neutrinos, which were created in tremendous numbers, along with photons, in the early stages of the hot Big Bang. Neutrinos have a rest mass that’s at least a factor of a trillion greater than the greatest possible mass a photon can have, and they don’t move slowly compared to the speed of light until the Universe is already hundreds of millions of years old.
Photons, even the lowest energy ones, would still be moving fast with respect to the speed of light. Even if they did have a mass, and even if they did act like dark matter, they couldn’t account for the dark matter that we actually have in the Universe. Their astrophysical effects would not correctly account for the observations of galaxies, clusters of galaxies, the cosmic web, and the imperfections that we observe in the cosmic microwave background. Dark matter must be something else.
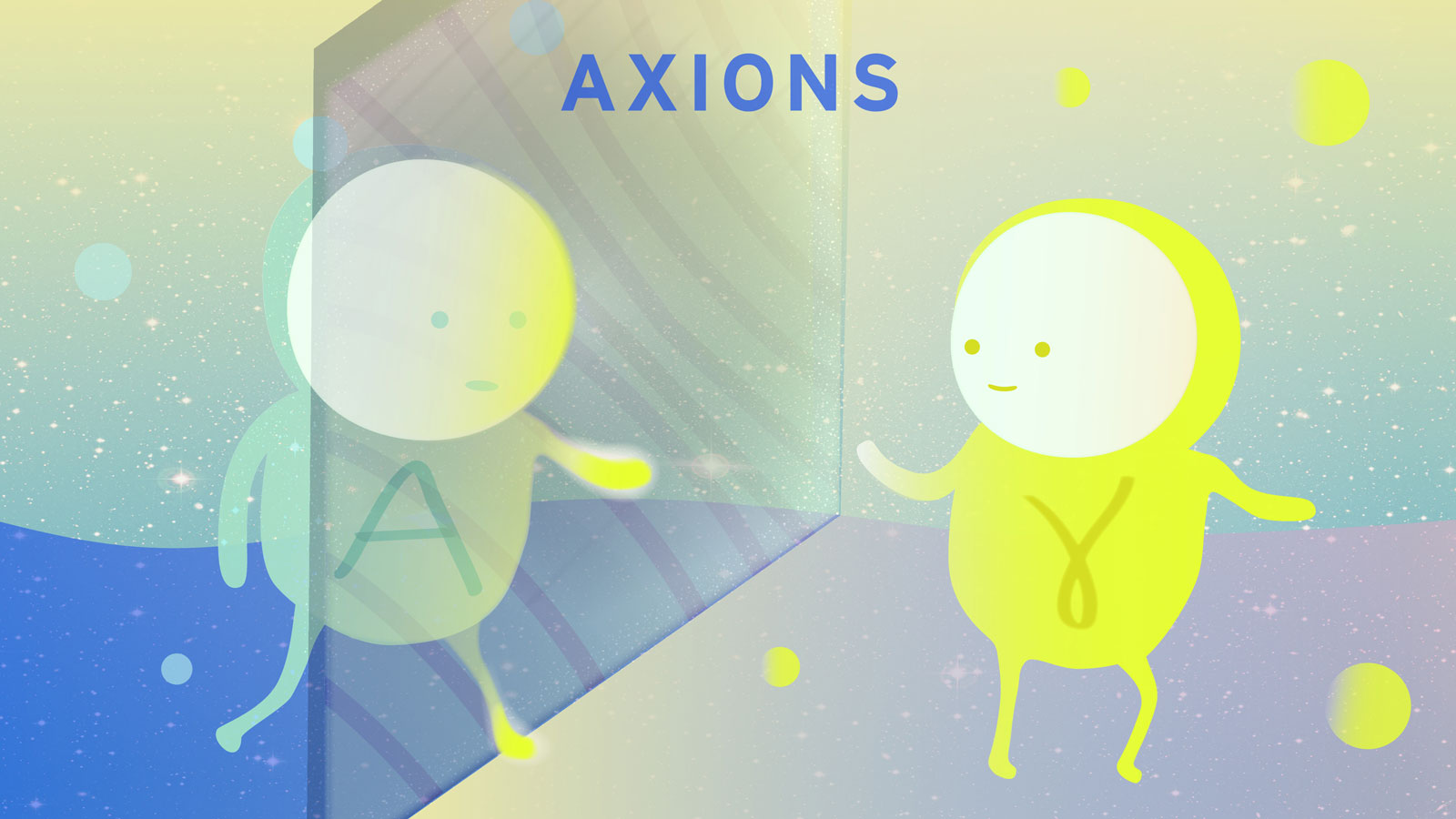
The one and only way that massive photons could, in principle, account for the dark matter we actually possess in the Universe is if they were somehow created after the Big Bang with practically no kinetic energy at all: if they were somehow born cold. Although it sounds bonkers, there’s actually a candidate dark matter particle that has a minuscule mass and comes along with a mechanism for producing them at rest: the axion.
Although the photon as-we-know-it doesn’t abide by those rules, theorists have come up with very clever modifications to the possible rules, and either a modified photon — plus an additional mechanism for them to be born, at very low energies, in very large numbers — or a related type of particle known as a dark photon could potentially become some sort of dark matter candidate. However, light as described by the rules we know, which are Maxwell’s electrodynamics and Feynman’s quantum electrodynamics, behaves as radiation, not as matter of any type.
There are lots of possibilities out there for what dark matter could potentially be. Unfortunately, none of the particles of the Standard Model, including even a massive photon, are capable of doing the job.
Ethan is on medical leave until May 6th. Please enjoy a republication of this article from the Starts With A Bang archives!