Lasers are weird and amazing
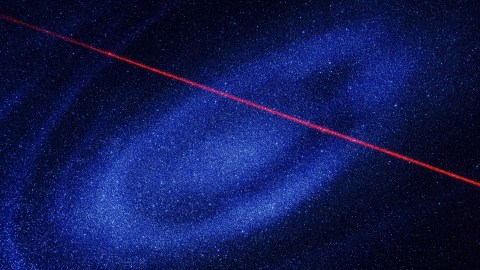
- Lasers are a quintessential quantum phenomenon.
- To make a laser, we must exploit the quantum energy levels of a particular material.
- Somehow, we humans have peered into the tiny realm of atoms and come back with a deep enough understanding to reshape the macroworld we inhabit.
The supermarket checkout scanner, the printer in your office, the pointer used in yesterday’s meeting — lasers are pretty much a part of everyday life now. You think about them very little, even as they do amazing things like instantly read barcodes or correct your nearsightedness via LASIK surgery.
But what is a laser, really? What makes them so special and so useful? Indeed, what makes a laser different from a simple lightbulb? The answers rest in the remarkable weirdness of quantum physics. Lasers are a quintessential quantum phenomenon.
Atomic energy
The key question we have to deal with here is the interaction of light and matter. In classical physics, light is made of waves of electromagnetic energy traveling through space. These waves can be emitted or absorbed by accelerating electrically charged particles of matter. This is what happens in a radio tower: Electrical charges are accelerated up and down the tower to create the electromagnetic waves that travel through space to your car and let you listen to your station of choice.
At the turn of the century, scientists wanted to apply this classical idea to create models of atoms. They imagined an atom as a little solar system, with the positively charged protons at the center and the negatively charged electrons orbiting around them. If an electron emitted or absorbed some light, i.e. electromagnetic energy, it would speed up or slow down. But this model didn’t hold. For one thing, there is always an acceleration happening when one thing orbits another — this is called centripetal acceleration. So the electron in this classical model of the atom must always be emitting radiation as it orbits — and thereby losing energy. That makes the orbit unstable. The electron would quickly fall onto the proton.
Niels Bohr got around this problem with a new model of the atom. In the Bohr model, an electron can only occupy a set of discrete orbits around the proton. These orbits were visualized like circular train tracks that the electrons rode as they circled about the proton. The farther out an orbit was from the proton, the more “excited” it was, and the more energy it held.
In the Bohr model, the emission and absorption of light was all about electrons jumping between these orbits. To emit light, an electron jumped from a higher orbit down to a lower orbit, emitting a packet of light energy called a photon. An electron could also jump from a lower orbit to a higher one if it absorbed one of these light packets. The wavelength of the light emitted or absorbed was directly related to the energy difference between the orbits.
There was much quantum weirdness in all of this. If the electron was bound to these orbits, that meant it was never between them. It jumped from one location to the other without ever occupying the intervening space. Also, light was both a particle — a photon that had a packet of energy — and a wave spread out through space. How do you imagine that? While the Bohr model was only a first step, modern versions of the theory still feature discrete energy levels and photon wave-particle duality.
Lasers make the photons jump
How does this relate to lasers? LASER stands for Light Amplification Through Stimulated Emission of Radiation. The ideas of “amplification” and “stimulated emission” in a laser are based on those specific energy levels of electrons in atoms.
To make a laser, you take some material and exploit its quantum energy levels.
The first step is to invert the population of the levels. Usually, most electrons will reside in the atom’s lowest energy levels — that is where they like to rest. But lasers rely on boosting most of the electrons to a higher, excited level — also called an excited state. This is done using a “pump” that pushes the electrons up to a specific excited state. Then, as some of these electrons begin spontaneously falling down again, they emit a specific wavelength of light. These photons travel through the material and tickle other electrons in the excited state, stimulating them to jump down, and causing more photons of the same wavelength to be emitted. By placing mirrors at either end of the material, this process builds up until there is a nice, steady beam of photons that are all the same wavelength. Some fraction of synchronized photons then escapes through a hole in one of the mirrors. That is the beam you see coming from your laser pointer.
This is exactly what does not happen in a light bulb, where atoms in the heated filament have electrons jumping up and down chaotically between different levels. The photons they emit have a wide range of wavelengths, which causes their light to look white. It is only by exploiting the weird quantum levels of electrons in an atom, the weird quantum jumps between those levels, and finally, the weird wave-particle duality of light itself, that those amazing and very useful lasers come into being.
There is, of course, a lot more to this story. But the basic idea you want to remember next time you’re at the grocery store check-out is simple. A world beyond your perception — the nanoworld of atoms — is incredibly different from the one you live in. Somehow, we humans have peered into that tiny realm and come back with a deep enough understanding to reshape the macroworld we inhabit.