The Smallest Black Hole in the Universe
And is there a limit to how small they can possibly be?
“They had discovered one could grow as hungry for light as for food.”
-Stephen King
When you turn your gaze skyward and probe the depths of the night sky, you’re instantly reminded that there’s an entire Universe out there full of wonders. But in addition to the meteors, planets, stars, nebulae and galaxies out there that illuminate the Universe, there are also forms of matter out there that are completely invisible to our eyes.
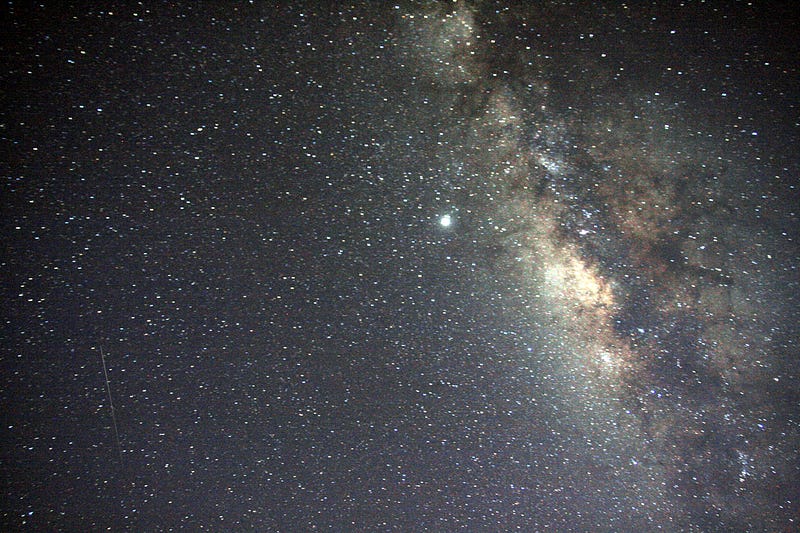
I’m not talking about the cold gas and dust out there that we can’t see in visible light, either. You see, those things are made out of the same building blocks — protons, neutrons and electrons — that we are. Even though they might not emit (and in fact, even absorb) visible light, if we look at the right wavelengths, we’ll be able to see them, too.
When we point our greatest observatories at the “dark” dust lane, above, that’s located towards our galactic center, this is what we see.
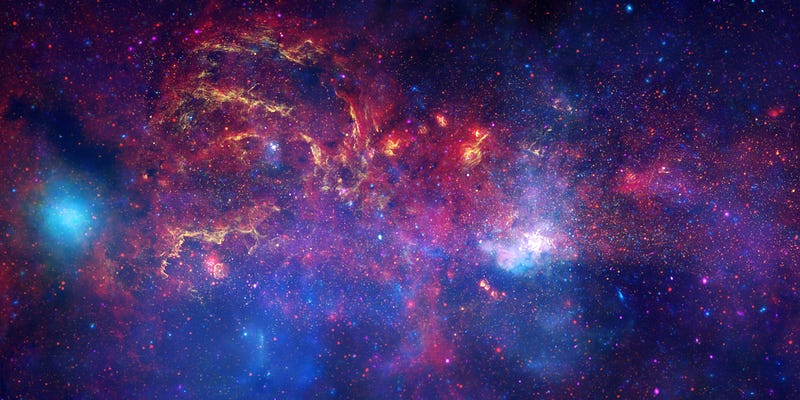
And yet, even if we’re only talking about the normal matter out there — the stuff that makes up stars, planets, gas, dust, and you and me — there are still sources out there that don’t emit any sort of light in any wavelength whatsoever. In fact, they can’t, because by definition, nothing can escape from them.
I’m talking, of course, about black holes.
We know that these objects exist not only theoretically, but observationally, too. In fact, just by looking at the central region of our galaxy, we can follow the orbits of stars, and find that they’re all orbiting a central mass that’s some four million times as massive as our Sun, yet emits no light.
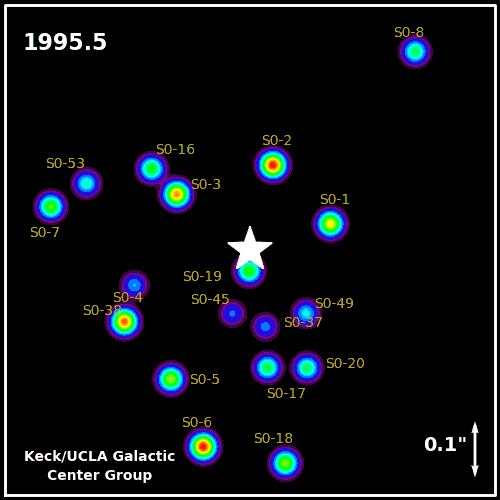
In fact, the center of the majority of galaxies contain supermassive black holes, many of them over a thousand times as large as the monster at the Milky Way’s center. These are among the largest black holes in the Universe, and are thought to form from the merging and devouring of millions of ancient corpses of dead, massive stars.
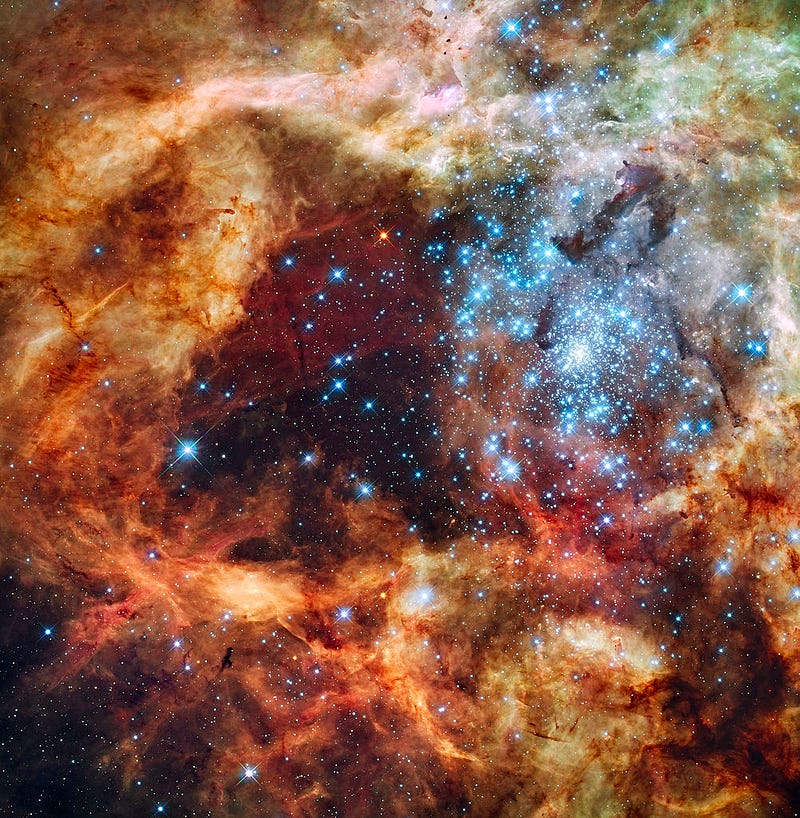
The largest, brightest, most massive stars are of course easier to see when you look at a young star cluster. You might think, in fact, that because they’re so much larger, they’ll live longer, having all that extra fuel to burn, but in fact the opposite is true!
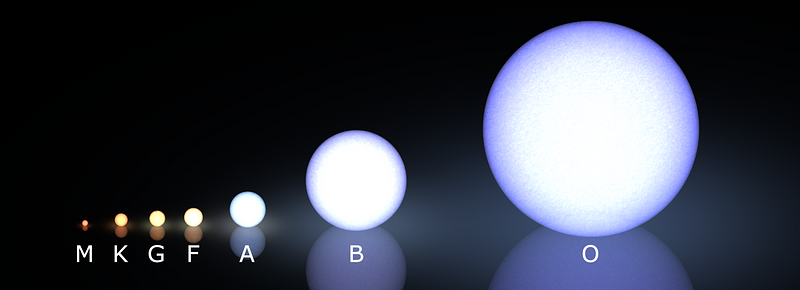
The most massive stars — O and B class stars — are literally tens of thousands of times brighter than a star like our Sun, due to the fact that they burn up their fuel tens of thousands of times as quickly. Even though they may be tens or even hundreds of times as massive as our Sun, they burn through their fuel so fast that their lifetime may be only a few million (or as short as a few hundred thousand) years! And when the most massive stars die, they don’t just die in a catastrophic supernova explosion…
but the star’s core also collapses, leaving either a neutron star or a black hole behind!
In general, the force of gravity works to compress a star, pulling it inwards and trying to get it to collapse. When nuclear fusion takes place inside the core of a star, that outward radiation pressure can balance the inward gravitational force, holding it up. Even when nuclear fusion runs out, matter is sturdy stuff, and atoms do an outstanding job of resisting collapse. In a star like our Sun (or even one four times as massive), when nuclear fusion comes to an end, the core of our star will shrink to about the size of Earth but no farther, as the atoms will reach a point where they refuse to budge any further.
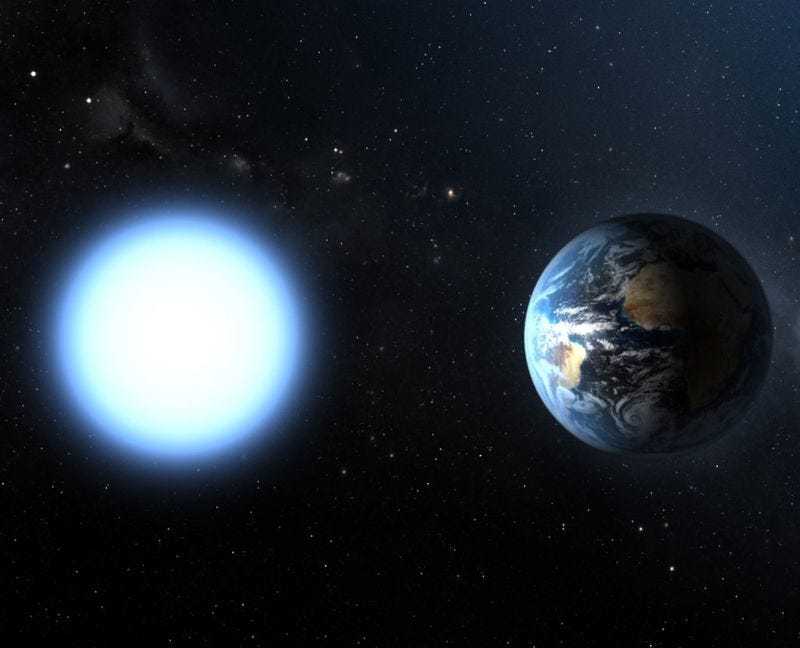
This pressure comes from the fact that quantum particles require more force to be compressed than even a Sun’s worth of gravity is capable of exerting. A star, however, that’s more than 400% the mass of ours will go supernova, and its central region will collapse down past the stage of atoms, collapsing down to a core of pure neutrons! Instead of being around the size of Earth, a neutron star is approximately a solar mass’ worth of neutrons in a sphere just a few kilometers in diameter.
Even though only a fraction of the original star remains in the core, neutron stars can range in mass anywhere from about the equal of our Sun all the way up to about three times as massive. But at masses beyond that, even the neutrons succumb to the force of gravity, and get compressed down to a size so small that light cannot escape from it. At that stage, we’ve progressed from a neutron star to a black hole!
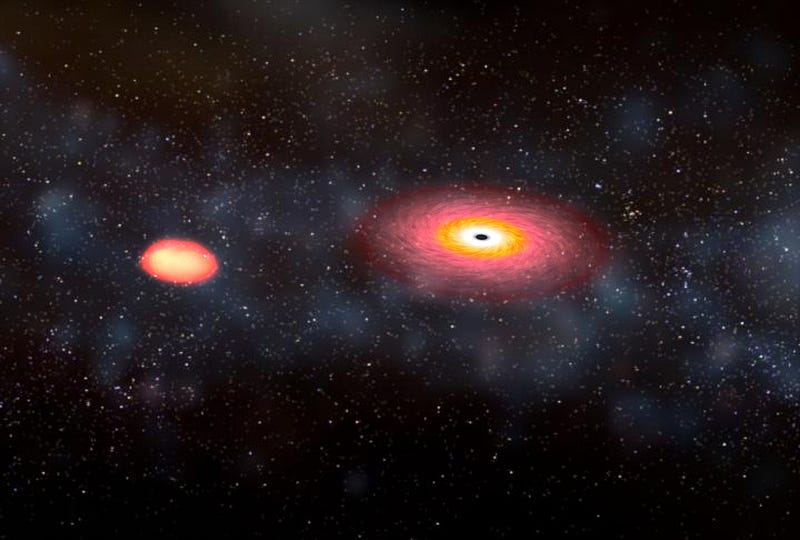
So what is the smallest known black hole out there? Right now, there are three candidates, some of which are more certain than others.
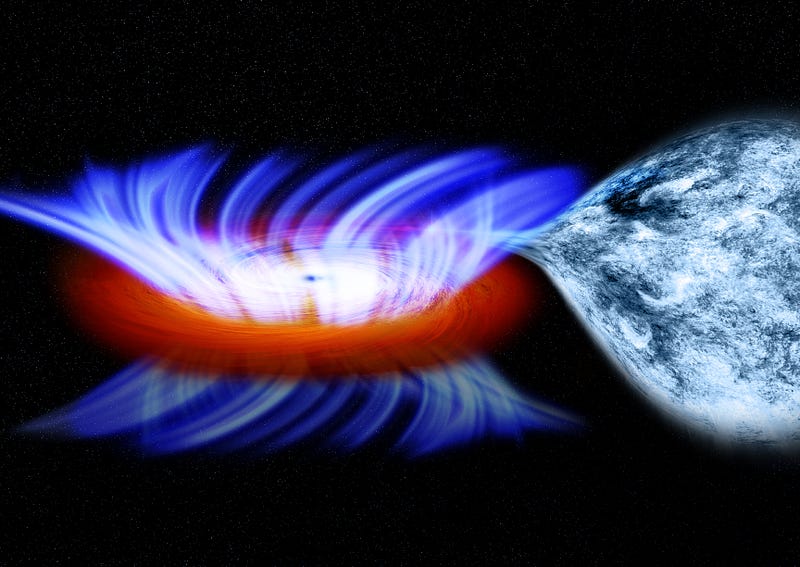
- IGR J17091-3624: A black hole in a binary system, which we can detect because of the intense stellar winds being generated by the binary-black hole system! Rather than matter falling into the black hole, approximately 95% of what’s being siphoned off of the companion star is getting ejected back into the interstellar medium. This is a low-mass black hole to be certain, but the mass is only pinpointed to be between three and ten times the mass of our Sun.
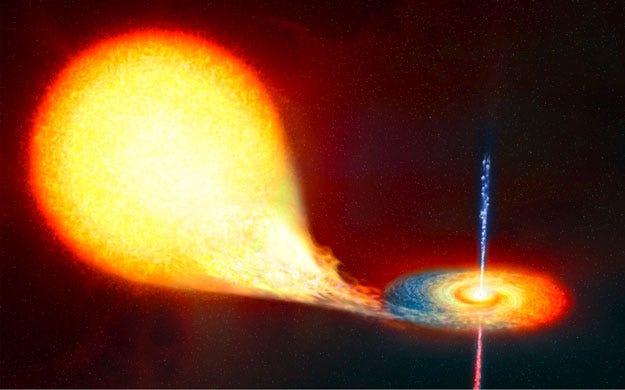
- GRO J0422+32: Another flickering, binary system, this one is located only 8,000 light-years from Earth, and the mass estimates vary wildly. Some teams claim this is a neutron star, with a mass of only 2.2 times the mass of our Sun; others claim it’s closer to four times the mass of our Sun while still others claim it’s as large as 10 solar masses. The jury’s still out, no doubt, but if I had to place my bets on the smallest known black hole, it’d be this next candidate…
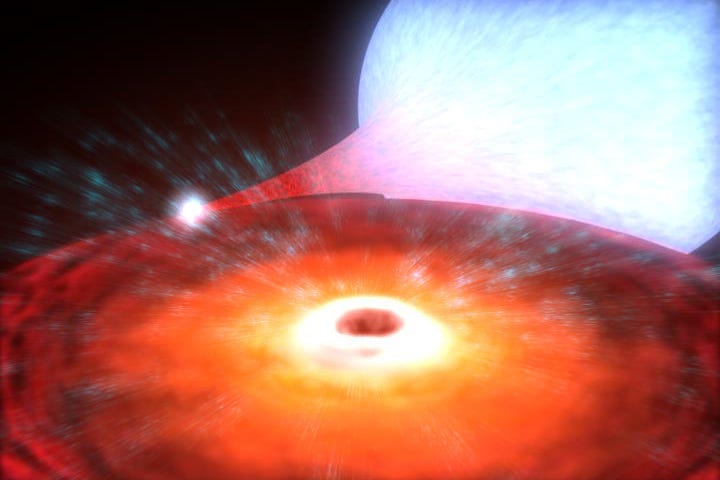
- XTE J1650-500: Originally announced to be only 3.8 Solar masses, estimates have since been revised to be more like 5 times the mass of our Sun. This binary system reliably emits X-rays from its accretion disk, and as we learn more and more about this class of objects, we’re uncovering relationships between the radiation emitted from outside of them and the mass of the black hole inside!
Wherever that cutoff between a neutron star and a black hole lies — whether it’s 2.5 or 2.7 or 3.0 or 3.2 solar masses — that’s where you might think the minimum mass black hole could possibly come from. But there are actually three other possibilities that we might yet discover!
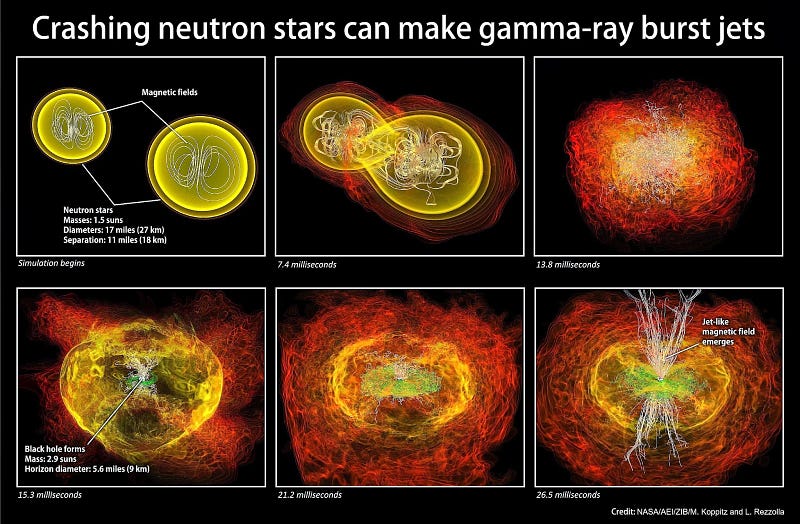
1.) Neutron star-neutron star mergers! This is the very process that produces the majority of certain very heavy elements such as gold in the Universe, and it does so by colliding two neutron stars together. Neutron stars are far more common than black holes, and although their collisions are relatively rare, happening once every 10,000-to-100,000 years or so in a galaxy, you must remember that the Universe is over 10 billion years old and contains nearly a trillion galaxies!
It’s quite conceivable that when two neutron stars collide, even if their mass wouldn’t have quite crossed the form-a-black-hole threshold on their own, the resultant process could create a black hole below the form-from-a-supernova mass. So there are good hopes for finding a two-point-something solar mass black hole just within our galaxy, which has likely seen around 100,000-to-1,000,000 of these events so far!
But let’s say you weren’t satisfied with what was around today, and wanted your black holes to be even smaller. Well, good news for the patient: all you have to do is wait!
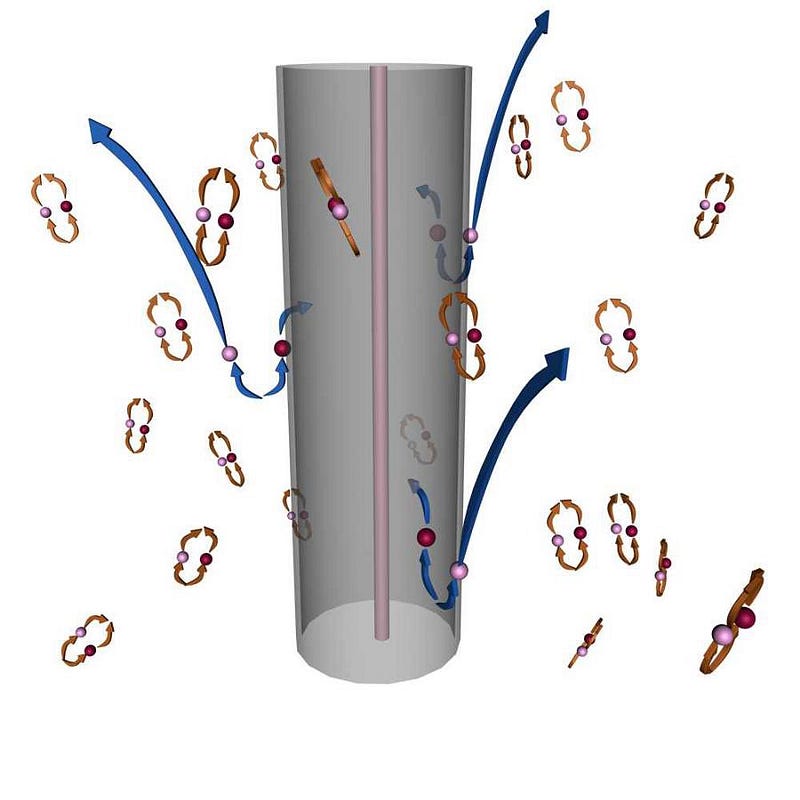
2.) Black holes lose mass over time! Because of the fact that the Universe is quantum in nature, producing particle-antiparticle fluctuations all the time both inside, outside and on the event horizon of black holes, these objects aren’t completely static in time. Although it happens very slowly, black holes evaporate thanks to a process known as Hawking radiation!
This isn’t a stream of particles and/or antiparticles that emanate from black holes, but rather some very low-energy, almost constant flux of blackbody radiation.
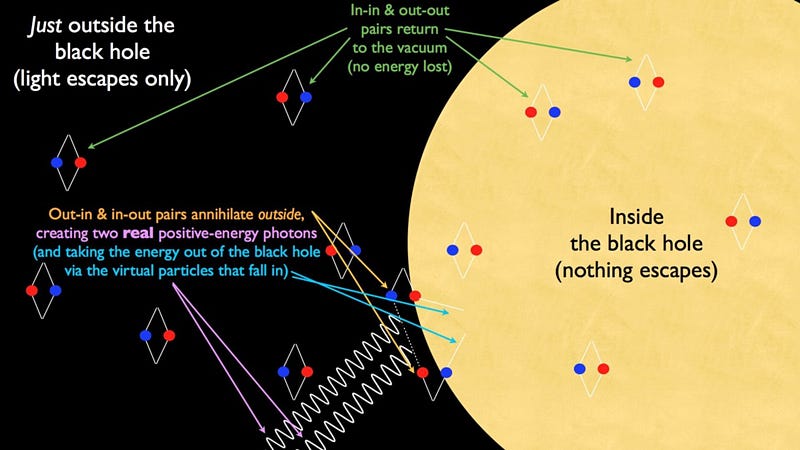
Over huge timescales — something like 10^68 or 10^69 years — these lowest mass black holes will evaporate, decreasing in mass slowly at first and then incredibly rapidly, losing the last few tonnes in just microseconds!
So if you want to see an even smaller black hole than what we’ve got in the Universe today, stick around for a while. And if you want them to be smaller, now, well, I’ve got bad news for you.
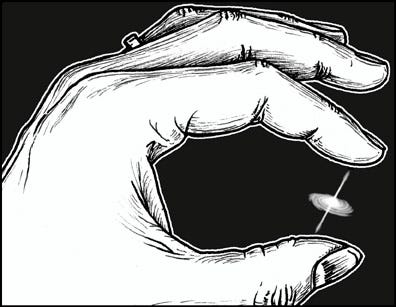
3.) The Universe could have been born with micro-black-holes, but it wasn’t. The idea of primordial black holes goes all the way back to the 1970s, and it’s kind of brilliant. You see, the Universe was once in a hot, dense, uniform, rapidly expanding state. If you had a region back then that was only 68% more dense than average, that region would automatically collapse to a black hole, and if you had many, small regions just like that, we could have had a Universe born full of micro-black-holes.
But we’ve measured what the magnitude of the density fluctuations in the very early Universe were, and how they change with scale as you look from the largest scales down to the smallest measurable ones.
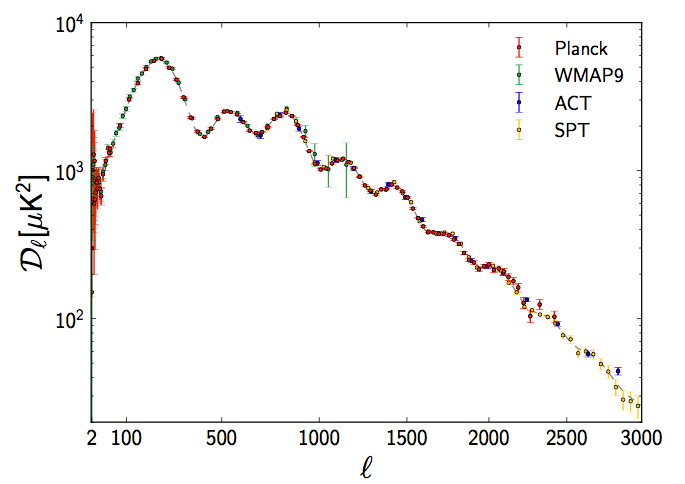
Rather than being 68% greater than average, typical fluctuations are only on the order of 0.003% greater, not nearly enough to have a Universe with even one primordial black hole in it. What’s worse, is that as you go to smaller and smaller scales — which is what you’d need to have a micro-black-hole — the fluctuations ever-so-slightly get smaller, making this a virtual impossibility. If things were different the Universe could have been full of them; that just isn’t our Universe.
And that’s the story of the smallest black holes in the Universe, from the ones we know to the ones we just haven’t found yet to the ones we simply need to wait for!
Enjoyed this? Leave a comment at the Starts With A Bang forum on Scienceblogs!