The Milky Way’s stars are gradually being ejected
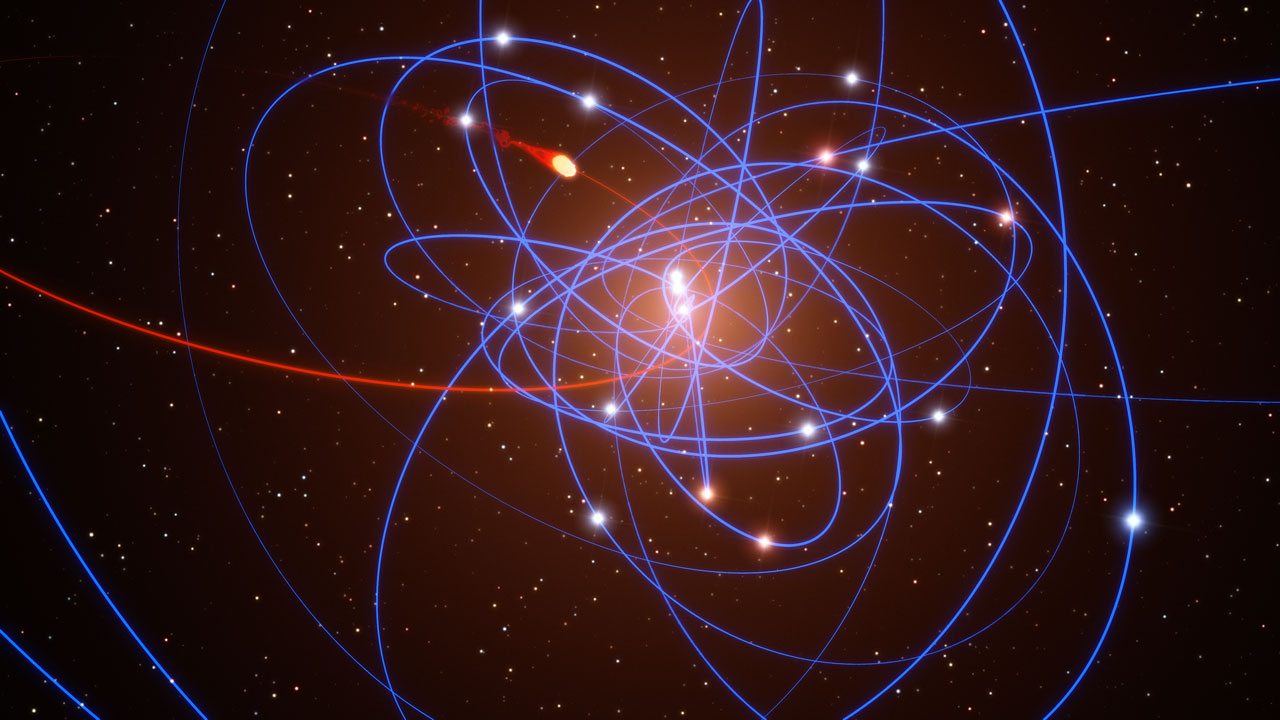
- Within our Milky Way galaxy, there are an estimated 200-400 billion stars, largely concentrated in the galactic disk and our central bulge.
- Although clouds of gas fall into our galaxy and new stars routinely form in the galactic plane, these stars don’t just die, but many are constantly getting kicked and ejected: far more than early estimates predicted.
- Recent data, particularly from the ESA’s Gaia mission, have taught us that these hypervelocity stars are far more common than we realized. It holds dire implications for the ultimate fate of our galaxy, as well as all the others.
Whenever we look up at the great expanse of the night sky, it’s easy to forget, from a cosmic perspective, just how confined our views are to our own backyard. The brightest objects of all are the Sun, Moon, and planets like Venus and Jupiter: objects right here in the Solar System. The glittering canopy of a dark night sky contains thousands of stars visible to the human eye: all of which are within our Milky Way, and most of which are within a few thousand light-years. Even the deep-sky objects, like nebulae and galaxies, are all contained within our Local Group. Despite the tens of billions of light-years that the expanse of the observable Universe stretches out for, nearly all of what’s most easily visible to us is right here in our local cosmic neighborhood.
But the grandest sight of all is the expanse of the Milky Way itself, stretching across the entire night sky. Within it, streams of light-blocking neutral matter stand in the way of hundreds of billions of stars, creating a diffuse glow that — with powerful enough observatories — can be resolved into individual points of light. It was once thought that these stars were all relatively stable in orbit around the Milky Way: like the planets within our Solar System. However, some revolutionary scientific discoveries have happened since that outdated picture held sway, and we now know that some of these stars have already received kicks that will soon eject them from the galaxy entirely. By investigating further, we can learn something about the Milky Way and, in turn, all galaxies: after a finite amount of time, they’ll dissociate, rather than being devoured by their central black holes. Here’s the story of stellar ejection.
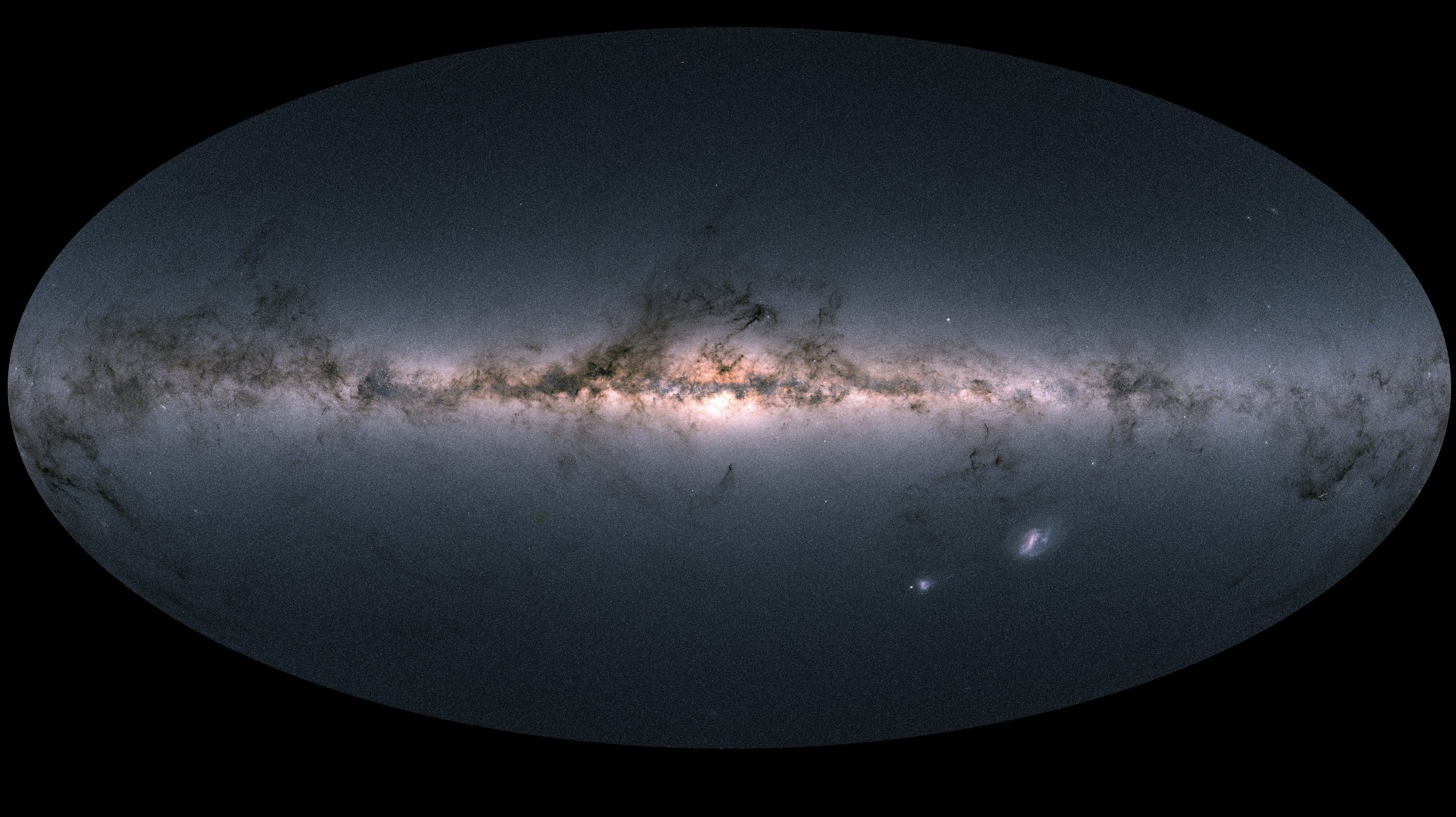
Stars within the galaxy
Simply by observing our galaxy with powerful, high-resolution telescopes, we can learn all sorts of facts about it. These include:
- how many stars there are, total,
- what the populations of different types of stars are,
- how massive and large our galaxy is,
- how the stars are distributed within it,
- and how quickly these stars are moving over time.
While the first set of facts about the stars within the galaxy can be gleaned simply by “snapshot” observations from a single moment in time, the last point — about how quickly these stars are moving over time — requires multiple observations over at least months and, ideally, years.
By observing the same star over the course of a calendar year, as Earth orbits around the Sun, we can conduct what are known as parallax measurements: measurements of how a star’s apparent position changes as Earth moves around the Sun and the Sun moves around the galaxy. Because we know our own motion, these types of measurements allow us to learn how far away each star is. Just as, by holding our thumb at arm’s length and switching which eye we view it through, we can determine how far away our thumb is by measuring how its apparent position shifts relative to the background, we can determine how far away the closest stars are as our perspective, from Earth, shifts over the course of a year.

But we can then go a step further, which wide-field observatories such as ESA’s Gaia and China’s ground-based LAMOST telescope enable, and measure how each of these stars is moving in three-dimensional space: along the line-of-sight from the redshift or blueshift it displays, plus in the transverse direction (up-down and left-right across the sky) from precise long-term observations.
For almost all cases, we learn that the stars within the Milky Way are orbiting around it in much the same way that the Sun orbits around our galaxy: in the same general direction, at roughly the same speed (around 220 km/s), with most stars remaining largely confined to the galactic plane. Most of the stars in our vicinity are moving relatively slowly compared to the Sun: at speeds of just 10-20 km/s relative to our parent star, or even slower than Earth moves in its orbit around the Sun.
However, there are a few weirdos: stars that are moving much, much faster than that. These so-called hypervelocity stars can be found moving at several hundreds of kilometers-per-second relative to the speeds of the other stars around them. First predicted back in 1988 by Jack Hills, they were originally thought to arise from binary star systems that experienced close encounters with a supermassive black hole. However, a closer examination of the birthplaces of stars revealed two other, more frequent physical causes behind this phenomenon.
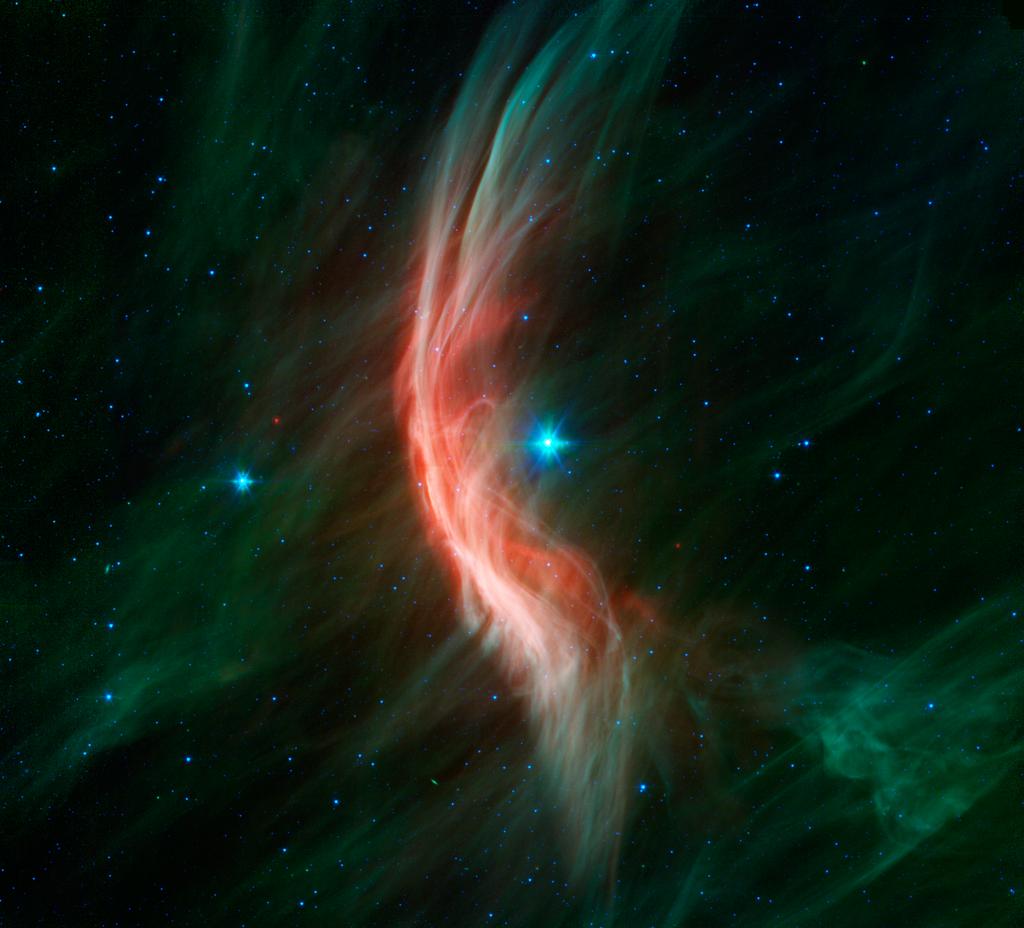
The death of the birthplaces of stars
The first place you might think to look for a fast-moving star is within either an open star cluster or a globular cluster of stars: locations that are not too far away (i.e., within our own Milky Way) where hundreds, thousands, or even millions of stars are all collected within a volume just a few light-years in radius. Within these star clusters, only about half of the stars inside are single star systems, with the rest being in binary, trinary, or star systems with four or more stars inside. Whereas the gravitational interaction between two passing stars simply just scatters, or redirects, them, the gravitational interaction between many bodies leads to some fascinating — and violent — phenomena.
One of these phenomena is known as violent relaxation. In a system with many comparably large masses all gravitationally bound together, interactions between multiple bodies within that system cause:
- the heaviest-mass objects to sink to the center,
- the lighter, lower-mass objects to “puff up” to higher-radius orbits,
- and some of these low-mass objects will be ejected, or kicked out, with extremely large velocities.
This is consistent with what we see for open star clusters within our galaxy: the sites of where new stars are born in the plane of the Milky Way. The youngest star clusters may contain up to several thousand stars, but over the span of just a few hundred million years, they typically dissociate completely, ejecting and unbinding all of the stars within them. With only a few rare exceptions, few open star clusters even make it to an age of one billion years before being completely gravitationally torn apart.
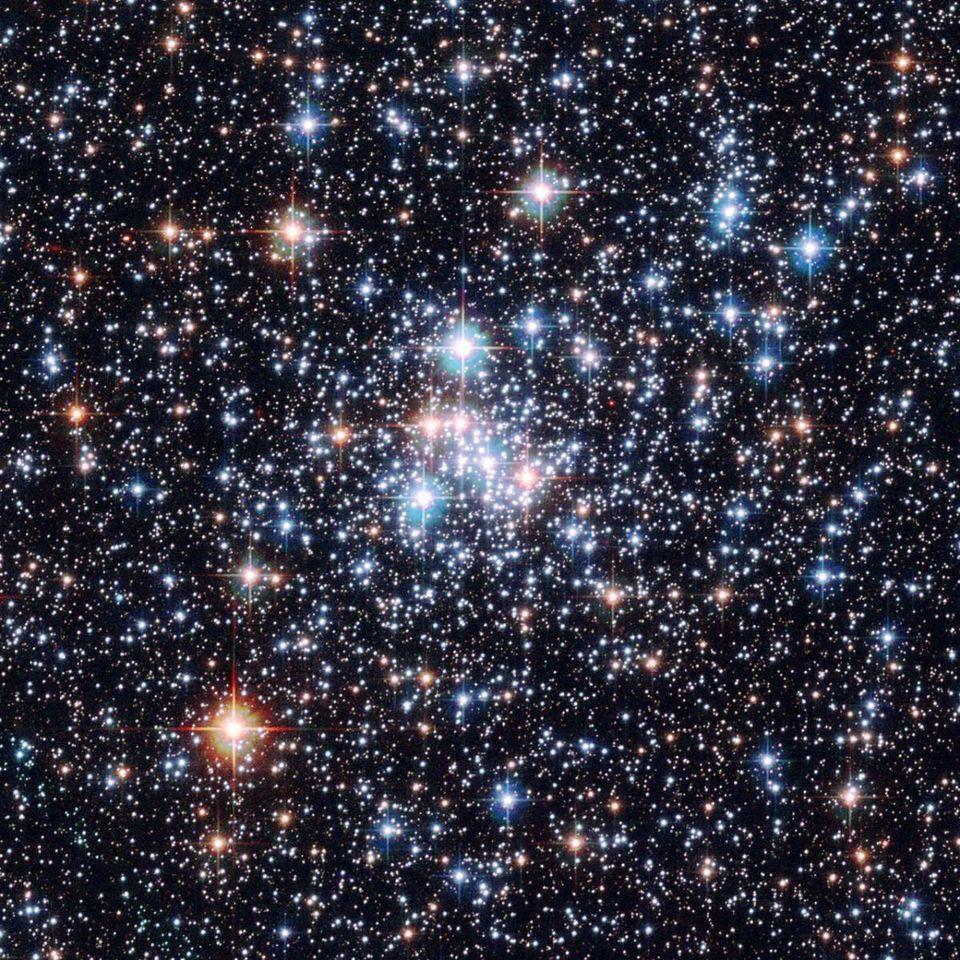
Hypervelocity stars
When clusters are torn apart, most of the stars that were once within it do get ejected, but only at speeds of tens of kilometers-per-second. However, beginning in 2005, astronomers have discovered a few stars that have been observed to have not only larger velocities in the range of hundreds of kilometers-per-second, but stars that are moving so fast they are destined to escape from the Milky Way’s gravity, the same way that a fast-enough moving rocket ship can escape from Earth’s gravitational pull. It’s as though something other than the typical gravitational interactions between objects in a gravitationally bound cluster is behind these objects: hypervelocity stars.
There are two main ways to energetically “kick” a star to great velocities, assuming it originated with a more typical set of parameters for a star within our galaxy.
- A cataclysmic event, such as a supernova, kilonova, or gravitational wave-driven merger, occurred in a system where another star was also present, and this star received a kick from this cataclysm.
- Or a star experiences a close encounter with a binary (or richer) system, where one of the members is a compact, high-mass object like a black hole, which can impart a high-velocity kick at the expense of leaving the remaining members more tightly bound.
Both of these scenarios are plausible, but which one — if either — explains the hypervelocity stars that we see?

Cataclysmic kicks versus the three-body problem
One way to try to discover the cause of hypervelocity stars is to take a census of the ones that we find, and to try to trace these stars back to a possible point of origin. When the first hypervelocity stars were found, Harvard astronomer Warren Brown (who first discovered them) initially sought to blame the supermassive black hole at the center of our galaxy, just as Jack Hills theorized back in 1988. As Brown noted years ago,
“It’s paradoxical, but a black hole doesn’t always suck in whatever gets close. In rare circumstances, a star that’s part of a pair can be ripped from its partner and launched through space at an amazing speed.”
Although this was the initial idea, it resulted in an extremely low prediction for the rate of hypervelocity stars expected: about 1 star ejected from the central area of the Milky Way with every 100,000 years that passes. Back when we only knew about a total of 10 or so hypervelocity stars, this was perhaps a reasonable explanation, even though it was biased by a search area that was confined to the region around the galactic center.
However, there are many, many more stellar mass black holes out there: hundreds of millions or possibly even a number in the low billions in the Milky Way alone, all of which experience gravitational encounters with passing star systems. Many of them were created through supernovae and/or the merger of compact objects, like neutron stars or other black holes, previously. Both cataclysms and three-body (or many-body) interactions could lead to far greater numbers of these hypervelocity stars than were initially expected.

The stars already on their way out
The first hypervelocity star was found just in 2005, but by the end of 2020, the number of known hypervelocity stars in the Milky Way had grown to more than 1000. In particular, by combining data from the ESA’s Gaia mission, which collected three-dimensional distance and motion data for more than 1 billion stars within the Milky Way, and from China’s LAMOST survey, which collected spectroscopic data for selected stars and star systems of interest, a whopping 591 new hypervelocity stars were discovered in 2020 alone: all in the galactic halo.
What’s remarkable about finding stars in the galactic halo is that you can trace their trajectories backward and see if they plausibly originate from either:
- the center of the Milky Way,
- a non-central region of the Milky Way’s disk,
- or a location that indicates they came from somewhere other than the Milky Way.
Remarkably, the idea that all of the hypervelocity stars would come from the galactic center was immediately and severely refuted by the new data. As it turned out, only 15% of the stars are on paths that can be traced back toward or near the center of the Milky Way, whereas 85% of the known hypervelocity stars originate from elsewhere.
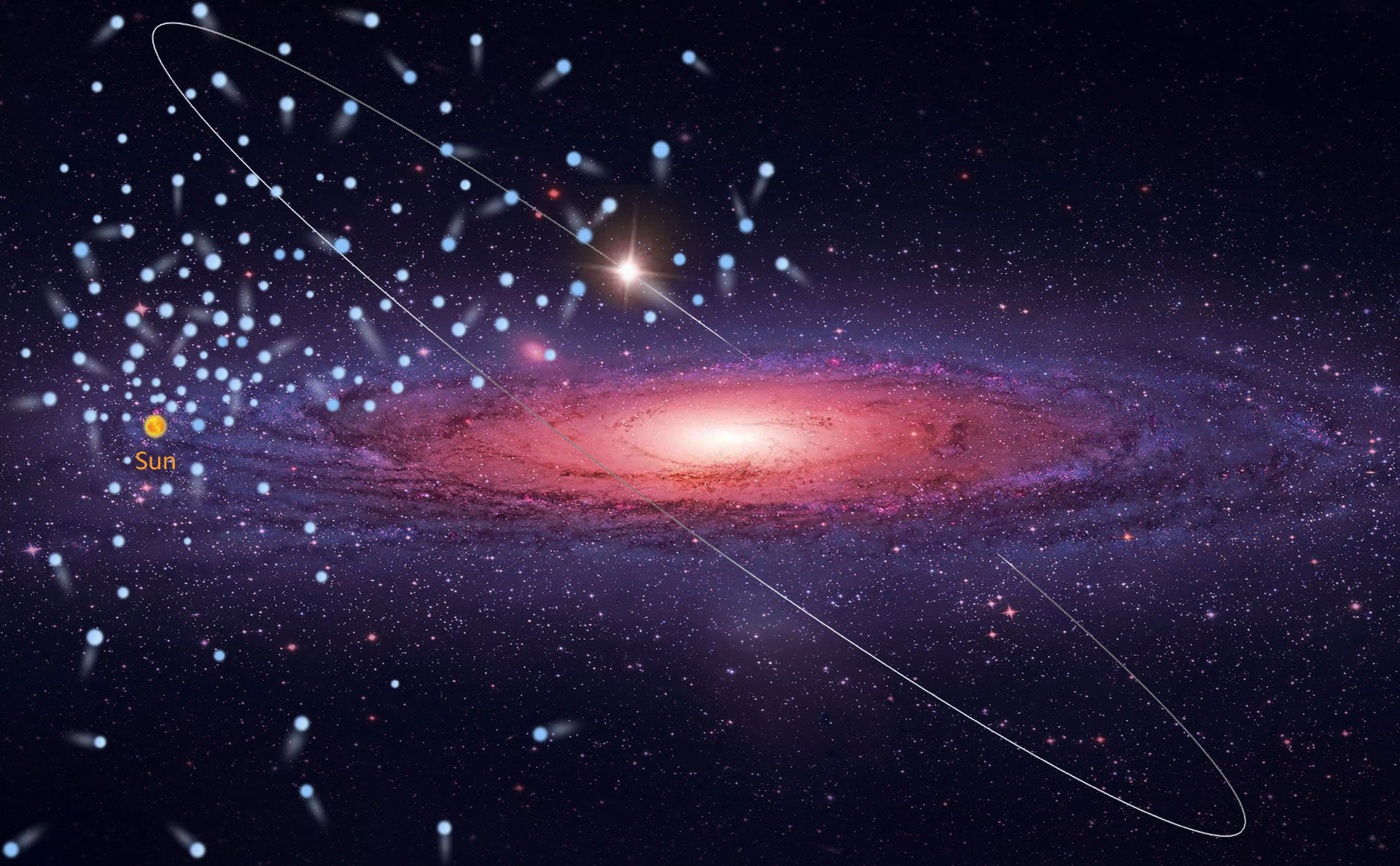
Given the fact that we exist within the Milky Way and are looking at stars that are relatively close by (within the Milky Way’s halo), you might expect that nearly all of the other 85% of hypervelocity stars would originate from somewhere else within the Milky Way’s disk: where stars, black holes, and stellar cataclysms are common. But that’s not what the data revealed. Instead, 55% of hypervelocity stars originated from the galactic disk, but a remarkable 30% appeared to have extragalactic origins: as though they came from a different galaxy than the Milky Way and just happen to be passing through our galaxy’s location right now.
This has severe implications for the abundance of hypervelocity stars.
If we’ve cataloged approximately 1 billion stars within the Milky Way, and approximately 1000 of them are observed to be hypervelocity stars — moving at speeds of hundreds of km/s (or, in some rare and spectacular cases, 1000+ km/s) — then it means that approximately 1-in-a-million stars, or 0.0001% of stars, are presently on their way out of the galaxies that they currently occupy. (And we have to recognize that even this is a biased sample; the majority of known hypervelocity stars are bright, giant-type stars.) This is something that’s come to pass in a Universe that’s “only” about 13.8 billion years old. As time continues to pass, astrophysical cataclysms will continue to occur, as will gravitational interactions with other stars, stellar systems, and black holes. And since those mechanisms are responsible for most hypervelocity stars, rather than interactions with the black hole at the galactic center, we can begin to say something meaningful about how long stars and stellar remnants can actually persist within the Milky Way.
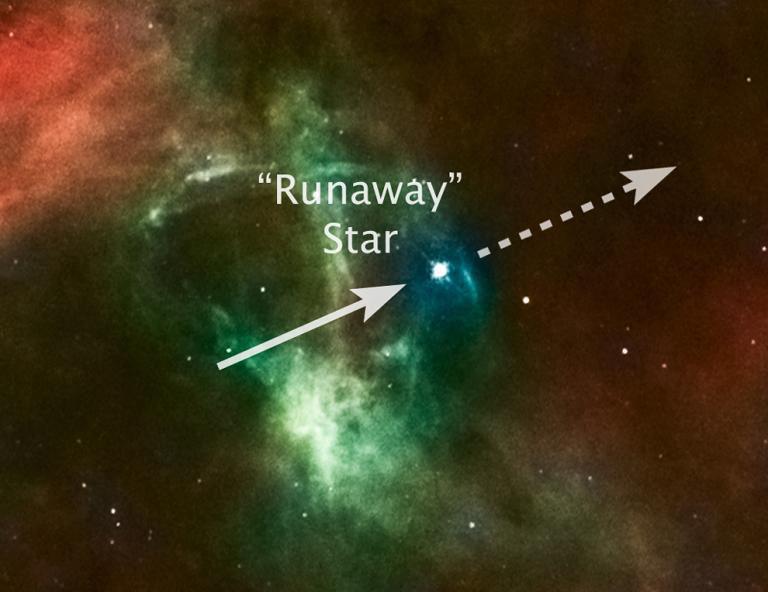
Just a matter of time
No matter how old “13.8 billion years” sounds for the age of the Universe, we have to recognize that our galaxy and our Local Group are both completely gravitationally bound. Other than the rare star, black hole, or substellar object that gets kicked to speeds above escape velocity within such a structure, the material that’s inside a gravitationally bound structure will remain inside that gravitationally bound structure. With an estimated ~200-400 billion stars within the Milky Way alone, you might be tempted to scoff at the idea that the types of interactions, either through cataclysms or multi-body gravitational interactions, could ever kick all of the stars, including whatever stellar remnants remain at the end of a star’s life, out of a galaxy like the Milky Way.
But this is, in a cosmic sense, just a matter of time. Even though the space separating the stars is enormous — after all, it’s more than 4 light-years from us to the nearest star — the sheer number of stars within each galaxy, the rapid speeds at which they move, and the virtually limitless amount of time we have to consider, ensures that eventually, one of these two types of interactions is likely to befall each and every star once enough time has passed. When the Universe is ten, a hundred, or a thousand times its present age, we can expect tens, hundreds, or thousands of times as many stars to be ejected versus (roughly) the number of stars that have been ejected, or kicked to hypervelocity speeds, thus far.

Conservatively, that means that when the Universe has reached an age of approximately 1017 years, or around a million times its present age, most galaxies will have already ejected most of their stars and stellar remnants. Over even longer periods of time, perhaps once ~1019 years have passed, there will be very few stellar remnants left inside any galaxy at all, as nearly all of these massive clumps of matter will have been hurled into intergalactic space by cataclysmic kicks and multi-body gravitational interactions. Yes, the supermassive black holes at the centers of galaxies will persist, but aside from a few lucky objects that weren’t ejected, little else should remain.
You have to remember that, given enough time, even extremely unlikely processes are bound to occur. As we’ve learned from studying, finding, and investigating hypervelocity stars as a class, they are both more common than initially thought and also largely originate from different locations (and different mechanisms) than we surmised at first. Even though cataclysms are rare, they happen, and they sometimes happen when other stars (or sub-stellar objects, or stellar remnants) are nearby. Even though multi-body gravitational interactions are uncommon, they’re going to eventually affect nearly every mass if we wait for long enough. When we think about the far future of our Universe, we don’t normally think about galaxies as “emptying out” their contents over time, but that’s precisely what studying hypervelocity stars seems to be teaching us. The stars in our galaxy won’t just all eventually die, they’ll eventually leave home as well: destined to wander the abyss of intergalactic space for eternity.