I’m a chemist, and I’m building a universal robot to make life and find aliens
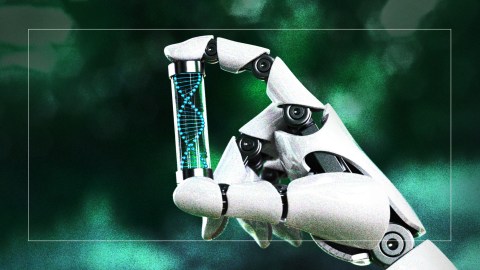
- Life is a process that directs the assembly of complex systems by assembling “memories.”
- This is the fundamental insight behind our search for the origin of life and life on other planets — only living organisms can produce complex molecules in a great abundance.
- Our lab is constructing chemistry-performing computers (“chemputers”) to synthesize any molecule from computer code. This is the first step toward solving the mystery of how life emerged from inorganic matter.
What is life? Scientists still cannot agree on an answer. Many suggest that life requires a metabolism, genetic material, and the ability to self-replicate, but there the possibility of broad agreement ends. Are viruses alive? What about a storm or a flame? Even worse, the driving force that leads to the emergence of life still eludes us.
Since the time of Darwin, scientists have struggled to reconcile the evolution of biological forms in a universe determined by fixed laws. These laws underpin the origin of life, evolution, human culture, and technology, as set by the boundary conditions of the universe. However, these laws cannot predict the emergence of these things.
Evolutionary theory works in the opposite direction, indicating how selection can explain why some things exist and not other things. To understand how open-ended forms can emerge in a forward-process from physics that does not include their design, a new approach to understand the transition from the non-biological to the biological is necessary.
One unique property of living systems is the existence of complex architectures that cannot form by chance. These architectures can exist over billions of years, resisting environmental decay. How is this achieved? Selection is the answer: It is the force that creates life in the universe via the emergence of evolutionary systems. Selection came before evolution.
Imagine you are a climber scaling a vertical rock face with a ladder, building it one rung at a time. The raw material for the ladder parts is being randomly “produced” and thrown at you. If the materials arrive too fast, you cannot catch the materials, and you will eventually die. If the materials arrive too slowly, you won’t be able to get to the top, and once again you will die. If the materials are coming at just the right pace, though, the “production” time and the “discovery” time for the parts will be balanced so that selection can happen.
The formation of these ladders must happen at the molecular level for selection to occur, yet causation is not accepted by physics as a fundamentally occurring process. Rather, causation emerges in complex systems. But where do these complex systems come from to help causation emerge?
“Assembly Theory” and the hallmark of life
A few years ago, we realized that it was possible to tell the difference between complex molecules and simple molecules by the number of steps needed to construct the molecule from a lineage of parts. The larger the number of parts required, the more complex the molecule. We call the shortest path for assembling a molecule its “assembly index.” The assembly index literally tells us the minimum amount of memory the universe must have to remember how to create that object as quickly and simply as possible.
We then realized that this observation led to a much deeper framework that we call “Assembly Theory,” which, simply put, helps explain why anything exists at all. This is because the assembly index allows ordering in time, which in turn explains why some objects exist before others: It is due to constraints in the pathway that leads to the object in question. In other words, if A is simpler than B, and B is simpler than C, both A and B need to exist before C exists.
How does this translate into a firm idea of how to find life? Assembly Theory allows us to identify objects that are both complex (that is, with a high assembly index) and form in such a high abundance that they could only be formed by life. The greater the abundance of objects with a high assembly index, the more improbable it is that the objects could be produced without a highly directed process requiring evolution. Therefore, Assembly Theory explains the mechanism or underlying framework from which selection drives the emergence of life itself.
Universal life detector
The quest to uncover the precise origin of life on Earth has been a great challenge for several reasons. One is that it is not possible to map the exact processes that gave rise to life at the level of atoms and molecules. Another is that the emergence of the specific life we find on Earth appears to be entirely contingent on the history of Earth, which cannot be fully reproduced in the laboratory.
However, this does not mean that the pursuit will forever elude science. I’m optimistic that we will be able to detect the origin of life in experiments in the laboratory on Earth, as well as find life elsewhere in the universe. We are hopeful that the plethora of exoplanets out there means that life is always going to be emerging somewhere in the universe — in the same way that stars are constantly dying and being born.
If we can shift our thinking to look for selection-producing collections of objects (like molecules analogous to the climber building the ladder) with high assembly indexes as the clear precursor to life, then our approach to finding life in the universe vastly expands. The objective now is to find complex objects with a shared causal history. We call this a “shared assembly space,” and it will help map interactions across the entire universe.
Another way to look for life in the universe is by designing experiments that allow us to look for the emergence of life in the laboratory. How might we do this? If life emerged over the course of 100 million years using the entire planet as a test tube or warm little pond, then how might we re-create such a massive experiment, and how would we know if we were successful? We must start with the universal life detector (ULD). The ULD will detect objects, systems, and trajectories that have high assembly indexes and, hence, are the products of selection.
“Chemputation” and searching chemical space
Answering big questions in science requires asking the right questions. I have long thought that the question of the origin of life should be framed as a search problem in “chemical space.” This means that a vast number of chemical reactions, starting from a set of simple input chemicals, needs to be explored over many reaction cycles and environments for the process of selection and causation to emerge over time.
For example, if a molecule is generated in a random soup, and that molecule can catalyze or cause its own formation, then the soup will be transformed from a collection of random molecules into a highly specific collection of molecules with multiple copies of each molecule. At the molecular level, the emergence of the self-replicating molecule can be seen as the simplest example of the emergence of “causal power” and is one of the mechanisms that allow selection to occur in the universe.
How can we search chemical space in a way that moves far beyond what computer simulations can accomplish? To do this, we need to build a series of modular robots that both understand and can perform chemistry. (A key challenge is that the physical architecture to do so does not exist yet, and most chemists think that the programmable control of chemical synthesis and reactions is impossible. However, I think it is possible. But proposing this idea is like suggesting the internet before computers existed.)
Around a decade ago, we asked if it was possible to build a universal chemical robot that could make any molecule. This seemed like an insurmountable problem, as chemistry is very messy and complex, and the instructions used to make molecules are often ambiguous or incomplete. As an analogy, compare this to the generalized abstraction of computation, in which the Turing machine can be used to run any computer program. Could a universal abstraction for chemistry be constructed — a type of chemical Turing machine?
To achieve this, we must consider the minimal “chemputing” architecture required to make any molecule. This is the key abstraction that allowed the concept of chemputation — the process of making any molecule from code in a chemputer — to be born. And the first working, programmable chemputer was built in 2018. Initially, chemputers were used to make known molecules, develop better routes of synthesis, and to discover new molecules.
The chemputer-mesh
We are aiming to design and build networks of chemputers, or a “chemputer-mesh,” dedicated to searching for the origin of life in my laboratory and across the world. All the chemputers in the mesh will use the same universal chemical programming language and aim to search chemical space for evidence of selection from very simple molecules. By designing an “assembly detector,” using the same principles as for the ULD but tailored for the laboratory, we aim to catch the driving force responsible for the origin of life in the act.
Compare this to the vast detectors at the Large Hadron Collider built to find the Higgs boson at high energies. Our assembly detector will look for complex molecules that have a high assembly index and are produced in large numbers from a soup of simple molecules. The next step will be to set up the chemputer-mesh to search the chemical universe to find those conditions from which life can emerge. If this is successful, and we can demonstrate how simply these conditions can emerge on Earth, we will be able to follow how evolution can start from the inorganic world — not just on our planet, but on all the exoplanets in the universe.