Why Cosmic Inflation’s Last Great Prediction May Fail
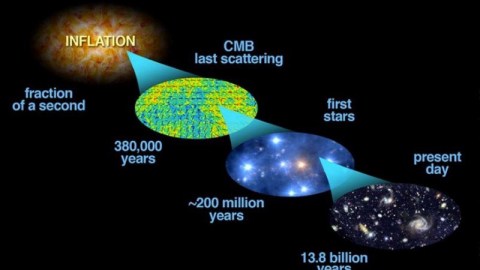
And what it means if we don’t see gravitational waves from inflation in the next 5–10 years.
“The paradigm of physics — with its interplay of data, theory and prediction — is the most powerful in science.” –Geoffrey West
One of the greatest scientific achievements of the early 20th century was the discovery of the expanding Universe: that as time goes on, distant galaxies are receding from us, as the space between us expands according to Einstein’s General Relativity. In the mid-20th century, a great idea was put forth, that if the Universe is getting bigger and cooler today, then it was smaller, hotter and denser in the past: the Big Bang. The Big Bang made a few extra predictions:
- there would be a great cosmic web of structure, with small, medium and large-scale structures clumped together in certain patterns,
- there would be a leftover glow of radiation from the early Universe, that’s cooled to just a few degrees above absolute zero,
- and there would be a specific set of ratios for the lightest elements in the Universe, for the different isotopes of hydrogen, helium and lithium.
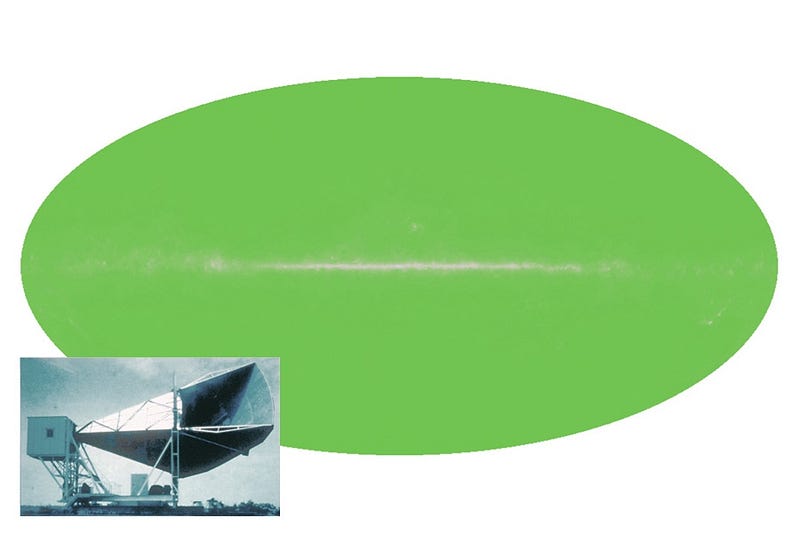
In the 1960s and 1970s, these predictions were all confirmed to varying degrees of accuracy, and the Big Bang became overwhelmingly accepted as the leading theory of where everything we can perceive and detect in the Universe originated. But there were a few questions that were unanswered when it came to the Big Bang, a few phenomena that were completely unexplained within this framework.
- Why was the Universe the exact same temperature everywhere?
- Why was the Universe so spatially flat; why did the expansion rate and the matter/energy density balance each other so perfectly well?
- If the Universe achieved such high energies early on, why haven’t we seen the stable relics that should be spread throughout the Universe from it?
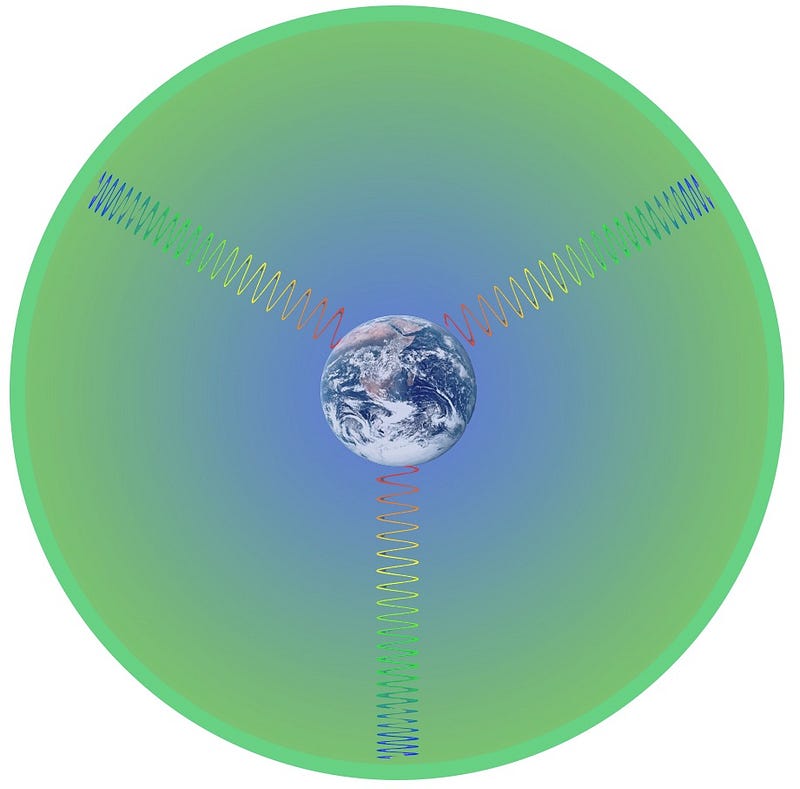
If the Universe were expanding according to the rules of General Relativity, there’s no reason to expect that regions of space separated by distances greater than the speed of light were connected, much less the same exact temperature. If you take the Big Bang all the way back to its logical conclusion — to an infinitely hot, dense state — there’s no way to come up with answers to these questions. You just have to say, “it was born this way,” and from a scientific point of view, that’s wholly dissatisfying.
But there’s another option. Perhaps, instead of the Universe just being born at the moment of the Big Bang with these conditions, there existed an early stage that set up these conditions and the hot, dense, expanding and cooling Universe that gave rise to us. This would be a job for theorists: to figure out what possible dynamics could set the stage for the Big Bang with these conditions to occur. In 1979/1980, Alan Guth put forth the revolutionary idea that would change the way we thought about our Universe’s origins: cosmic inflation.
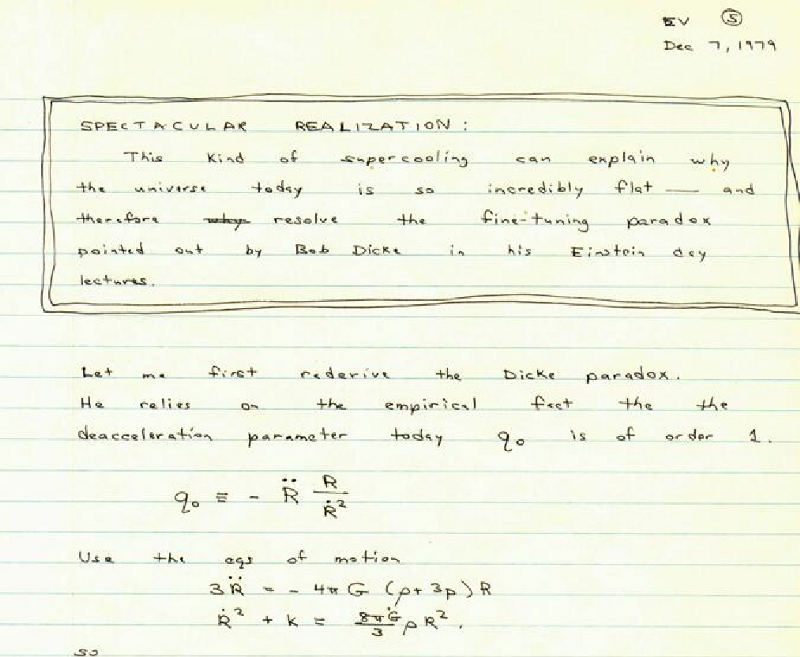
By postulating that the Big Bang was preceded by a state where the Universe wasn’t filled with matter-and-radiation, but rather by a huge amount of energy inherent to the fabric of space itself, Guth was able to solve all of these problems. In addition, as the 1980s progressed, further developments occurred that made it clear that, in order for inflationary models to reproduce the Universe we saw:
- to fill it with matter-and-radiation,
- to make the Universe isotropic (the same in all directions),
- to make the Universe homogeneous (the same in all locations),
- and to give it a hot, dense, expanding state,
there were quite a few classes of models that could do it, as developed by Andrei Linde , Paul Steinhardt, Andy Albrecht, with additional details worked out by people like Henry Tye, Bruce Allen, Alexei Starobinskii, Michael Turner, David Schramm, Rocky Kolb and others. But the simplest ones — the ones that solved the problem and had the fewest free parameters — fell into just two categories.
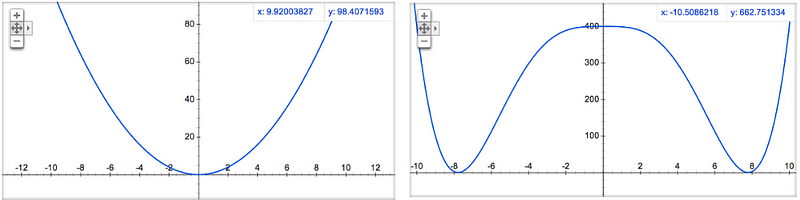
There was new inflation, where you had a potential that was very flat at the top and that the inflaton field could “roll down, slowly” to reach the bottom, and there was chaotic inflation, where you had a U-shaped potential that, again, you’d roll down slowly.
In both these cases, your space would expand exponentially, be stretched flat, have the same properties everywhere, and when inflation came to an end, you’d get back a Universe that very much resembled our own. In addition, you’d also get six extra, new predictions out, all of which had not yet been observed at the time.
- A Perfectly Flat Universe. Because inflation causes this rapid, exponential expansion, it takes whatever shape the Universe happened to be and stretches it to tremendous scales: to scales much, much larger than what we can observe. As a result, the part that we see looks indistinguishable from flat, the same way that the ground outside your window may look flat, but it’s actually part of the entire, curved Earth. We just can’t see enough to know what the true curvature actually is.
- A Universe with fluctuations on scales larger than light could’ve traveled across. Inflation — by causing the space of the Universe to expand exponentially — causes what happens on very small scales to get blown up to much larger ones. This includes quantum fluctuations, which normally fluctuate in-place in empty space. But during inflation, thanks to the rapid, exponential expansion, these small-scale energy fluctuations get stretched across the Universe onto gigantic, macroscopic scales that should wind up spanning the entire visible Universe!
- A Universe with a maximum temperature that’s not arbitrarily high. If we could take the Big Bang all the way back to arbitrarily high temperatures and densities, we’d find evidence that the Universe once reached at least the temperature scale at which the laws of physics break down: the Planck scale, or around energies of 10^19 GeV. But if inflation occurred, it must have occurred at energy scales lower than that, with the result that the maximum temperature of the Universe post-inflation must be some energy scale lower than 10^19 GeV.
- A Universe whose fluctuations were adiabatic, or of equal entropy everywhere. Fluctuations could have come in different types: adiabatic, isocurvature, or a mixture of the two. Inflation predicted that these fluctuations should have been 100% adiabatic, which means that detailed measurements of the types of quantum fluctuations the Universe started off with should reveal signatures in the microwave background and in large-scale cosmic structure.
- A Universe where the spectrum of fluctuations was just slightly less than having a scale invariant (n_s < 1) nature. This is a big one! Sure, inflation generically predicts that these fluctuations should be scale-invariant. But there’s a slight caveat, or a correction to that: the shape of the inflationary potentials that work — their slopes and concavities — affect how the spectrum of fluctuations departs from perfect scale invariance. The two simplest classes of inflationary models, new inflation and chaotic inflation, give predictions for n_s that typically cover the range between 0.92 and 0.98.
- And finally, a Universe with a particular spectrum of gravitational wave fluctuations. This is the last one, and the only major one that hasn’t yet been confirmed. Some models — like the simple chaotic inflation model — give large-magnitude gravitational waves (the kind that could’ve been seen by BICEP2), while others, like the simple new inflation model, can give very small-magnitude gravitational waves.
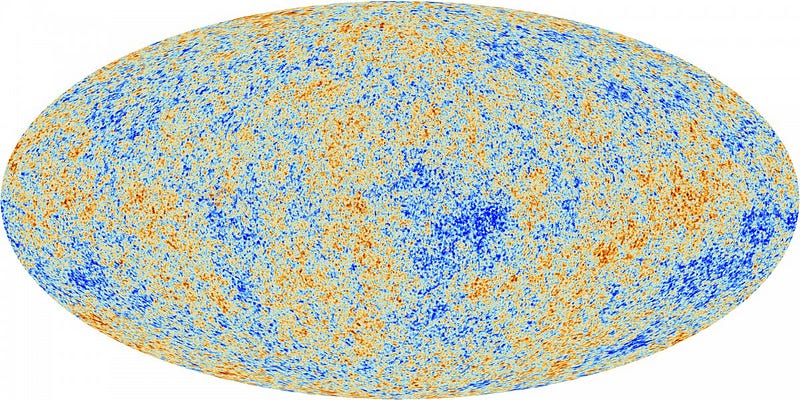
Over the past 35 years, we’ve made incredible, all-sky measurements of the fluctuations in the cosmic microwave background, from scales as large as the entire visible Universe down to angular resolutions of a mere 0.07°. As space-based satellites became more and more capable over time — COBE in the 1990s, WMAP in the 2000s, and now Planck in the 2010s — we’ve gained incredible insight into the Universe when it was less than 0.003% its current age.
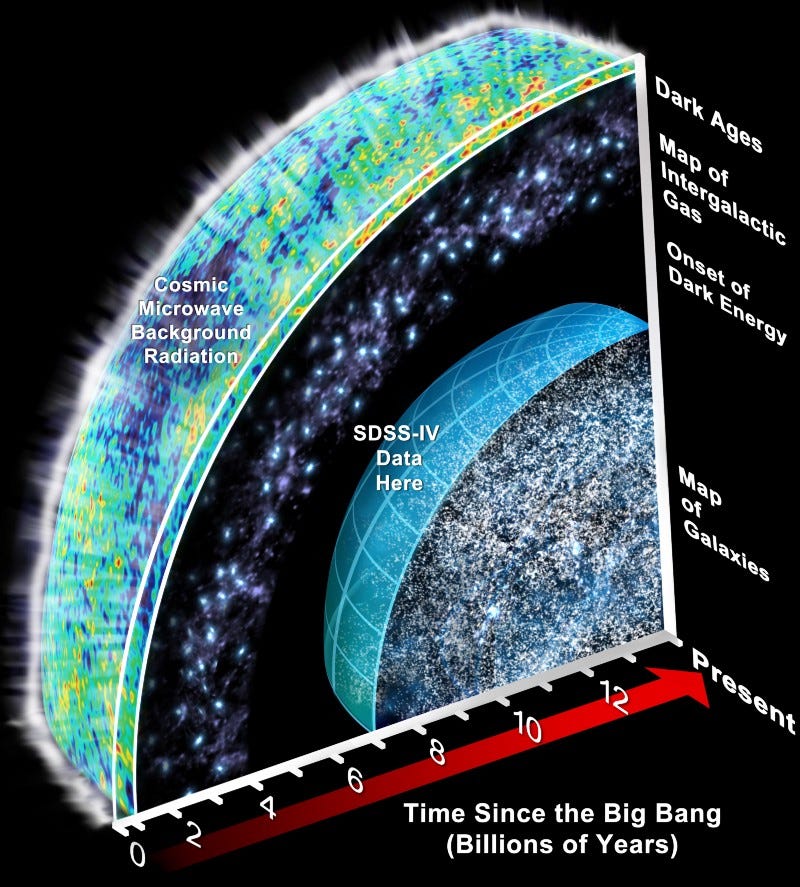
Similarly, large-scale structure surveys have become incredibly ubiquitous, with some covering the entire sky and others covering huge patches at even greater depths. With the Sloan Digital Sky Survey providing the best modern data sets, we’ve been able to confirm the first five of these six predictions, placing inflation on a very firm footing.
- The Universe is observed to be exactly spatially flat — with a curvature of 1, exactly — to a precision of 1.0007 ± 0.0025, as best shown by the large-scale structure of the Universe.
- The fluctuations in the cosmic microwave background show a Universe with scales that extend up to and beyond the horizon of the observable Universe.
- The maximum temperature that our Universe ever could have achieved, as shown by the fluctuations in the cosmic microwave background, is only ~10^16 GeV, or a factor of 1,000 smaller than a non-inflationary Universe.
- The types of fluctuations the Universe was born with, to the best of our measurements, are 100% adiabatic, and 0% isocurvature. The correlations between the cosmic microwave background and the large-scale structure of the Universe show this, although this wasn’t confirmed until the early 2000s.
- And from the latest data from the most advanced cosmic microwave background satellite, Planck, gives us a scalar spectral index (which comes from the densityfluctuations) that’s not only less than 1, it’s precisely measured to be n_s = 0.968 ± 0.006.
That last number, n_s, is really, really important if we want to look for the sixth and final prediction of inflation: gravitational wave fluctuations.
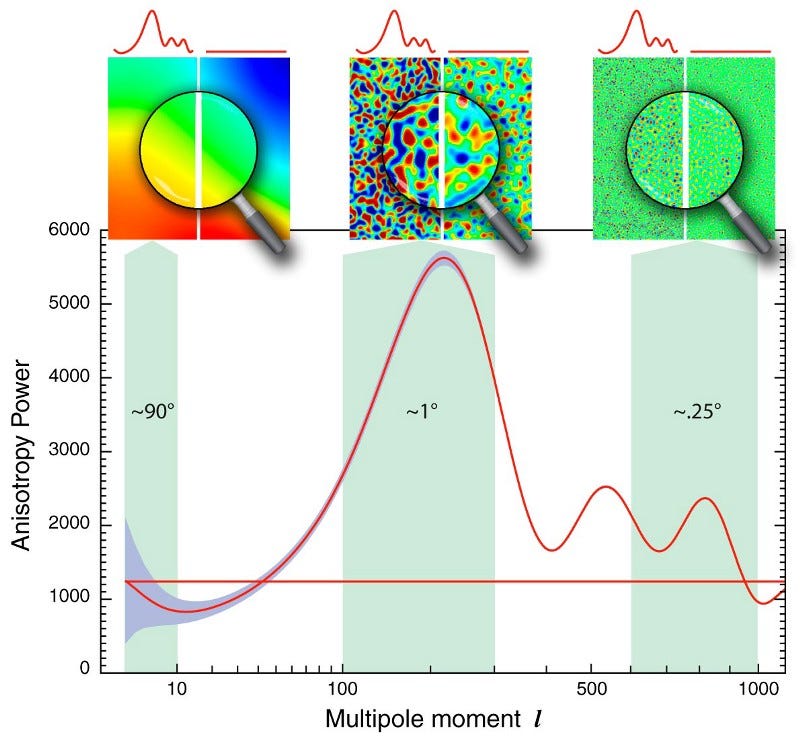
The spectrum of fluctuations in the microwave background looks like the squiggled line, above, today, but it grew out of the interplay of all the different forms of energy over time, from the end of inflation until the Universe was 380,000 years old. It grew from the density fluctuations at the end of inflation: the horizontal line. Only, that line isn’t quite horizontal; there’s a slight tilt to the line, and the slope represents the departure of spectral index, n_s, from 1.
The reason this is important is that inflation makes a specific prediction for a special ratio (r), where r is the ratio of the gravitational wave fluctuations to the scalar spectral index, n_s. For the two main classes of inflationary models — as well as in other models — there is a huge disparity in what r is predicted to be.
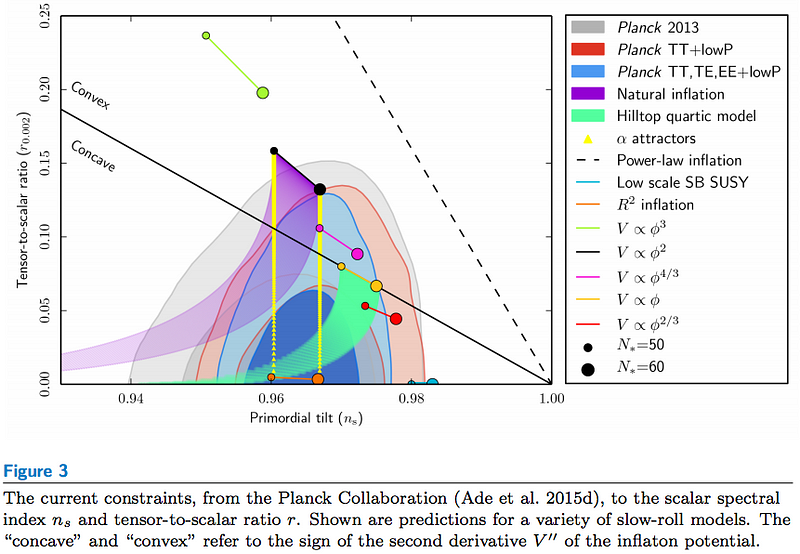
For chaotic models, r is typically very large: no smaller than about 0.01, where 1 is the maximum conceivable value. But for the new inflation models,r can vary from as large as about 0.05 down to tiny, minuscule numbers like 10^–60! But these various r values are often correlated with specific values for ns, as you can see above. If n_s turns out to actually be the value that we’ve best measured it to be right now — 0.968 — then the simplest models you can write down for both chaotic inflation and new inflation only give values of r that are bigger than about 10^–3.
As reported by Mark Kamionkowski in his talk at AAS (and based on his paper here), all the simple models one can write down, for the measured value of n_s, means that r can’t range from 10^–60 to 1; it can only range from 10^–3 to 1. And this could be very, very problematic in short order, because there are a whole host of ground-based surveys that are measuring the type of signal that can measure r, already constrained to be less than 0.09, if it’s greater than or equal to ~10^–3.
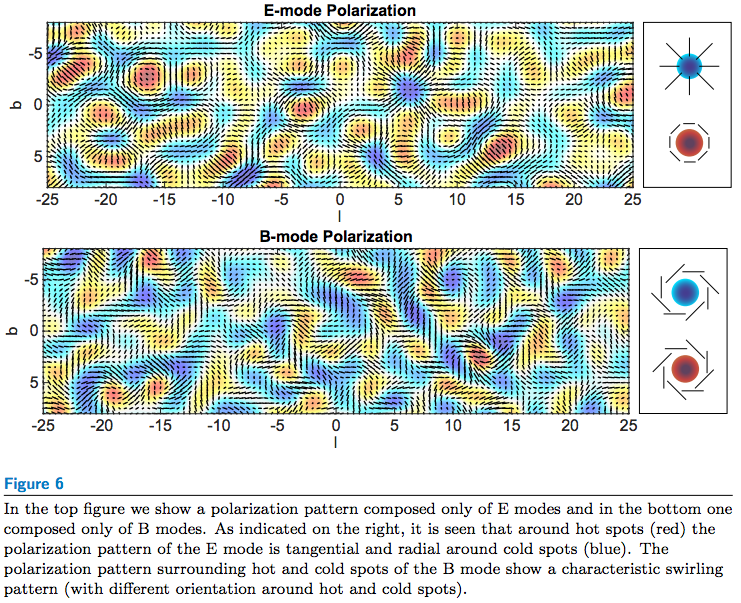
The gravitational wave fluctuations produced by inflation cause both E-mode and B-mode polarizations, but the density fluctuations (and ns) show up in only the E-modes. So if you measure the B-mode polarizations, you can learn about the gravitational wave fluctuations and determine r!
This is what experiments such as BICEP2, POLARBEAR, SPTPOL and SPIDER, among others, are working to measure right now. There are B-mode polarization signals caused by lensing effects, but if the inflationary fluctuations are larger than r ~ 0.001, they’ll be able to be seen in 5–10 years by the experiments running and planned to run over that time.
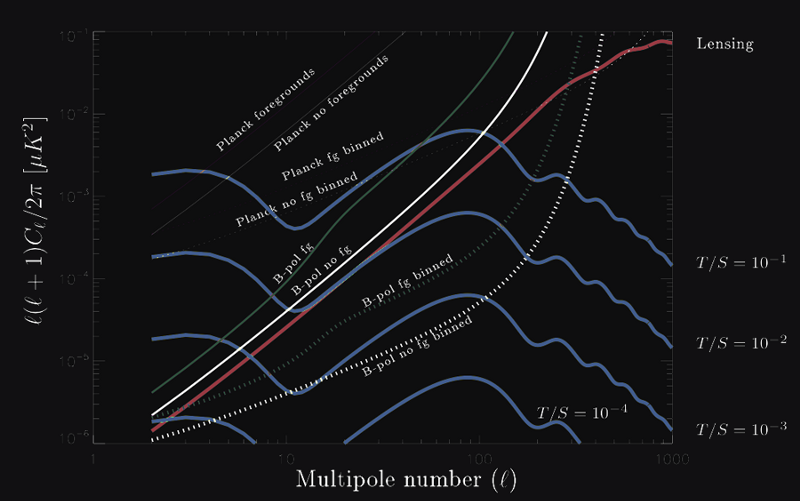
If we find a positive signal for r, either a chaotic inflation (typically if r > 0.02) or a new inflation (typically for r < 0.04, and yes, there’s overlap) model could be strongly, strongly favored. But if the measured value for n_s stays what it’s thought to be right now, and after a decade we’ve constrained r < 10^–3, then the simplest models for inflation are all wrong. It doesn’t mean inflation is wrong, but it means inflation is something more complicated than we first thought, and perhaps not even a scalar field at all.
If nature is unkind to us, the last great prediction of cosmic inflation — the existence of primordial gravitational waves — will be elusive to us for many decades to come, and will continue to go unconfirmed.
This article was partially based on information obtained during the 227th American Astronomical Society meeting, some of which may be unpublished.
Leave your comments on our forum, and check out our first book: Beyond The Galaxy, available now, as well as our reward-rich Patreon campaign!