When Did The Universe Become Transparent To Light?
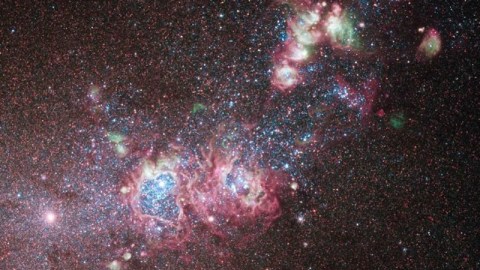
Depending on how you measure it, there are two different answers that could be right.
If you want to see what’s out there in the Universe, you first have to be able to see. We take for granted, today, that the Universe is transparent to light, and that the light from distant objects can travel unimpeded through space before reaching our eyes. But it wasn’t always this way.
In fact, there are two ways that the Universe can stop light from propagating in a straight line. One is to fill the Universe with free, unbound electrons. The light will then scatter with the electrons, bouncing off in a randomly-determined direction. The other is to fill the Universe with neutral atoms that can clump and cluster together. The light will then be blocked by this matter, the same way that most solid objects are opaque to light. Our actual Universe does both of these, and won’t become transparent until both obstacles are overcome.
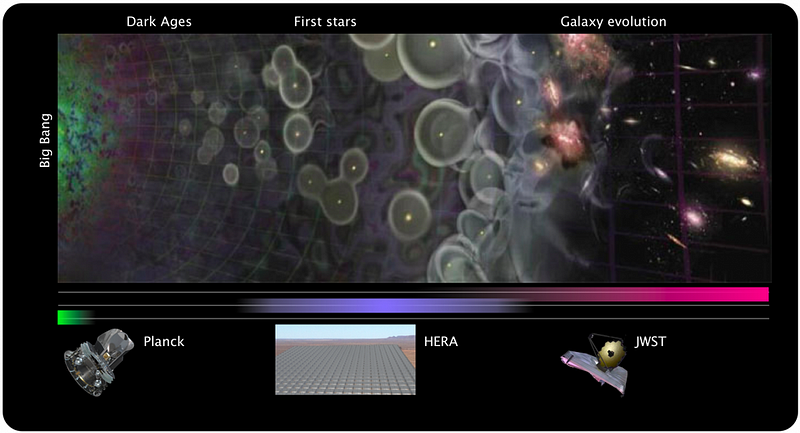
In the earliest stages of the Universe, the atoms that make up everything we know of weren’t bound together in neutral configurations, but rather were ionized: in the state of a plasma. When light travels through a dense-enough plasma, it will scatter off of the electrons, being absorbed and re-emitted in a variety of unpredictable directions. So long as there are enough free electrons, the photons streaming through the Universe will continue to be kicked around at random.
There’s a competing process occurring, however, even during these early stages. This plasma is made of electrons and atomic nuclei, and it’s energetically favorable for them to bind together. Occasionally, even at these early times, they do exactly that, with only the input from a sufficiently energetic photon capable of splitting them apart once again.
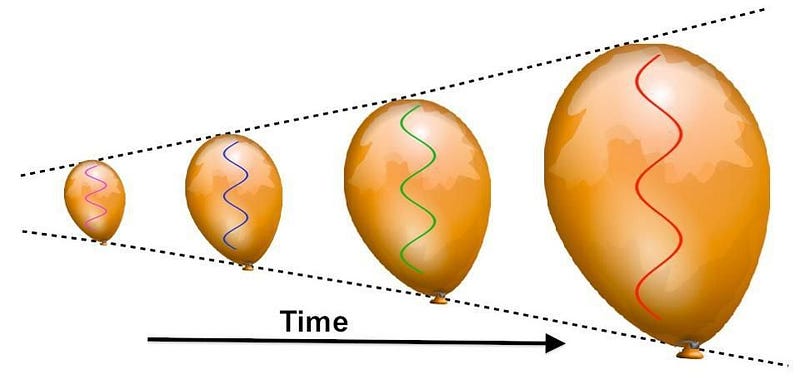
As the Universe expands, however, it not only gets less dense, but the particles within it get less energetic. Because the fabric of space itself is what’s expanding, it affects every photon traveling through that space. Because a photon’s energy is determined by its wavelength, then as that wavelength gets stretched, the photon gets shifted — redshifted — to lower energies.
It’s only a matter of time, then, until all the photons in the Universe drop below a critical energy threshold: the energy required to knock an electron off of the individual atoms that exist in the early Universe. It takes hundreds of thousands of years after the Big Bang for photons to lose enough energy to make the formation of neutral atoms even possible.
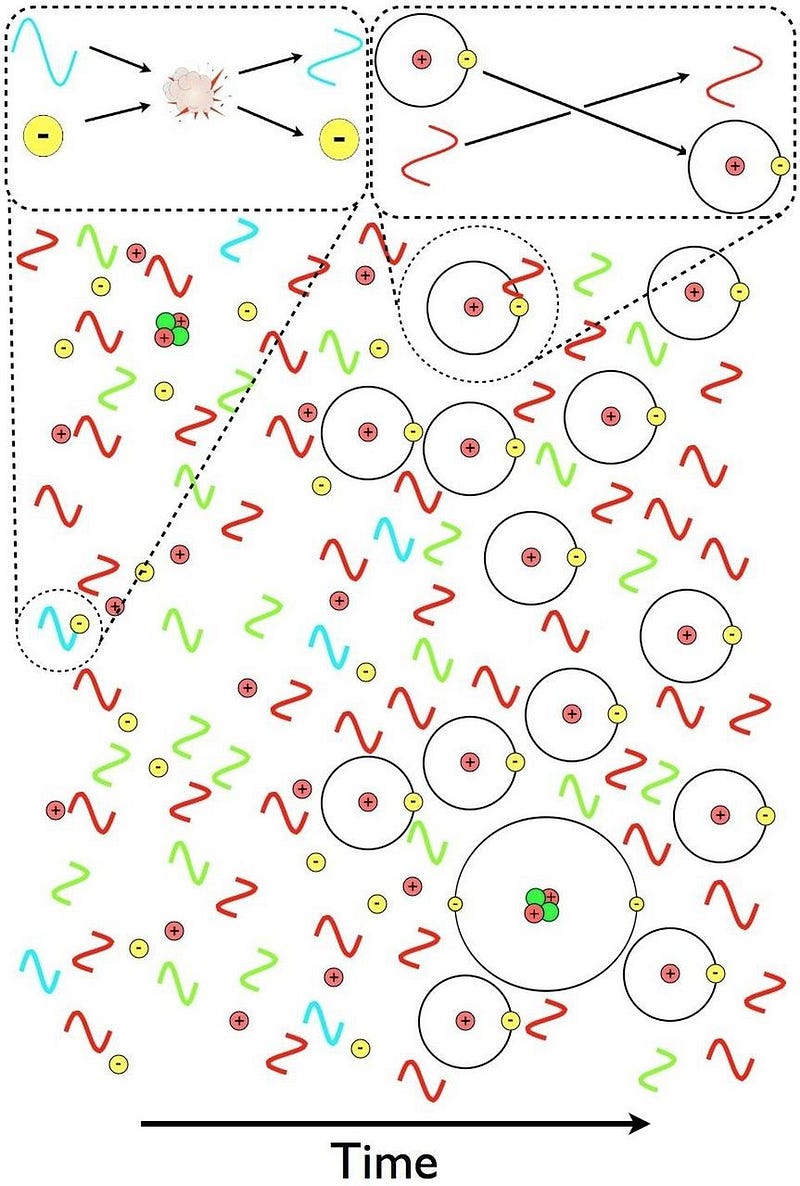
Many cosmic events happen during this time: the earliest unstable isotopes radioactively decay; matter becomes more energetically important than radiation; gravitation begins pulling matter into clumps as the seeds of structure start growing. As the photons become more and more redshifted, another barrier to neutral atoms appears: the photons emitted when electrons bind to protons for the first time. Every time an electron successfully binds with an atomic nucleus, it does two things:
- It emits an ultraviolet photon, because atomic transitions always cascade down in energy levels in a predictable fashion.
- It gets bombarded by other particles, including the billion-or-so photons that exist for every electron in the Universe.
Every time you form a stable, neutral atom, it emits an ultraviolet photon. Those photons then continue on, in a straight line, until they encounter another neutral atom, which they then ionize.
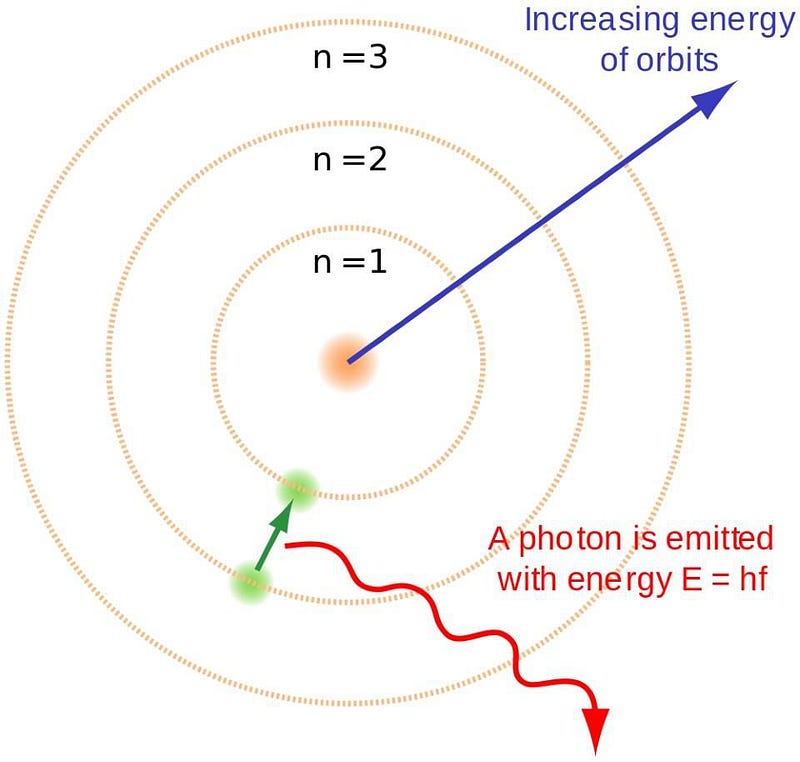
There’s no net addition of neutral atoms through this mechanism, and hence the Universe cannot become transparent to light through this pathway alone. There’s another effect that comes in, instead, that dominates. It’s extremely rare, but given all the atoms in the Universe and the more-than-100,000 years it takes for atoms to finally and stably become neutral, it’s an incredible and intricate part of the story.
Most times, in a hydrogen atom, when you have an electron occupying the first excited state, it simply drops down to the lowest-energy state, emitting an ultraviolet photon of a specific energy: a Lyman alpha photon. But about 1 time in 100 million transitions, the drop-down will occur through a different path, instead emitting two lower-energy photons. This is known as a two-photon decay or transition, and is what is primarily responsible for the Universe becoming neutral.
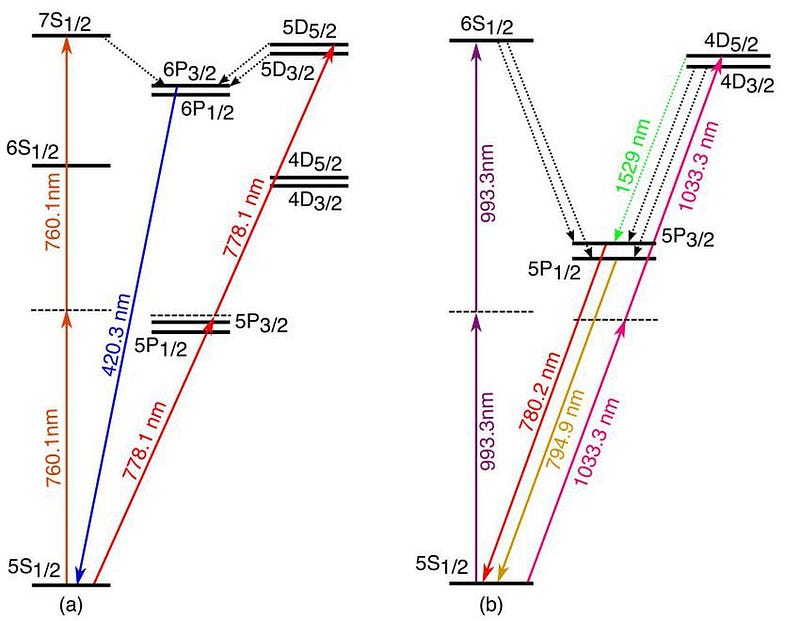
When you emit a single photon, it almost always collides with another hydrogen atom, exciting it and eventually leading to its reionization. But when you emit two photons, it’s extraordinarily unlikely that both will hit an atom at the same time, meaning that you net one additional neutral atom.
This two-photon transition, rare though it is, is the process by which neutral atoms first form. It takes us from a hot, plasma-filled Universe to an almost-equally-hot Universe filled with 100% neutral atoms. Although we say that the Universe formed these atoms 380,000 years after the Big Bang, this was actually a slow, gradual process that took about 100,000 years on either side of that figure to complete. Once the atoms are neutral, there is nothing left for the Big Bang’s light to scatter off of. This is the origin of the CMB: the Cosmic Microwave Background.
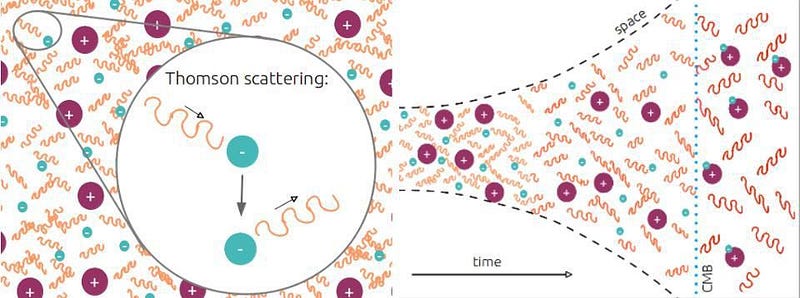
This marks the first time that the Universe becomes transparent to light. The leftover photons from the Big Bang, now long in wavelength and low in energy, can finally travel freely through the Universe. With the free electrons gone — bound up into stable, neutral atoms — the photons have nothing to stop them or slow them down.
But the neutral atoms are now everywhere, and they serve an insidious purpose. While they may make the Universe transparent to these low-energy photons, these atoms will clump together into molecular clouds, dust, and collections of gas. Neutral atoms in these configurations might be transparent to low-energy light, but the higher-energy light, like that emitted by stars, gets absorbed by them.
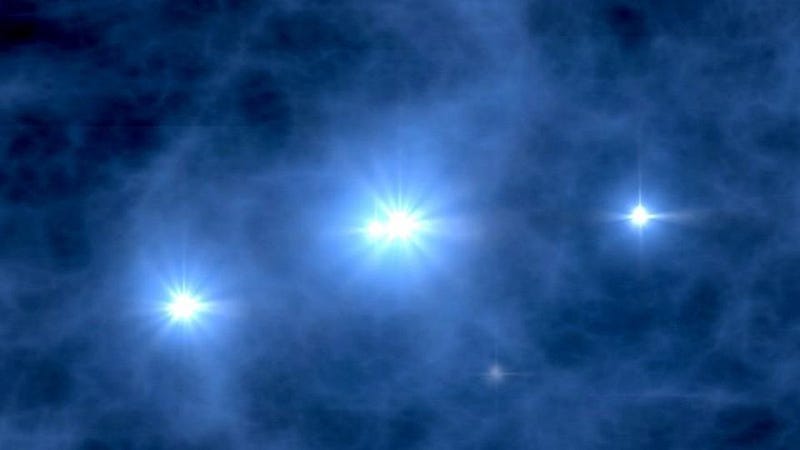
When all of the atoms in the Universe are now neutral, they do an amazingly good job of blocking starlight. The same long-awaited configuration that we required to make the Universe transparent now makes it opaque again to photons of a different wavelength: the ultraviolet, optical, and near-infrared light produced by stars.
In order to make the Universe transparent to this other type of light, we’ll need to ionize them all again. This means that we need enough high-energy light to kick the electrons off of the atoms they’re bound to, which requires an intense source of ultraviolet emission.
In other words, the Universe needs to form enough stars to successfully reionize the atoms within it, rendering the tenuous, low-density intergalactic medium transparent to starlight.
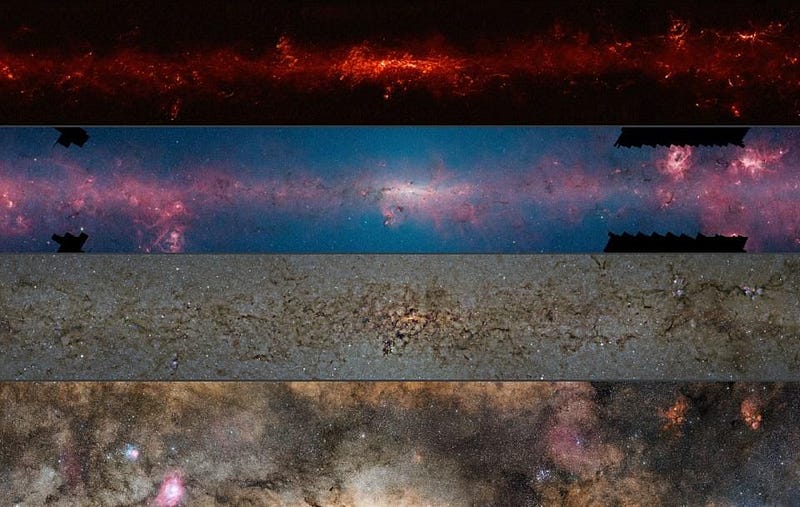
We see this even in our own galaxy: the galactic center cannot be seen in visible light. The galactic plane is rich in neutral dust and gas, which is extremely successful at blocking the higher-energy ultraviolet and visible light, but infrared light goes clear through. This explains why the cosmic microwave background won’t get absorbed by neutral atoms, but starlight will.
Thankfully, the stars that we form can be massive and hot, where the most massive ones are much more luminous and hotter than even our Sun. Early stars can be tens, hundreds, or even a thousand times as massive as our own Sun, meaning they can reach surface temperatures of tens of thousands of degrees and brightnesses that are millions of times as luminous as our Sun. These behemoths are the biggest threat to the neutral atoms spread throughout the Universe.
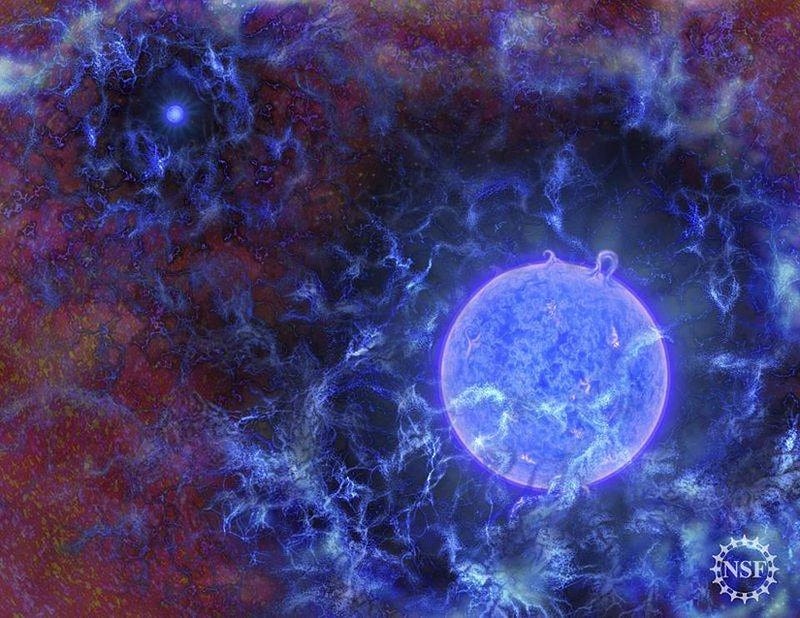
What we need to happen is for enough stars to form that they can flood the Universe with a sufficient number of ultraviolet photons. If they can ionize enough of this neutral matter filling the intergalactic medium, they can clear a path in all directions for starlight to travel unimpeded. Moreover, it has to occur in sufficient amounts that the ionized protons and electrons can’t get back together again. There is no room for Ross-and-Rachel style shenanigans in the effort to reionize the Universe.
The first stars make a small dent in this, but the earliest star clusters are small and short-lived. For the first few hundred million years of our Universe, all the stars that form can barely make a dent in how much of the matter in the Universe remains neutral. But that begins to change when star clusters merge together, forming the first galaxies.
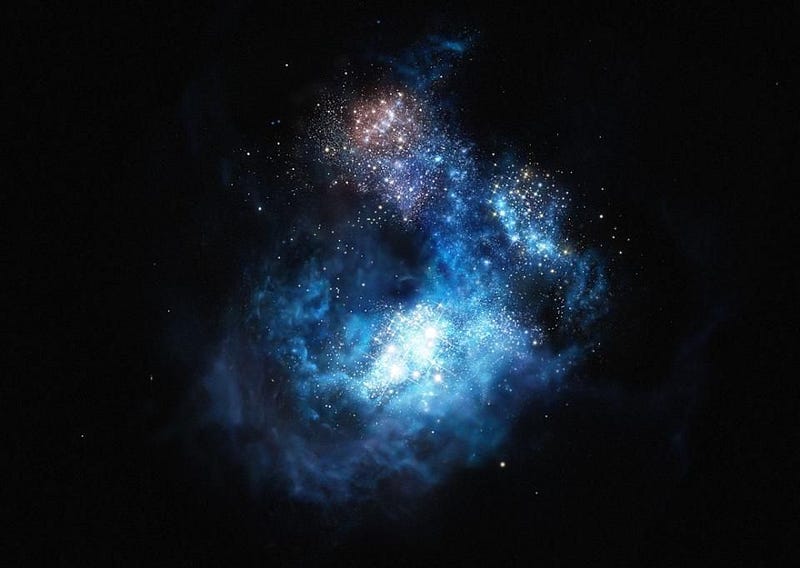
As large clumps of gas, stars, and other matter merge together, they trigger a tremendous burst of star formation, lighting up the Universe as never before. As time goes on, a slew of phenomena take place all at once:
- the regions with the largest collections of matter attract even more early stars and star clusters towards them,
- the regions that haven’t yet formed stars can begin to,
- and the regions where the first galaxies are made attract other young galaxies,
all of which serves to increase the overall star formation rate.
If we were to map out the Universe at this time, what we’d see is that the star formation rate increases at a relatively constant rate for the first few billion years of the Universe’s existence. In some favorable regions, enough of the matter gets ionized early enough that we can see through the Universe before most regions are reionized; in others, it may take as long as two or three billion years for the last neutral matter to be blown away.
If you were to map out the Universe’s neutral matter from the start of the Big Bang, you would find that it starts to transition to ionized matter in clumps, but you’d also find that it took hundreds of millions of years to mostly disappear. It does so unevenly, and preferentially along the locations of the densest parts of the cosmic web.
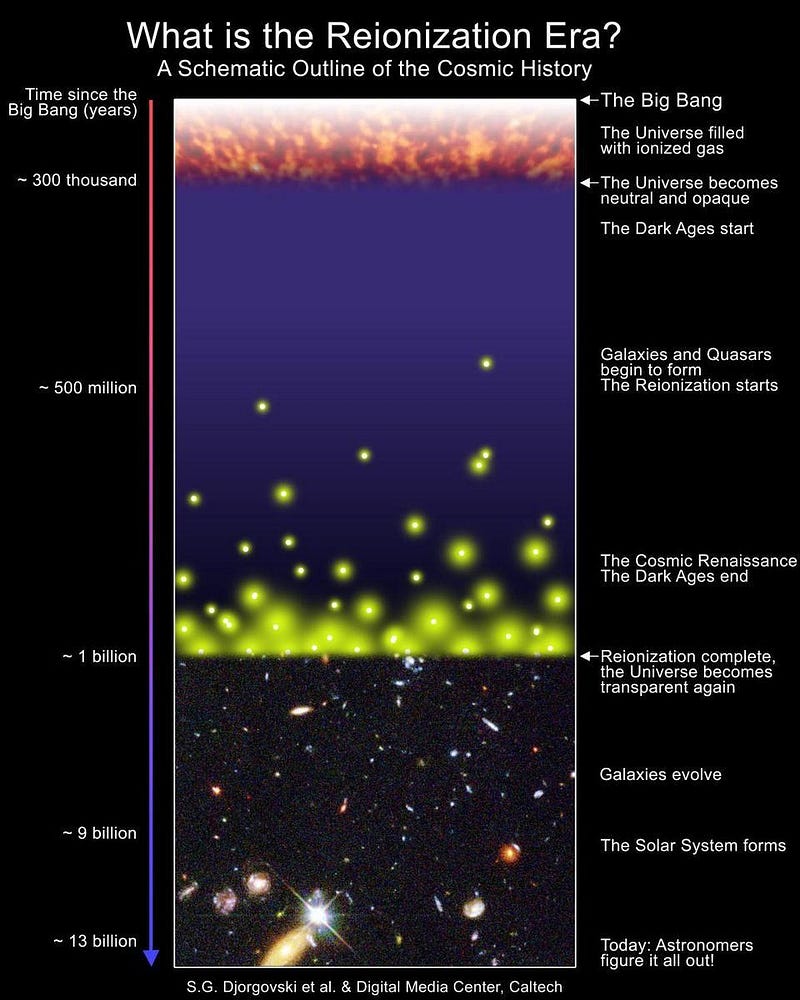
On average, it takes 550 million years from the inception of the Big Bang for the Universe to become reionized and transparent to starlight. We see this from observing ultra-distant quasars, which continue to display the absorption features that only neutral, intervening matter causes. But reionization doesn’t happen everywhere at once; it reaches completion at different times in different directions and at different locations. The Universe is uneven, and so are the stars and galaxies and clumps of matter that form within it.
The Universe became transparent to the light left over from the Big Bang when it was roughly 380,000 years old, and remained transparent to long-wavelength light thereafter. But it was only when the Universe reached about half a billion years of age that it became fully transparent to starlight, with some locations experiencing transparency earlier and others experiencing it later.
To probe beyond these limits requires a telescope that goes to longer and longer wavelengths. With any luck, the James Webb Space Telescope will finally open our eyes to the Universe as it was during this in-between era, where it’s transparent to the Big Bang’s glow but not to starlight. When it opens its eyes on the Universe, we may finally learn just how the Universe grew up during these poorly-understood dark ages.
Ethan Siegel is the author of Beyond the Galaxy and Treknology. You can pre-order his third book, currently in development: the Encyclopaedia Cosmologica.