What Was It Like When The Universe Made Its Heaviest Elements?
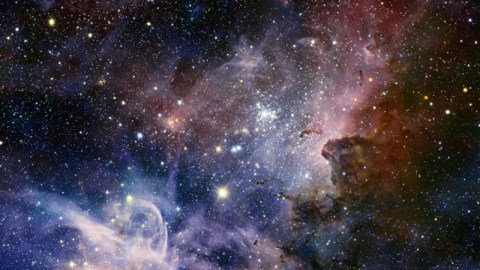
The heaviest elements in the periodic table have their own unique story. No, they don’t come from a supernova.
When it comes to the elements of the Universe, every one of them has its own unique story. Hydrogen and helium were created in the earliest stages of the Big Bang; light elements like carbon and oxygen are created in Sun-like stars; heavier elements like silicon, sulfur and iron are created in more massive stars; elements beyond iron are made when those massive stars explode in supernovae.
But the most massive elements of all at the very high end of the periodic table — including platinum, gold, radon, and even uranium — owe their origins to an even rarer, more energetic process. The heaviest elements of all come from merging neutron stars, a fact that was long suspected but only confirmed in 2017. Here’s the cosmic story of how the Universe got there.
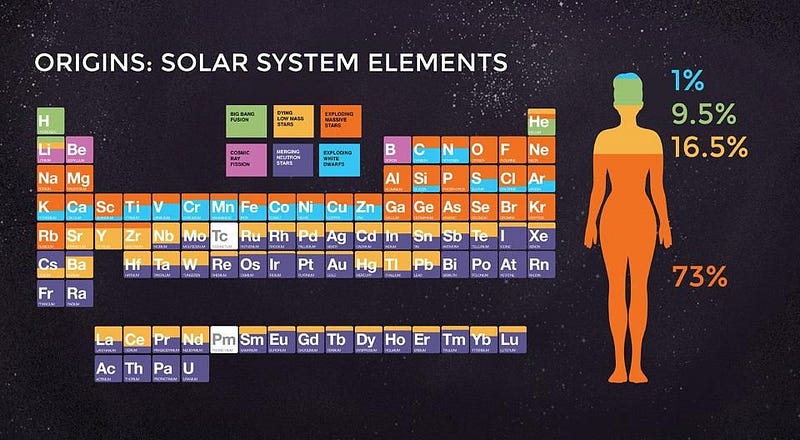
Whenever you form stars, they arise from a large molecular cloud of gas that contracts down into a variety of clumps. The clumps grow more and more massive over time, as the atoms and molecules inside radiate heat away and allow them to collapse down. Eventually, they grow massive and dense enough that nuclear fusion can ignite inside of them. Eventually, these clumps will evolve into stars.
In the earliest stages, with just hydrogen and helium, stars grew to enormous masses: tens, hundreds, or even thousands of times the mass of the Sun, typically. Later on, the presence of heavier elements enabled more efficient cooling, keeping the average mass much lower and limiting the maximum to only 200–300 times as great as our Sun.
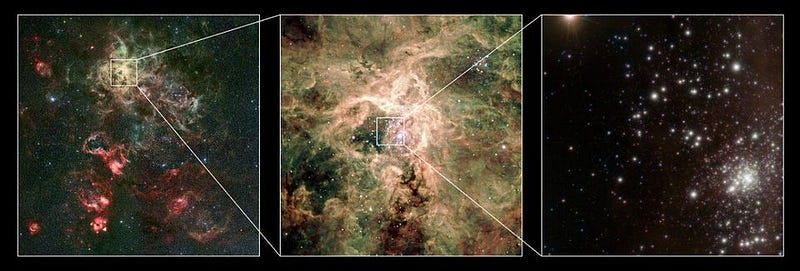
Still, even today, stars come in a wide variety of masses and sizes. They also come in a wide variety of distributions. While many of the star systems out there are similar to our own — possessing only one star surrounded by planets — multi-star systems are extremely common as well.
The REsearch Consortium On Nearby Stars (RECONS) surveyed all the stars they could find within 25 parsecs (about 81 light-years), and discovered 2,959 stars total. Of those, 1533 were single star systems, but the remaining 1426 were bound into binary, trinary, or even more complex systems. As our observations have shown us, these clustering properties are independent of mass. Even the most massive stars can be commonly found grouped together in twos, threes, or even greater numbers.
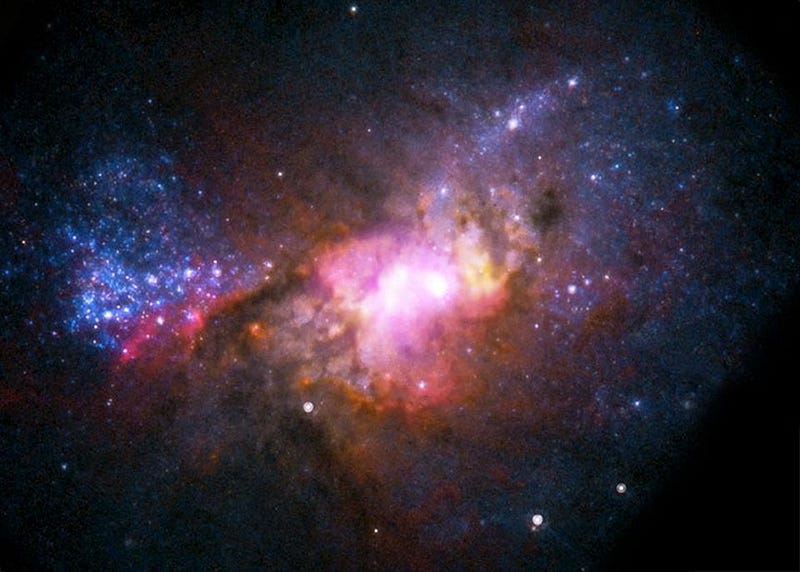
Over the history of the Universe, the most massive periods of star formation occur when galaxies interact, merge together, or fall into massive groups and clusters. These events will gravitationally perturb the hydrogen gas present within a galaxy, triggering an event known as a starburst. During a starburst, that gas gets rapidly converted into stars of all masses and in enormous varieties of groupings: singles, binaries, trinaries, all the way up to at least sextuple systems.
The more numerous, less massive stars will burn through their fuel slowly, living extremely long times. About 80–90% of the stars ever created are still fusing hydrogen into helium, and will remain doing so until more time than the present age of the Universe has gone by. The next step up in mass, to Sun-like stars, makes a big difference for a large slew of elements present in our Solar System today.
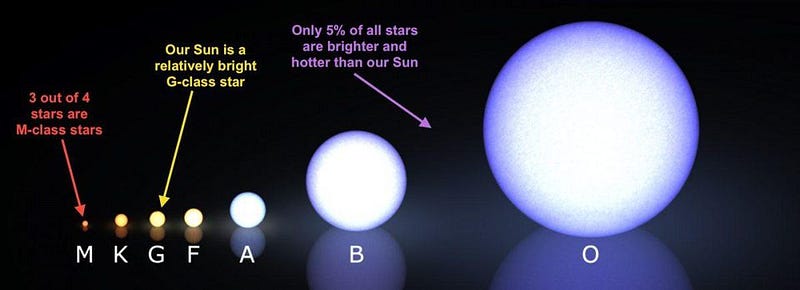
During most of their lives, Sun-like stars will fuse hydrogen into helium, while during the late stages, they swell into red giants while their cores fuse helium into carbon. As they evolve, however, and approach the end of their lives, these stars begin producing free neutrons, which start to get absorbed by the other nuclei present within the star.
One by one, neutrons get absorbed by a variety of nuclei, allowing us to not only create elements like nitrogen, but many of the heavier elements that go beyond what are made in supernovae. Strontium, zircon, tin and barium are examples; smaller amounts of elements like tungsten, mercury and lead are also produced. But lead is the limit; the next element upwards is bismuth, which is unstable. As soon as lead absorbs a neutron, bismuth decays, and so we’re back below lead again. Sun-like stars cannot get us over that hump.
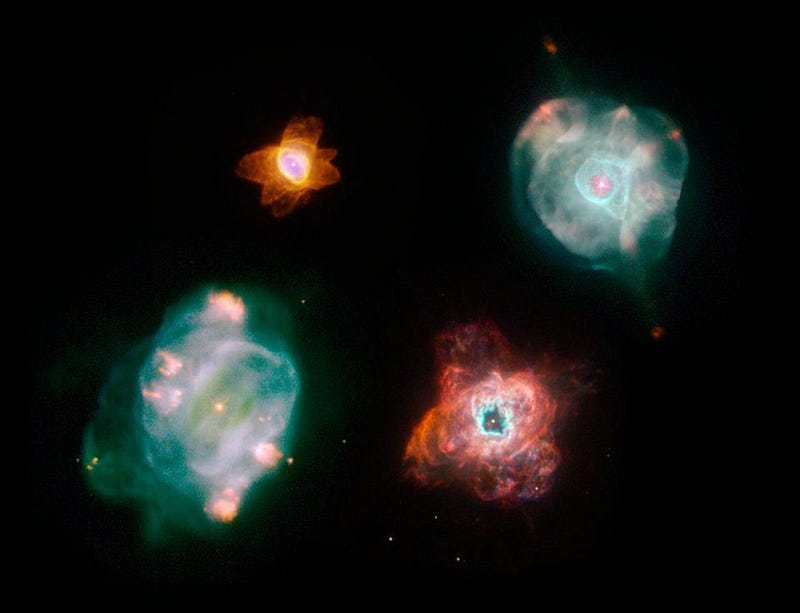
Neither can the most massive stars. Although they’re quite small in number, these cosmic behemoths account for a significant fraction of the total mass that goes into star formation. These stars, despite having the most matter inside them, are the shortest-lived, since they burn through their fuel far more quickly than any of the other star types. They fuse hydrogen into helium, helium into carbon, and then work their way up the periodic table to iron.
After iron, however, there’s nowhere to go that’s energetically favorable. These stars, in their final moments, see their cores implode, creating either neutron stars or black holes at their centers, while triggering a runaway fusion reaction in the outer layers. The result is a supernova explosion, coupled with a barrage of neutrons that rapidly get captured, creating many of the elements heavier than iron.
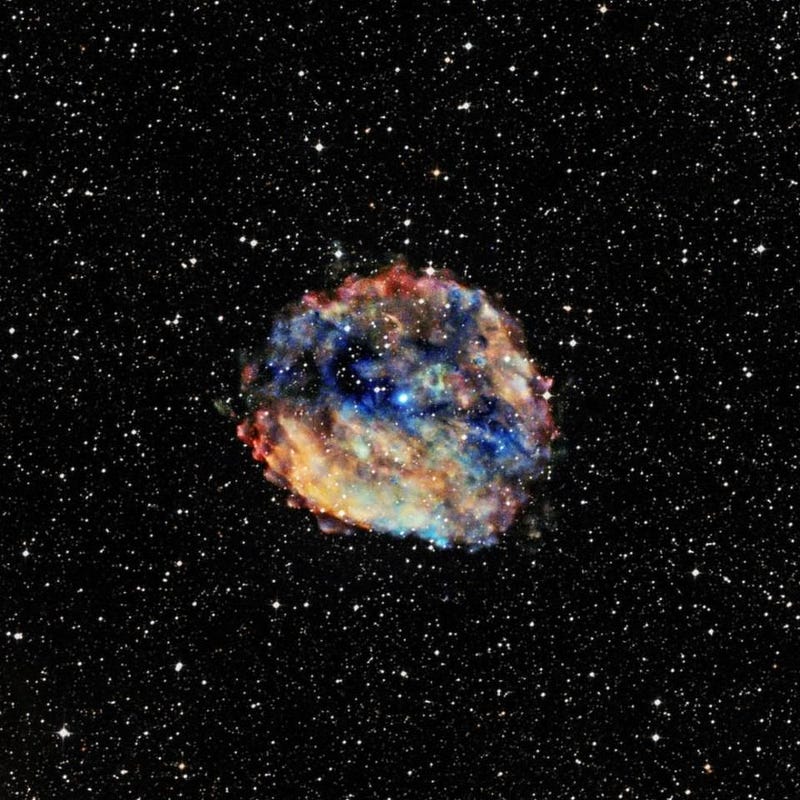
Still, there are gaping holes in the periodic table, even with all of this. At the low end, lithium, beryllium and boron will only get created when high-energy particles zipping through the Universe — cosmic rays — slam into nuclei, blasting them apart through a process known as spallation.
At the high end, elements from rubidium (element 44) and upwards, including most of the iodine, iridium, platinum, gold, and every element heavier than lead requires something else. These supernovae, many of which occur in binary systems, will very frequently leave neutron stars behind. When two or more stars go supernova in the same system, the existence of multiple neutron stars bound together leads to a tremendous possibility: a binary neutron star merger.
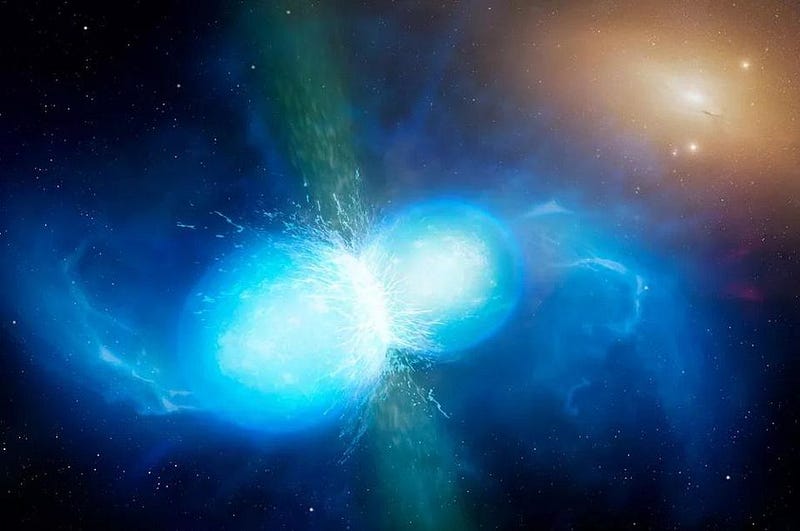
For a long time, it was speculated that merging neutron stars would provide the origin of these elements, as two massive balls of neutrons smashing together could create an endless variety of heavy atomic nuclei. Sure, most of the mass from these objects would merge together into a final-stage object like a black hole, but a few percent should be ejected as part of the collision.
In 2017, observations made with both telescopes and with gravitational wave observatories confirmed that not only are neutron star mergers responsible for the overwhelming majority of these heavy elements, but that short-period gamma ray bursts can be linked to these mergers as well. Now known as a kilonova, it’s well-understood that neutron star-neutron star mergers are the origin of the majority of the heaviest elements found throughout the Universe.
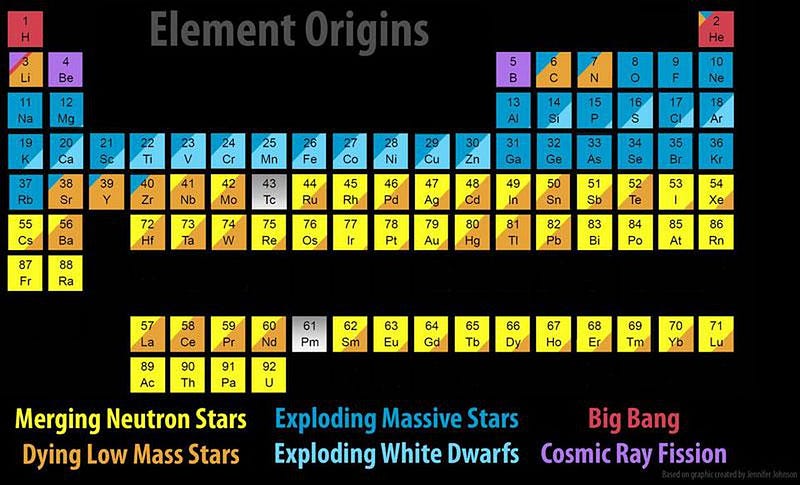
We frequently, when we talk about the history of the Universe, discuss it as though it were a series of events that happened at particular, well-defined instants in time. Although there are some moments in cosmic history that can be classed that way, the lives and deaths of stars are not so easily categorized.
Star formation increases for the first 3 billion years after the Big Bang, then falls off and gradually declines. Heavy elements are present from when the Universe was less than 100 million years old, but the last populations of pristine gas were not destroyed until 2–3 billion years after the Big Bang.
And the elements of the periodic table are continuously being created and destroyed by these processes that take place mostly inside stars and in interacting stellar remnants. Remarkably, we know today how many elements and of what different types are present, but it’s a story that’s constantly in flux.
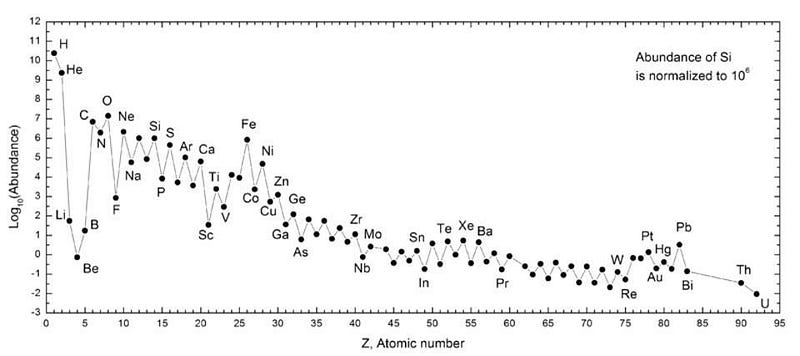
The heaviest elements of all, though, were created through one mechanism alone: neutron star mergers. Sure, supernovae can get you all the way up the periodic table, but only in insignificant amounts. Dying Sun-like stars can slowly drive the creation of heavier and heavier elements, but you cannot maintain anything beyond lead through that process. Cosmically, the only way we create significant amounts of the heaviest elements of all is through the inspiral and merger of the densest physical objects in the known Universe: neutron stars.
Now that gravitational wave observatories have confirmed our cosmic picture of this creation, the tools and technology are at hand for investigating them further and in greater detail. The next step will show us, observationally, how the Universe’s elemental abundances have evolved throughout space. At long last, a map of the chemical history of the Universe is within our reach.
Further reading on what the Universe was like when:
- What was it like when the Universe was inflating?
- What was it like when the Big Bang first began?
- What was it like when the Universe was at its hottest?
- What was it like when the Universe first created more matter than antimatter?
- What was it like when the Higgs gave mass to the Universe?
- What was it like when we first made protons and neutrons?
- What was it like when we lost the last of our antimatter?
- What was it like when the Universe made its first elements?
- What was it like when the Universe first made atoms?
- What was it like when there were no stars in the Universe?
- What was it like when the first stars began illuminating the Universe?
- What was it like when the first stars died?
- What was it like when the Universe made its second generation of stars?
- What was it like when the Universe made the very first galaxies?
- What was it like when starlight first broke through the Universe’s neutral atoms?
- What was it like when the first supermassive black holes formed?
- What was it like when life in the Universe first became possible?
- What was it like when galaxies formed the greatest numbers of stars?
- What was it like when the first habitable planets formed?
- What was it like when the cosmic web took shape?
- What was it like when the Milky Way took shape?