Throwback Thursday: Why Observatories Shoot Lasers at the Universe
And how, in the end, they help us gain the resolution of a space-based telescope without leaving the ground!
Image credit: Y. Beletsky/ESO, via http://www.eso.org/public/images/potw1036a/.
“But certainly the laser proved to be what I realized it was going to be. At that moment in my life I was too ignorant in business law to be able to do it right, and if I did it over again probably the same damn thing would happen.” –Gordon Gould, inventor of the LASER
You’re used to the iconic image of an observatory’s dome surrounded by a dark sky. From within, a telescope peers up at the heavens. And with a huge amount of light-gathering power that dwarfs a fully dilated human eye, we can use this tremendous tool to peek into the dark depths of the Universe.
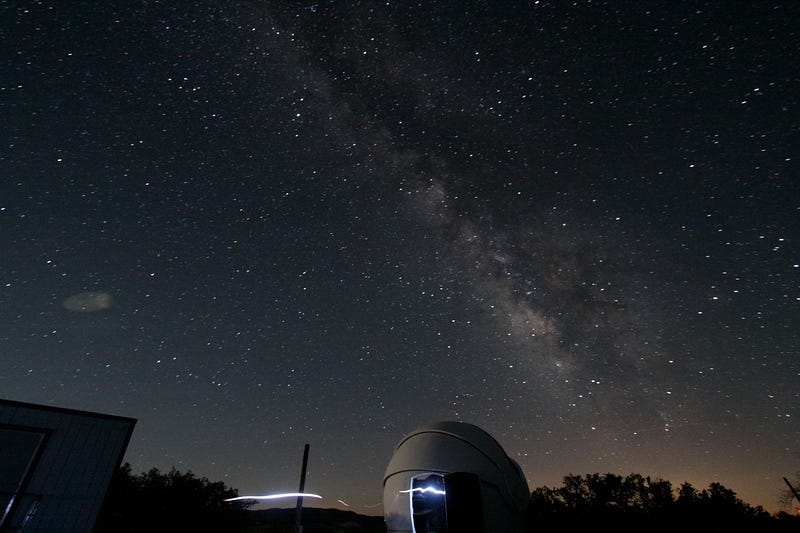
Size is a big deal in astronomy: if you double the diameter of your telescope, you quadruple your light gathering power. It’s no wonder that we keep going larger and larger, with the current largest telescopes at 10 meters in diameter and new ones planned to double, triple or even quadruple that!
Still, size isn’t everything. Nearly a century ago, Edwin Hubble was using the famed 100-inch Hooker telescope on Mt. Wilson. Along with the latest in photographic techniques, he was taking images like this one, in which he discovered that Andromeda — the galaxy in the picture — lay far beyond our Milky Way. This image, below, was taken back in 1923.
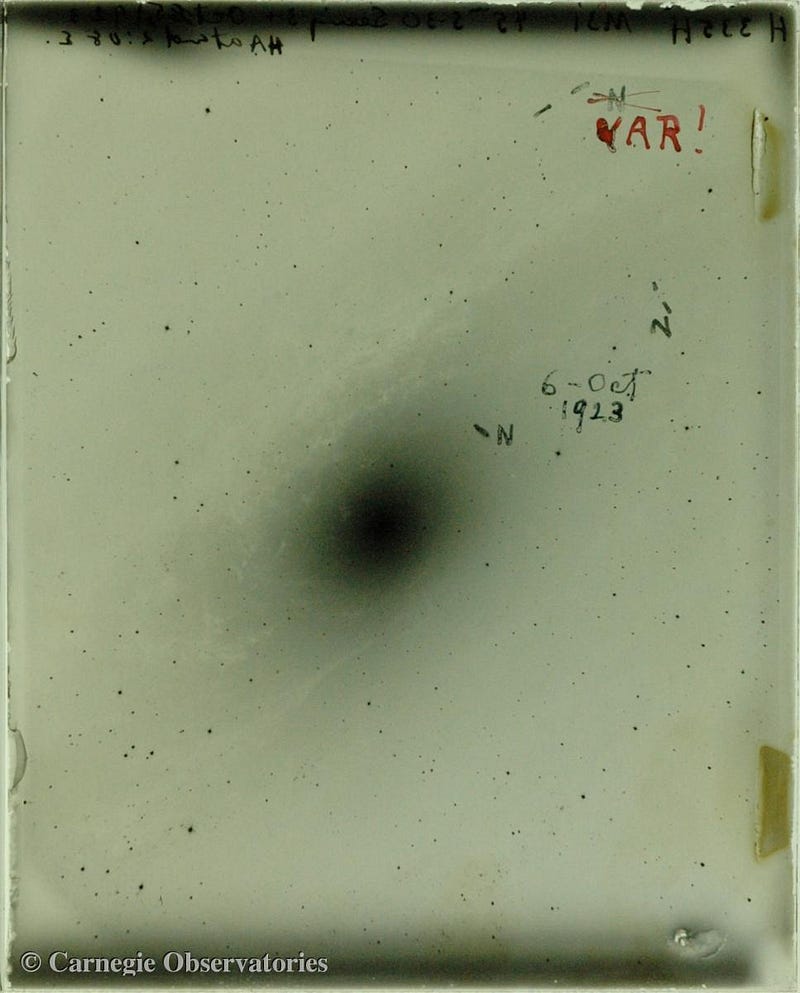
But although our images of Andromeda today are incredibly improved over this effort, it isn’t due to size. Remember: size isn’t everything. Nearly a century later, the largest optical telescopes are only about four times the diameter of the telescope Hubble was using a century ago, and there are only a handful that large. Even the Hubble Space Telescope — the greatest telescope of our generation — is smaller than that 100-inch relic!
Yet, when the Hubble telescope takes a look at a galaxy nearly 100 times as distant as Andromeda, it can make it out in far greater detail than Edwin Hubble could ever see looking at any galaxy, and in fact is able to resolve individual stars in there.
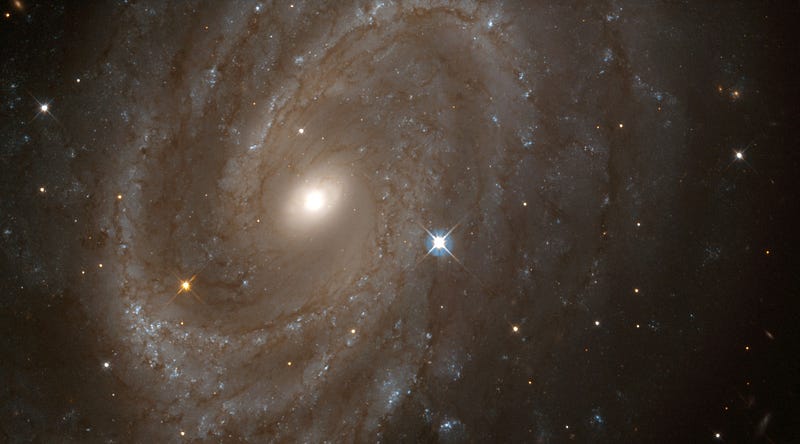
There are two reasons for this incredible improvement in quality: first off, there have been huge advances in optical systems. Photographic plates have been replaced with charge-coupled devices (CCDs), analog equipment has been replaced by digital, and photons can be counted one-at-a-time. In short, a hobbyist today — for just a few thousand dollars — can do better science than the most advanced professionals could — with equipment ten times the size — a century ago.
But the second reason the Hubble Space Telescope is so fantastic is its location: it’s in space!
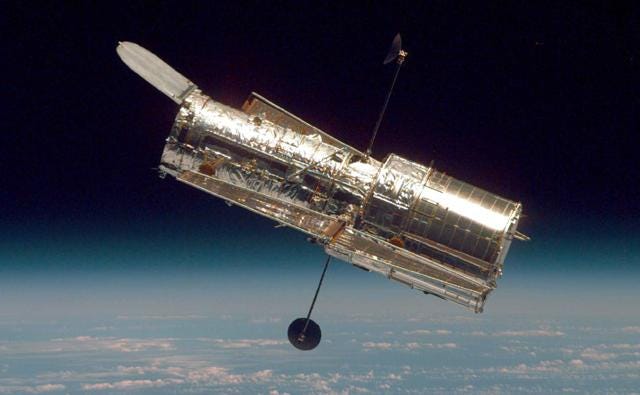
For astronomy, being in space is a tremendous advantage over being stuck here on the surface of the Earth. Take the following simple example: look up at a point of light in the night sky, and just watch it. Is it a constant, unflinching source of light, or does it twinkle, even a little bit?
If it twinkles, then what you’re looking at is a star. And if not, then it’s a planet, and this is the simplest way to tell the two apart without having to come back night-after-night and seeing if its position has changed.
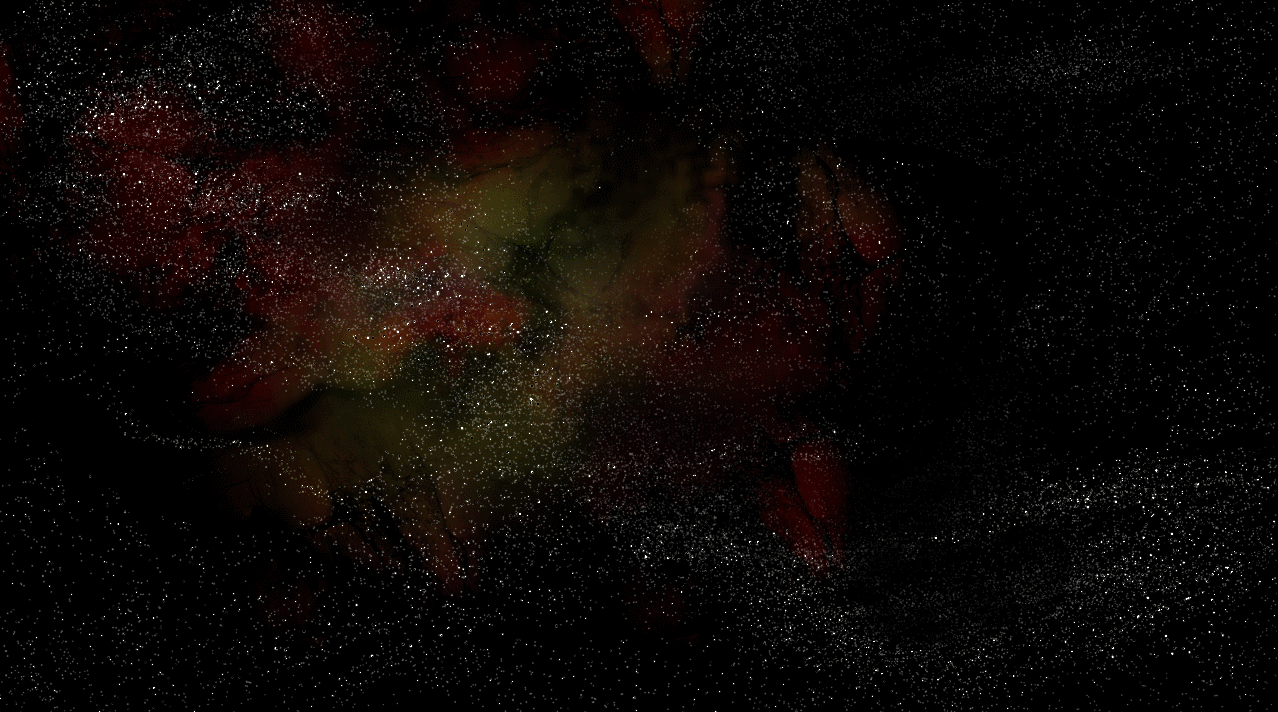
The first humans to see a star (other than the Sun) not twinkle in the sky were the first humans to travel to outer space: from the vantage point of anyone — human or telescope — it’s only the effects of the atmosphere that cause that twinkling. In reality, that star is fixed in the sky, and it shouldn’t matter whether you’re on the surface of the Earth or hundreds of miles (or kilometers) above it.
But if you’re observing a star from the ground, you need to peer through around 100 kilometers of atmosphere to get there, and with all those atoms boggling around, our view gets affected.
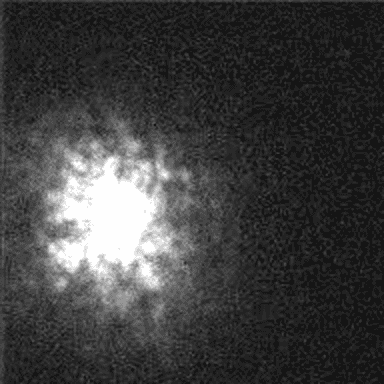
Our atmosphere is a turbulent entity, with gases rising and falling, and swooping past rapidly, from any point of view, in stratified layers. It’s fair to say that the lowest layers are the densest and the most disruptive to our observations, and that’s why we often build telescopes and observatories at extremely high altitudes: there’s less atmosphere to contend with!
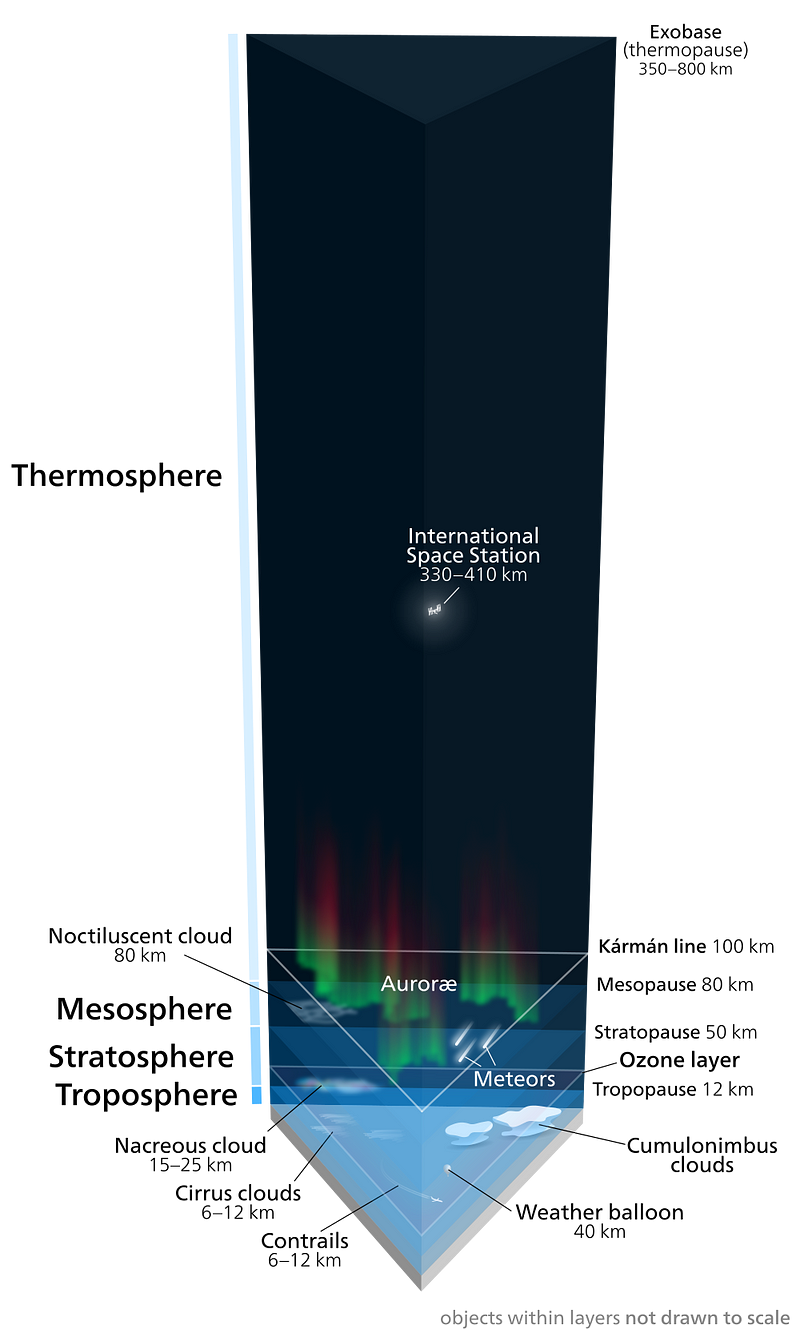
But if you’ve ever seen a photo like the one below — of an observatory shooting a yellow-orange laser into the night sky — this is our attempt to compensate for the atmosphere.
And not to toot our own horn too much, but what we’re actually doing is nothing short of brilliant.
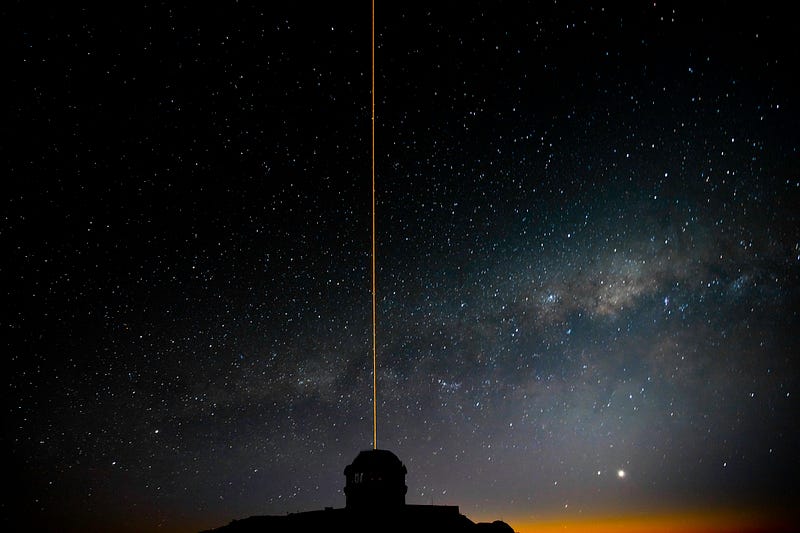
The laser used here on these observatories takes advantage of a special property of our atmosphere: certain elements are segregated from others at specific altitudes.
One of the elements that’s very rare is sodium, which happens to be concentrated in a thin layer about 100 km (60 miles) up. If you fire a sodium laser into the air, it will excite those sodium atoms found at that particular altitude, which then spontaneously de-excite, creating an artificial light source to be used as a guide star.
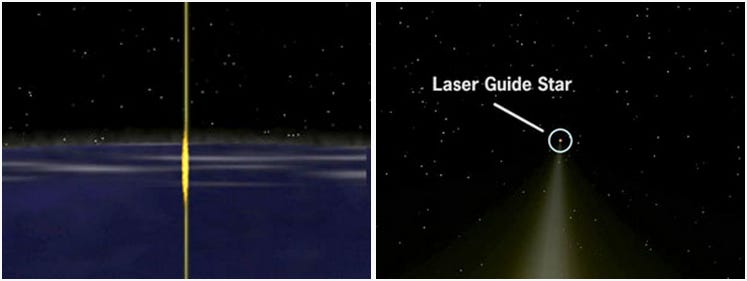
The light from this artificial star then travels back to the telescope through that 100 km of atmosphere, and gets distorted by that same turbulent air column that all the other light coming to your telescope must pass through. Only this time, we know for absolute certain that this should be a single, point source of a particular wavelength at a particular location. So no matter what the light that we actually get back from that artificial star looks like, we know what it should look like: that single point source.
So what do we do about it? We adapt.
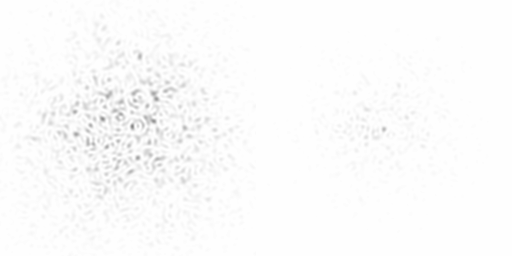
We can compute exactly what the shape of a mirror would need to be — at any instant — to undo the turbulent effects of the atmosphere, and return our artificial guide star to simply being a single point of light at the correct location.
What we then do is we delay the light from all the other sources coming into the telescope, and actually mechanically adapt a mirror along the light path to be the exact shape it needs to be to undo the effect of the atmosphere, which we then pass the delayed light through.
This provides a way for us to literally undo a huge portion of the atmosphere’s effects, rewarding us with an optical image that’s been corrected for all that turbulent air.
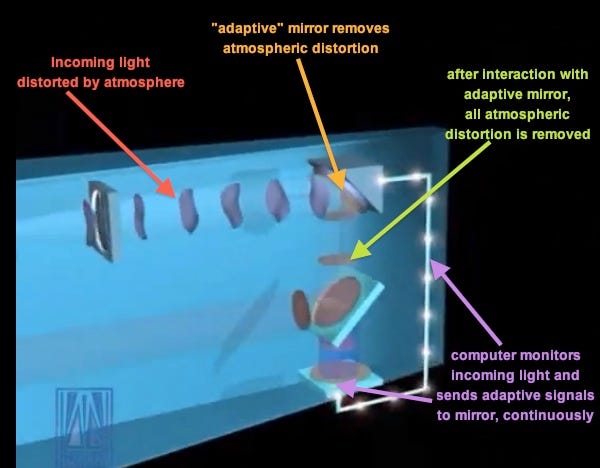
We update the shape of this mirror on a continuous basis, and this allows us to obtain — to the best of our ability — an image that undoes all the negative effects of the atmosphere. This entire setup is the most advanced technique in the field known as adaptive optics, and it’s perhaps the most spectacular, revolutionary advance in ground-based astronomy since the invention of photography. Here’s a lovely video from Gemini Observatory, detailing how the entire process works.
Adaptive optics, in general, has allowed us to resolve binary stars in a system that, without it, would look only like noisy pixels of light, simply jumping around.
As of 2012, for the first time, we’ve used this advanced version of adaptive optics to obtain a cleaner, higher-resolution image than even the space-based Hubble Telescope could obtain! Have a look at the composite below to see what we’re talking about.
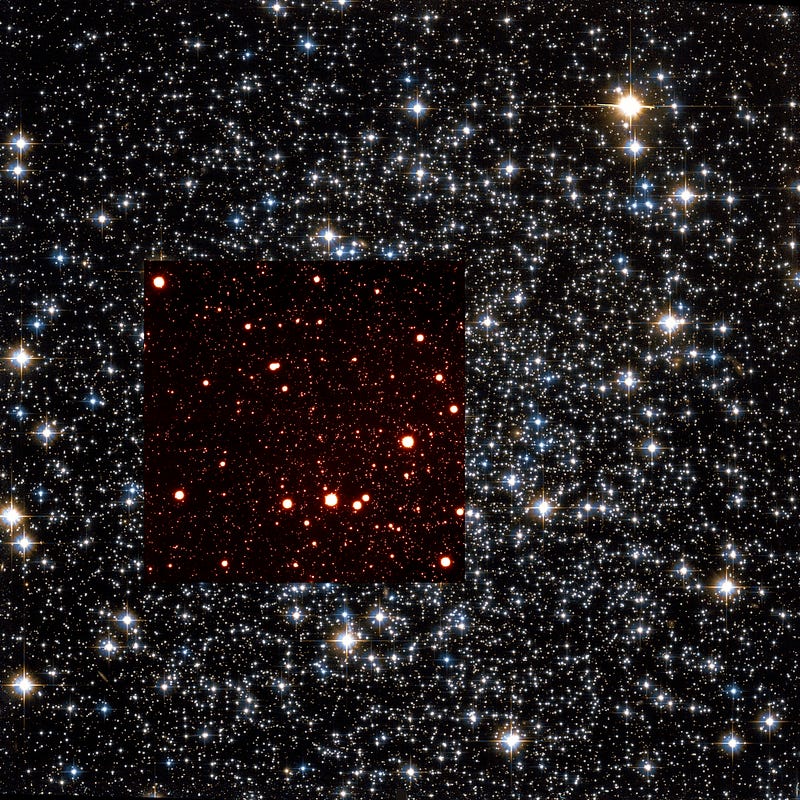
In a number of instances here, the Gemini image — taken from a ground-based 8.19-meter telescope outfitted with cutting-edge adaptive optics — outperforms the 2.4-meter Hubble Space Telescope which is in space! Have a look for yourself, and see if you can’t identify — side-by-side — a number of instances where Gemini uncovers stars that Hubble missed.
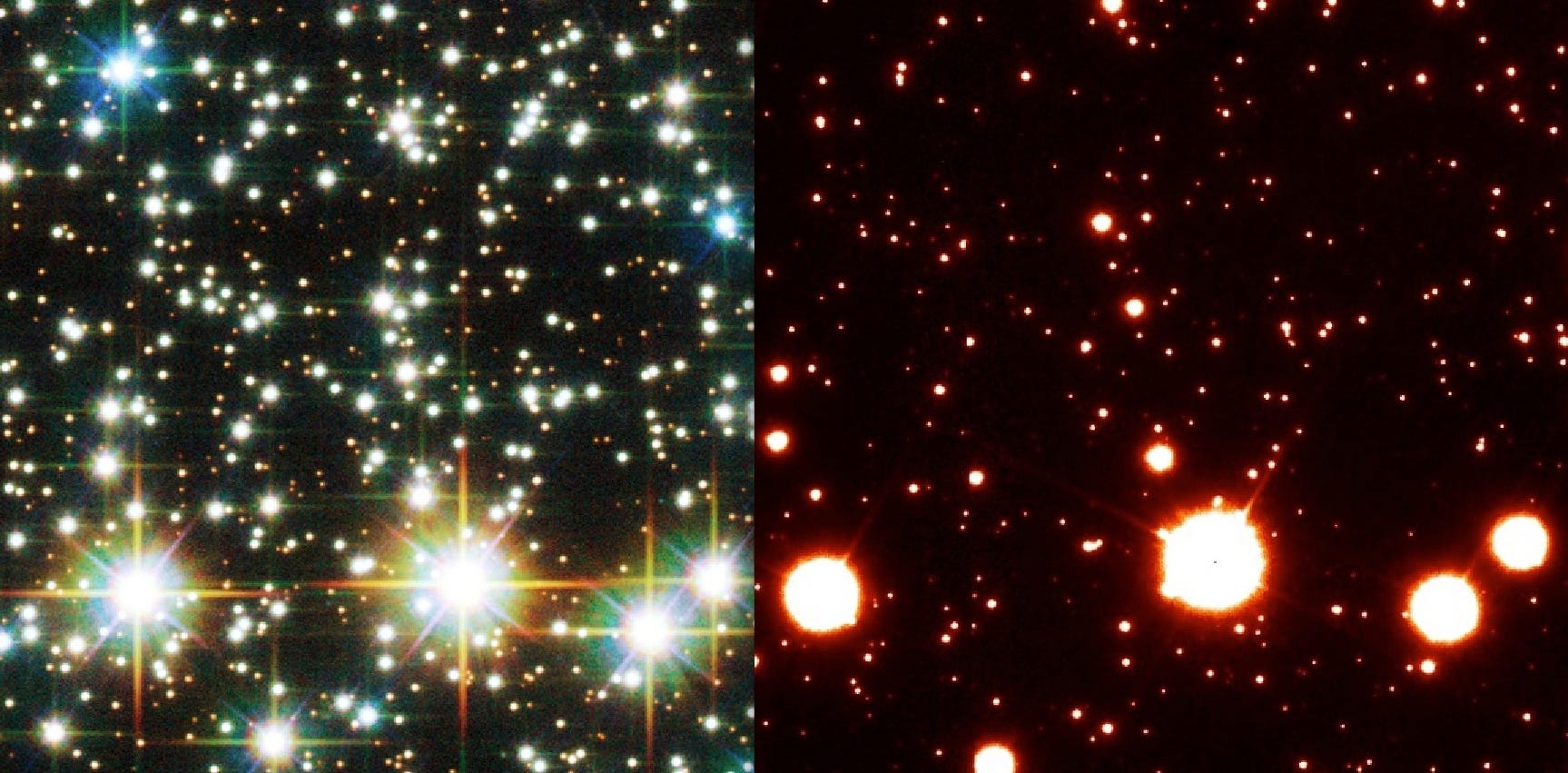
That was a view of the interior of globular cluster NGC 288, but adaptive optics systems on the Keck, Gemini and Lick observatories now routinely perform comparably to telescopes like Hubble that don’t even have to contend with the atmosphere!
The adaptive optics techniques have allowed us, for example, to look inside the Orion Nebula like never before.
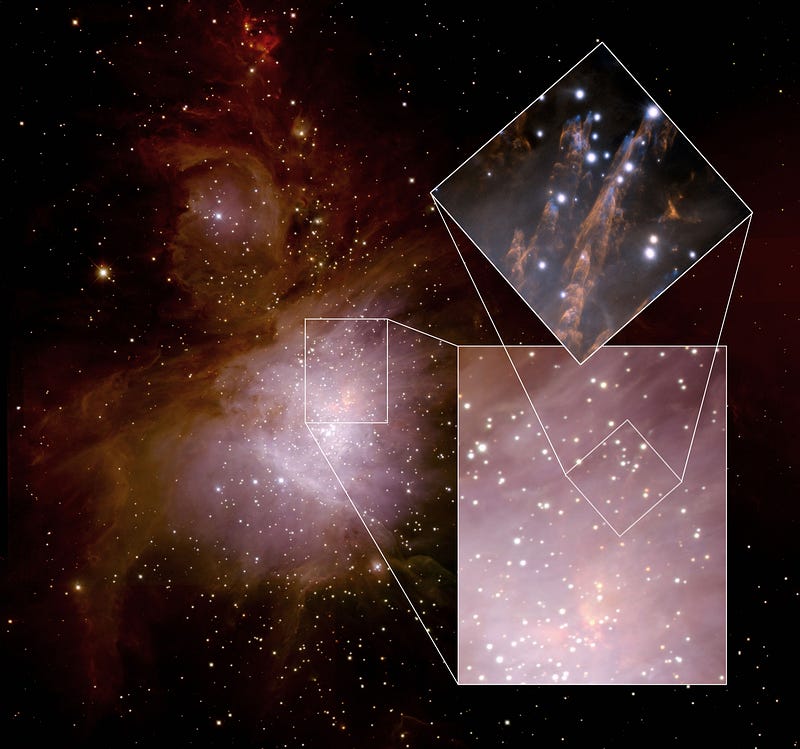
So the next time you see an observatory (or even an image of one) shooting a laser up at the Universe, there’s no need to pretend we’re fighting aliens, attacking a distant civilization, or beaming energy to a distant location.
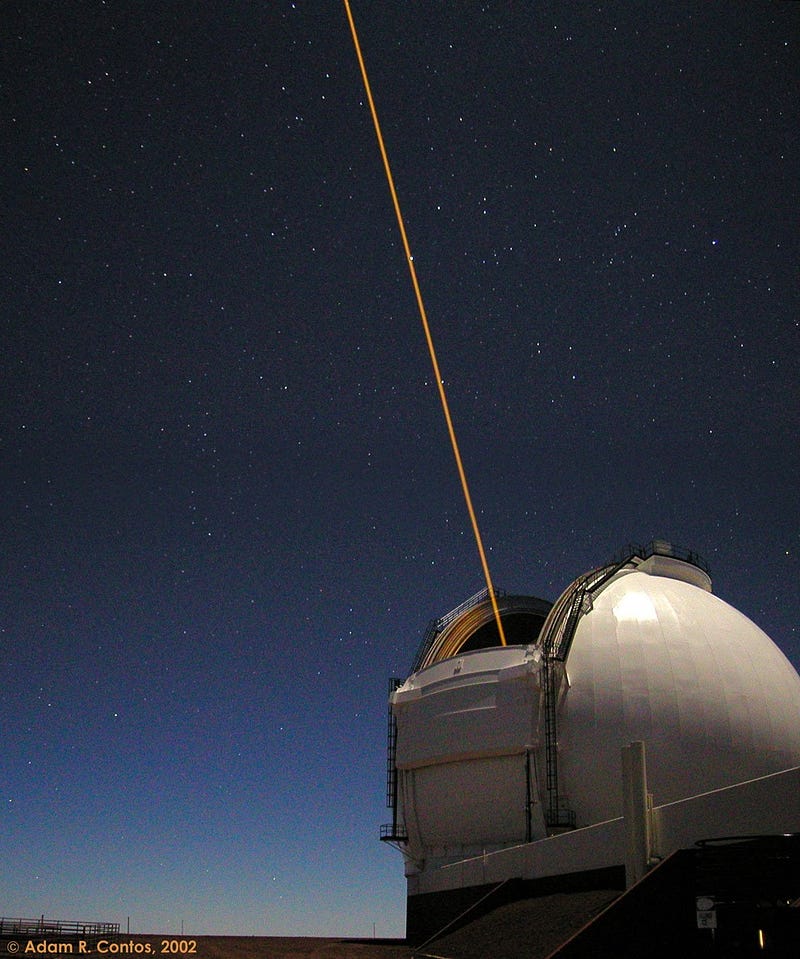
As is often the case with science, we’re actually doing something much more spectacular: we’re using our best technology, to the best of our abilities, to get the resolution of a space-based observatory, all without leaving the Earth!
Leave your comments at the Starts With A Bang forum on Scienceblogs!