Throwback Thursday: How Quantum Levitation Works
A little magnetism, a few impurities and liquid nitrogen make the magic happen!
“I see miracles all around me
Stop and look, it’s all astounding
Water, fire, air and dirt
Fucking magnets, how do they work?” –Insane Clown Posse
It might seem like there are mysteries in this world that science has no hope of explaining. But for everything we can’t explain, there are some absolutely amazing things we never would have tried if it weren’t for science, and the scientific predictions that our best theories have come to make!
For example, take a look at this video, and see if you can figure out what’s happening here. (No spoilers if you speak French!)
There’s clearly something going on with superconductivity, as the video (and article) title tell you, as well as some magnetism, too. But what’s the physics behind this incredible behavior?
Even if Insane Clown Posse doesn’t think we can, let’s see if we can’t get it right! And let’s do it by starting with the basic type of magnetism you all know: ferromagnetism.
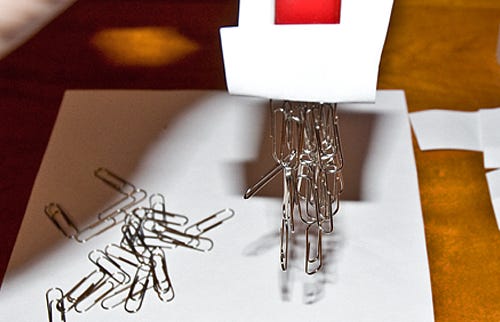
Ferromagnetism is how permanent magnets work, from iron blocks capable of picking up paper clips to the magnets sticking to your refrigerator. The basic principle is that you apply an external magnetic field, and not only does your ferromagnetic material wind up internally magnetized in the same direction as the external field, it remains magnetized even after that field is turned off!
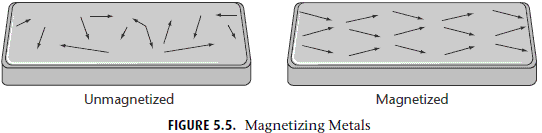
Although this is the type of magnet we’re most familiar with, nearly all materials are not ferromagnetic. Why not?
Because most materials don’t remain magnetized once that external field is removed. So what happens inside these other materials when you apply an external magnetic field? They are either diamagnetic, where they magnetize anti-parallel to the external field, or paramagnetic, where they magnetize parallel to the external field. (Incidentally, all materials exhibit diamagnetism, but some materials are either also paramagnetic or ferromagnetic, which can easily overwhelm the effect of diamagnetism.)
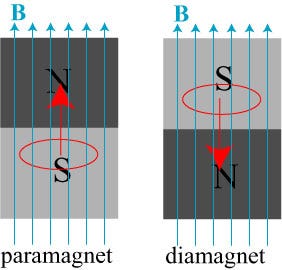
At normal temperatures, you’ve probably heard of the electromagnetic phenomenon of Faraday’s law of induction, which says that if you change the magnetic field inside of a material, it generates an internal, electrical current that works to oppose that change! Well, if you bring a material with any sort of conduction at all into or out of a magnetic field, you’re going to create tiny currents inside of the material — known as eddy currents — that oppose the internal change in the magnetic field.
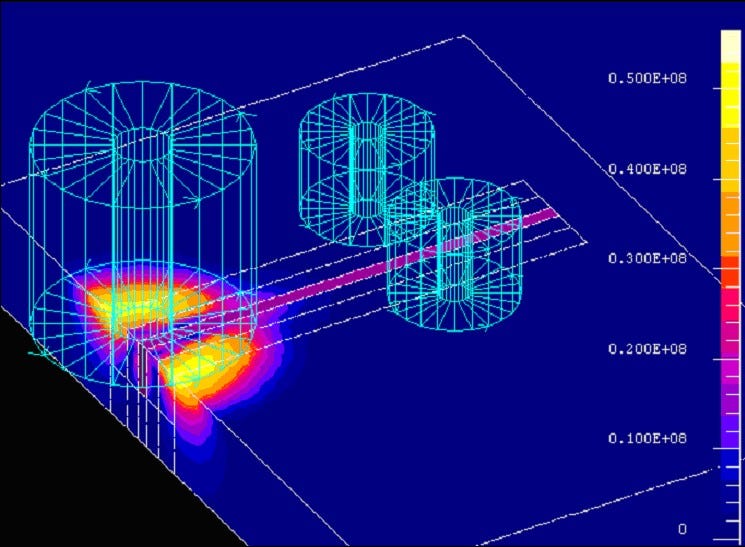
Now, at normal temperatures, these currents are extremely temporary, as they encounter resistance and decay away.
But what if you eliminated the resistance? What if you drove it down all the way to zero?
Believe it or not, you can drive the resistance down to zero in pretty much any material; all you have to do is bring it down to low enough temperatures, until it becomes a superconductor!
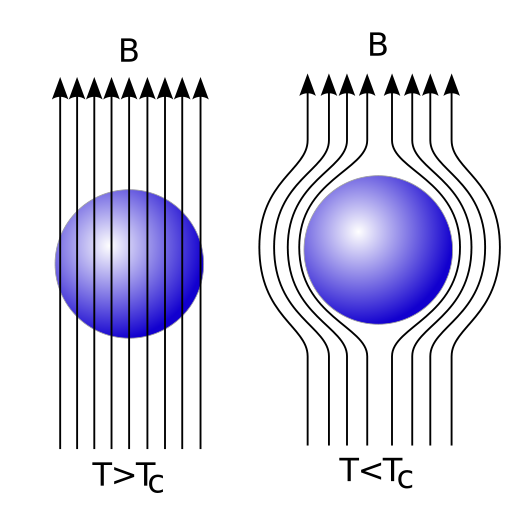
Every material has a critical temperature (labelled Tc, above), and when you cool that material below its critical temperature, is no longer has any resistance to electrical current at all. But just what is it that happens when you drop the temperature of a material below its critical temperature, to make it superconducting? It expels all the magnetic fields from inside! This is known as the Meissner Effect, and it turns a superconducting material into a perfect diamagnet.
“Hang on,” you may say, “how does that explain this quantum levitation?”
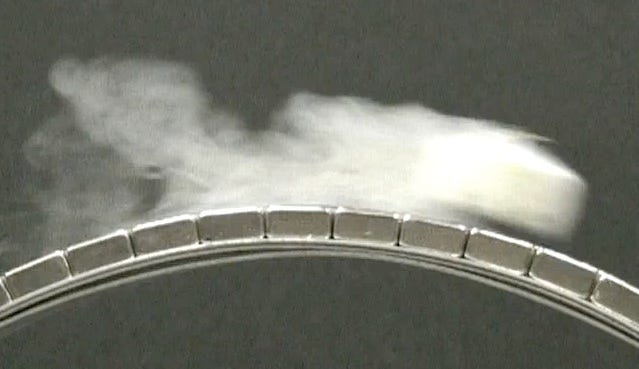
Well, it doesn’t, of course. Because what I just told you is for a Type I superconductor, like aluminum, lead, or mercury.
But there’s another type of superconductor, one with impurities in it, like the one in the French video I showed you earlier, and also in this amazing video, below.
If your material is an alloy, made of a mixture of materials, it can, first off, be made to superconduct at higher temperatures than any plain old element can on its own. In the late 1980s, scientists discovered that Yttrium Barium Copper Oxides (YBCOs) could begin superconducting at temperatures above 77 K for the first time, which means you can drop their resistance all the way down to zero using liquid nitrogen, which is cheap and easily available!
Alloys (and a few rare elements, like niobium, vanadium, and technetium) can also have a magnetic penetration depth that’s larger than their superconducting coherence length, which means that external magnetic field lines can run all the way through the material, even if the thing is superconducting! In this (relatively rare) case, the magnetic field will be expelled from everywhere in that material (the Meissner effect, remember?) except through these regions where the magnetic flux is “pinned” instead.

To sum up, in a Type II superconductor, the magnetic field lines can penetrate all the way through, from one end of the material to the other. And if the magnetic field can get through, guess what else it can do? Make those eddy currents! And with the resistance driven so far down (to zero, effectively) by these ultra-low temperatures, these currents don’t simply decay away; they’re sustained in perpetual motion, so long as the temperature remains low enough that the material remains superconducting! (Below about 93 K for YBCOs.)
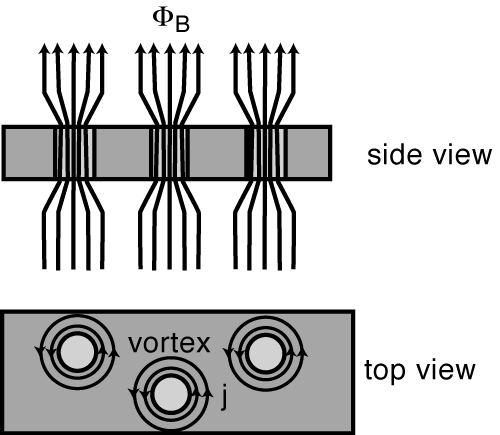
So in the regions where the fields are expelled, which is most of the material, you get a perfect diamagnet. In the regions where the flux is pinned, the magnetic field lines are concentrated, they pass all the way through the material and cause sustained eddy currents, and this is what pins the superconductor in place! (When you hear the term flux pinning, these confined field lines in the impure regions are what they’re talking about!)
So that’s it: you make the material (that’s a Type II superconductor with a critical temperature above liquid nitrogen temperatures) superconduct, you place it above a carefully-oriented magnetic track — like the one below — so that the magnetic flux is pinned through the superconductor and that it can only move along the track, and so long as you remain in a superconducting state, you’ll continue to levitate, thanks to this quantum phenomenon!
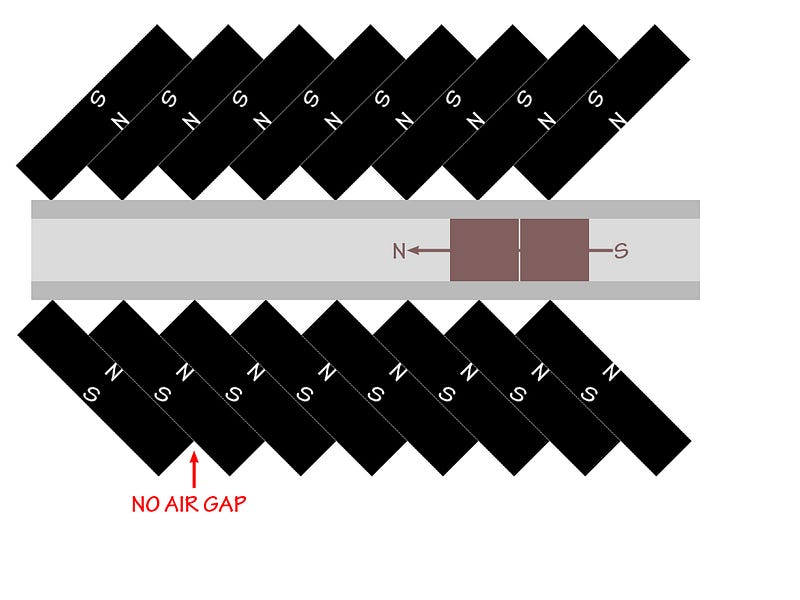
Way back in 2009, I happened to virtually cross paths with Matthew Sullivan of Ithaca College, who has applied this to create some amazing resources, including — for eye candy — the video below!
So not only do “we” understand magnetism (sorry, ICP, scientist’s aren’t lying), now you do, too! And that’s true even when applied to levitating, quantum superconductors. Now go forth and spread the knowledge, because it’s too good to not share it! Okay, and if you need one more hypnotic animation… here you go!
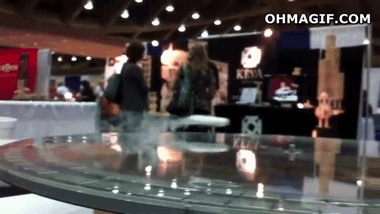
Enjoyed this? Leave a comment at the Starts With A Bang forum on Scienceblogs!