This Is Why We Will Never Know Everything About Our Universe
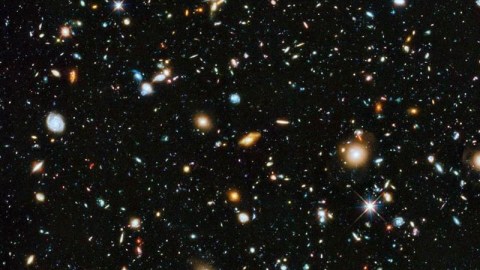
Even the best science imaginable has its limits.
In terms of ambition, it’s hard to ask for more than to know absolutely everything there is to know about the Universe. That’s the ultimate scientific dream: not just to understand the laws that govern reality as fully and deeply as possible, but to understand how every single particle in existence behaved from the moment of the Universe’s birth up through the present day.
But this dream isn’t necessarily one we’re capable of realizing, even with arbitrarily good equipment and ideal observational methods. As vast as the Universe is, the part of it that’s observable to us, both now and in the future, is still finite. With a finite number of particles and a finite amount of energy present within our observable Universe, the information we can glean is finite, too. Here’s what we know about the scientific limits of knowledge.
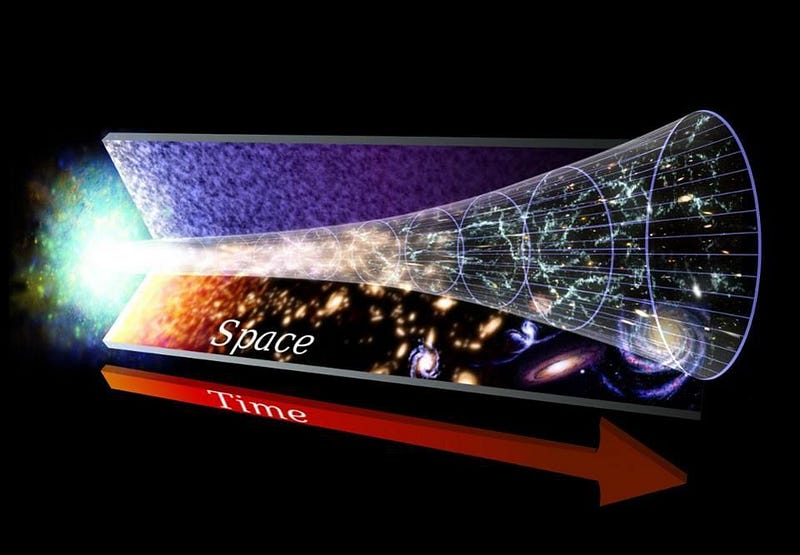
Think about the Big Bang, and the fact that the Universe we inhabit today arose from a hot, dense state that expanded and cooled. Think back to that moment in time, some 13.8 billion years ago. Even though the fabric of space itself is expanding, and even though light can move through space at the ultimate cosmic speed limit (the speed of light), there’s a limit to how far away we can see.
No matter how quickly the fabric of space expands, nor how fast the speed of light is, nor how much time has passed since the Big Bang, none of these properties are infinite. Therefore, we can only see a finite distance away, and there’s only going to be a finite amount of matter contained within the visible Universe. The amount of information we have access to is finite.
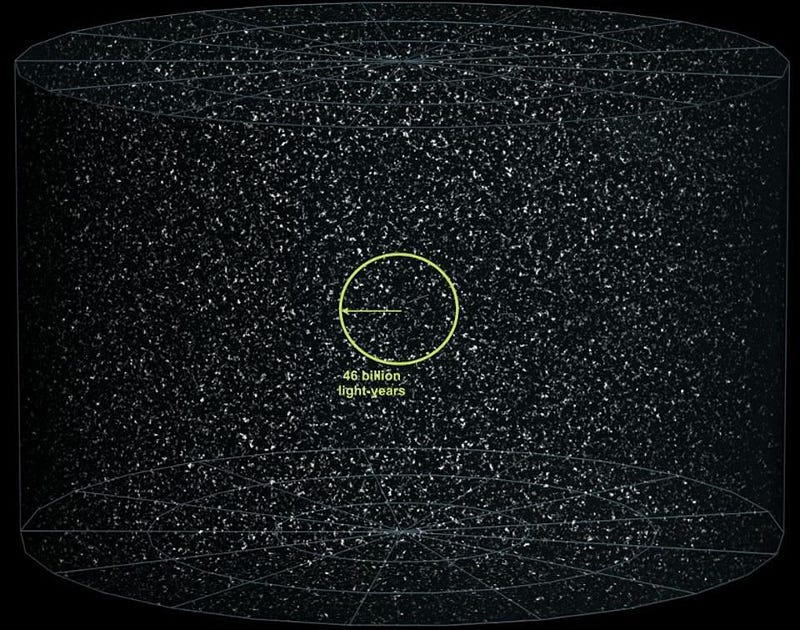
Many discoveries throughout our history have enabled us to better understand the Universe around us. Even though we don’t know everything, there are enormous sources of knowledge that have empowered us to draw far-reaching conclusions about our Universe. We know what it’s made up of in terms of matter, energy, radiation and so on.
We know how many stars are present within our galaxy (about 400 billion) and how many galaxies are present throughout the visible Universe (about 2 trillion). We know how the Universe clumps and clusters together into galaxy groups, clusters, and filaments, and how they’re separated by vast cosmic voids. We know the scale of the cosmic distances defining these structures, and how the Universe is evolving over time.
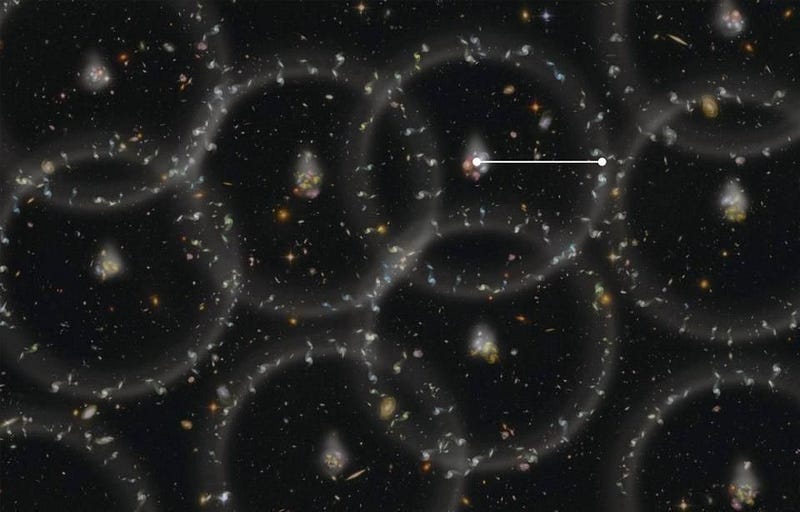
It’s a remarkable story that all fits together beautifully in the framework of the Big Bang and General Relativity. When we discovered that a galaxy’s measured distance was correlated with its apparent recession speed from us, it offered an intriguing and revolutionary possibility. Perhaps the galaxies were not all speeding away from our location, but the fabric of space itself was expanding.
If this were the case, then the Universe should not only be expanding but cooling, as the wavelength of light would be stretched to lower and lower energies as time went on. We should see a leftover glow with particular properties dating back to the earliest times: the cosmic microwave background. We should see an evolving web of cosmic structure. And we should see that the earliest clouds of gas should have specific ratios of light elements, with no heavy elements present at all.
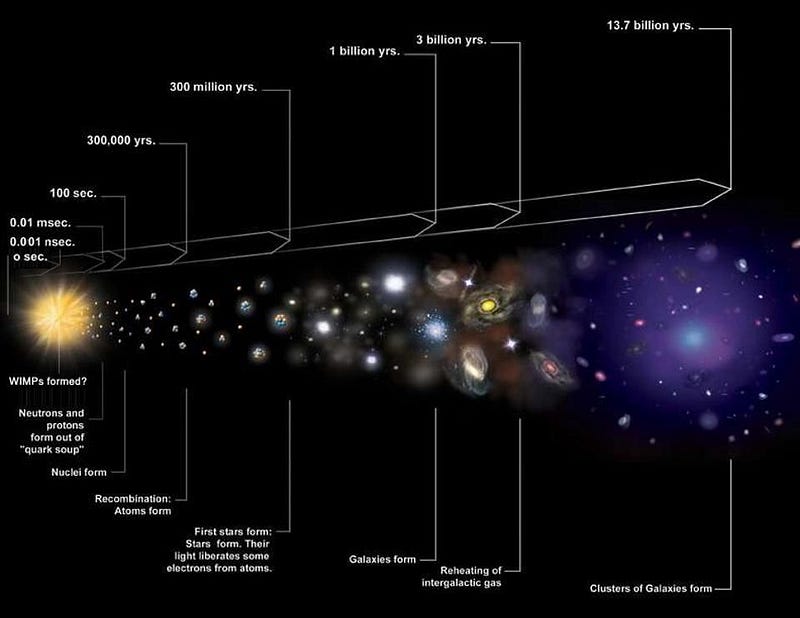
All of these predictions and a great many more have been made and verified about the early Universe. This has led to the current state of cosmic affairs, where we understand that our Universe began in a hotter, denser, more uniform and more rapidly-expanding state: this is what we know as the hot Big Bang.
It’s very tempting to claim that the Big Bang was, therefore, the beginning. You might then think that if we can understand the beginning, and the laws governing reality, we can know everything that’s occurred in all of existence. All we’d need to do is take the laws of physics and extrapolate. But when we naively extrapolate back to the earliest stages of the Universe and compare what we expect to what we observe, there are some major surprises.
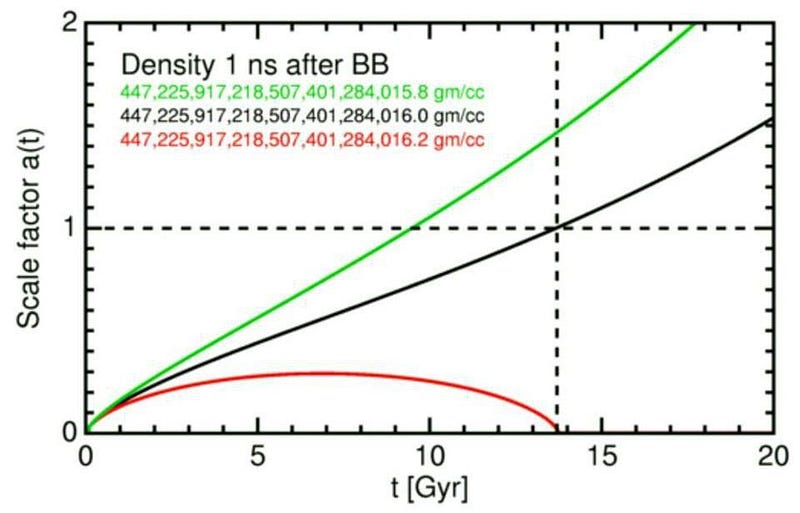
You see, there were a few major puzzles that arise if you try and go all the way back to the very beginning within the framework of an arbitrarily hot, dense state as the Universe’s beginning.
- The Universe would’ve expanded into oblivion or recollapsed almost immediately, never forming stars or galaxies, unless the initial expansion rate and the initial energy density were perfectly balanced.
- The Universe would have different temperatures in different directions — something it was observed not to have — unless something caused it to have the same temperature everywhere.
- The Universe would have been filled with high-energy relics that had never been detected, a consequence of extrapolating back arbitrarily in the past.
And yet, when we viewed our Universe, it did have stars and galaxies, it was the same temperature in all directions, and it didn’t have these high-energy relics.
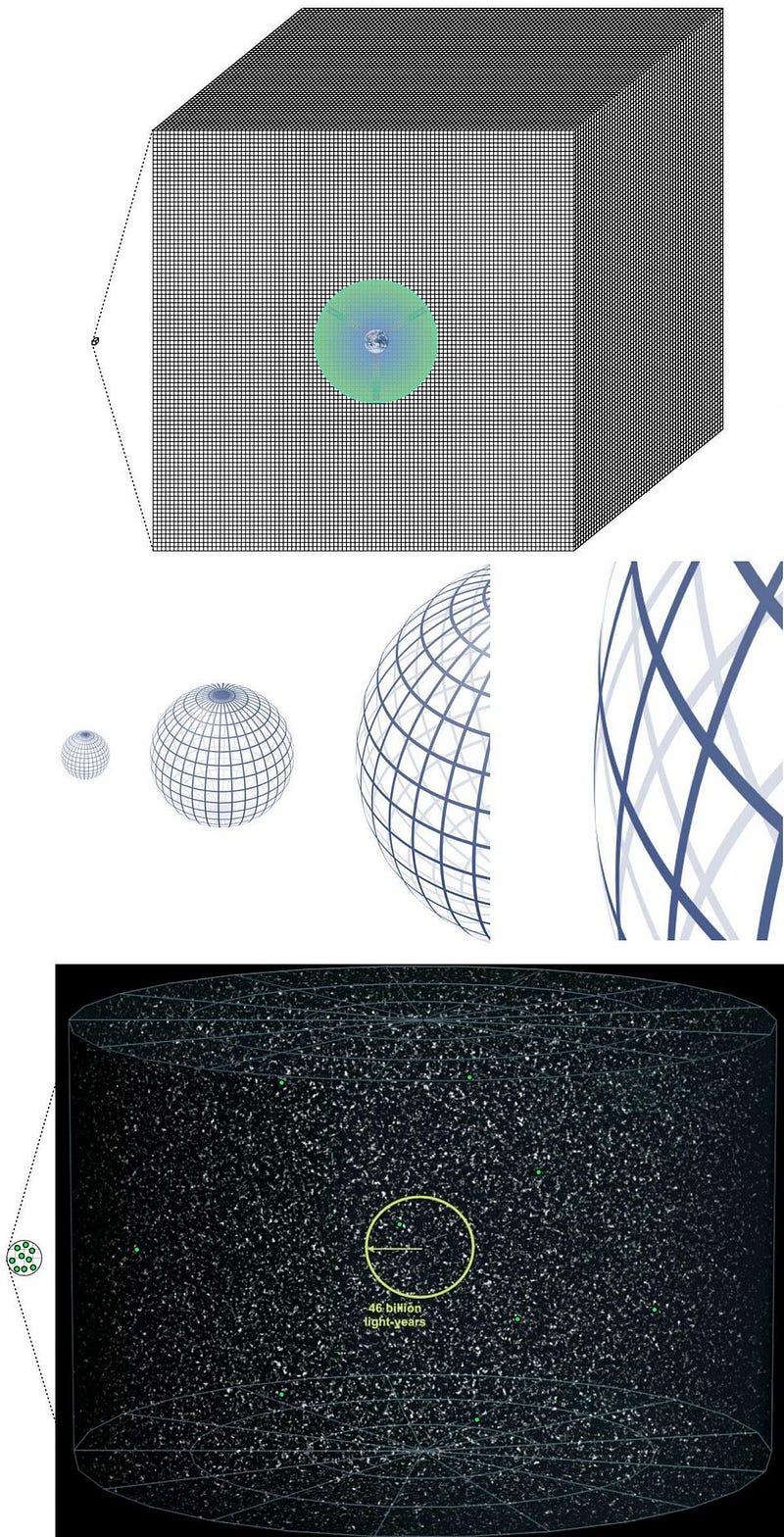
The solution to these problems was the theory of cosmic inflation, which replaced the idea of a singularity with a period of exponentially expanding space, and which predicted those initial conditions that the Big Bang on its own could not. In addition, inflation made six other predictions for what we would see in our Universe:
- A maximum temperature achieved in the hot Big Bang that’s well below the Planck energy scale.
- The existence of super-horizon fluctuations, or temperature/density fluctuations on scales larger than light could have traversed since the Big Bang.
- Density fluctuations that are 100% adiabatic and 0% isocurvature in nature.
- Almost-perfectly scale-invariant density fluctuations, but with slightly greater magnitudes on large scales than small ones.
- An almost-perfectly flat Universe, with quantum effects producing curvature at the 0.01% level or below.
- And a Universe filled with a primordial gravitational wave background, which should imprint itself on the Big Bang’s leftover glow.
The first five of them have been verified or validated to the best of our observational capabilities, while the sixth remains below our detection threshold.
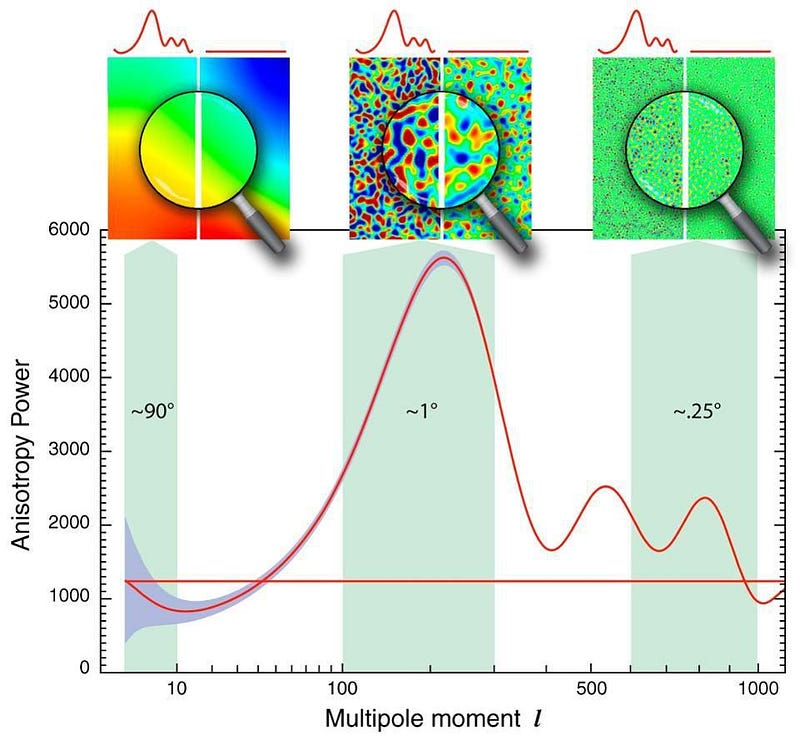
But now, we run into a problem. A big, existential, hold-the-phone type of problem as far as the idea of knowing everything about our existence is concerned. We were able to look at the Universe around us today and use the available evidence to construct the idea of the Big Bang, and then make new predictions to test the Big Bang for ourselves.
The unanswered problems and unexplained puzzles of the Big Bang paved the way for us to develop cosmic inflation, which reproduces the Big Bang’s successes, explained those puzzles, and then itself made new predictions with observable consequences.
All of that is a spectacular example of the successes of science. But it should leave you wanting more. The next logical question about our origins, of course, becomes this: where did cosmic inflation come from?
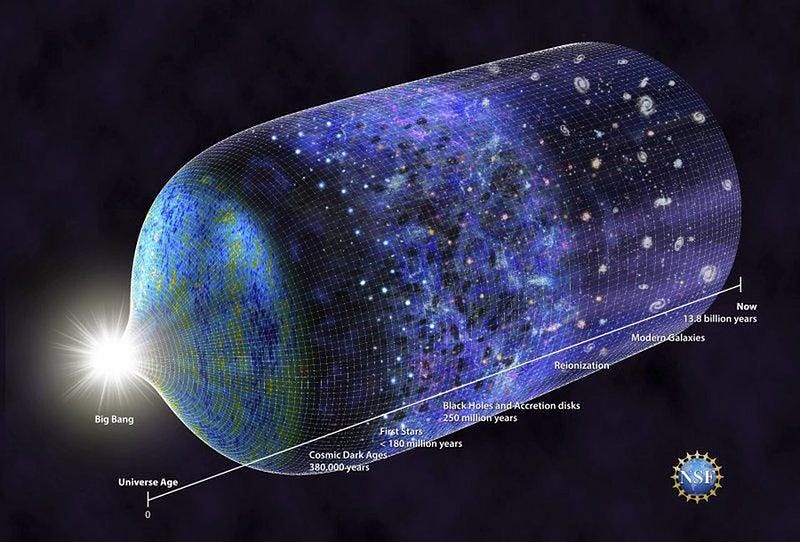
Was cosmic inflation a state that was eternal to the past, meaning that it had no origin and always existed, right up until the moment it ended and created the Big Bang?
Was inflation a temporary state that had a beginning, where it emerged from a non-inflationary spacetime some finite time in the past?
Was inflation a small part of a cyclical state, where time loops back on itself from some far future state where the Universe will begin inflating again?
These sound like interesting, difficult, and compelling questions, and intriguing possibilities, to boot. Surely, knowing where our Universe comes from involves not just stating the Big Bang, but knowing where the Big Bang came from. If the answer is cosmic inflation, then we’ll want to know where cosmic inflation came from.
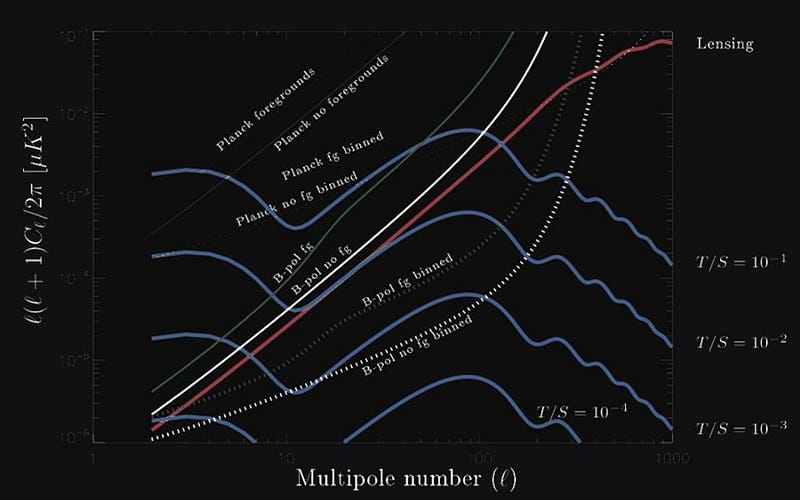
But we can’t know. This is where we run into the fundamental limits of information contained within the Universe, which is the only way we have to know anything about the Universe itself. There is nothing we can observe, in our Universe, that allows us to tell these three possibilities apart.
In all but the most contrived models of inflation (and some of those have already been ruled out), it’s only the last 10^-33 seconds or so of inflation that impacts our Universe. The exponential nature of inflation wipes out any information that occurred prior to that, separating it from anything we can observe by, well, inflating it beyond the portion of our Universe that we can observe.
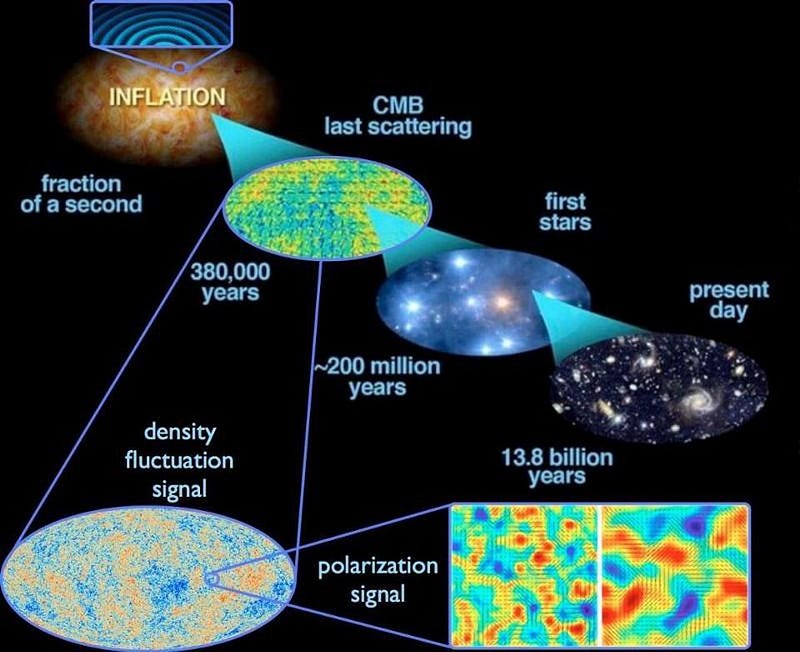
What we’re left with is an observable Universe that’s huge:
- 46 billion light-years in radius,
- containing some 2 trillion galaxies,
- a total of around 10²⁴ stars,
- 10⁸⁰ atoms,
- and nearly 10⁹⁰ photons.
The total amount of energy in all the particles, antiparticles, quanta of radiation, and even in empty space itself adds up to around 1054kilograms, including dark matter and dark energy.
But those astronomically-large numbers are still finite. Moreover, they contain no information about what happened in the Universe prior to the last tiny fraction-of-a-second of inflation. Most viable models of inflation would leave no testable, observable signature of inflation’s beginnings, and therefore we have no way of knowing how — or even if — the Universe started.
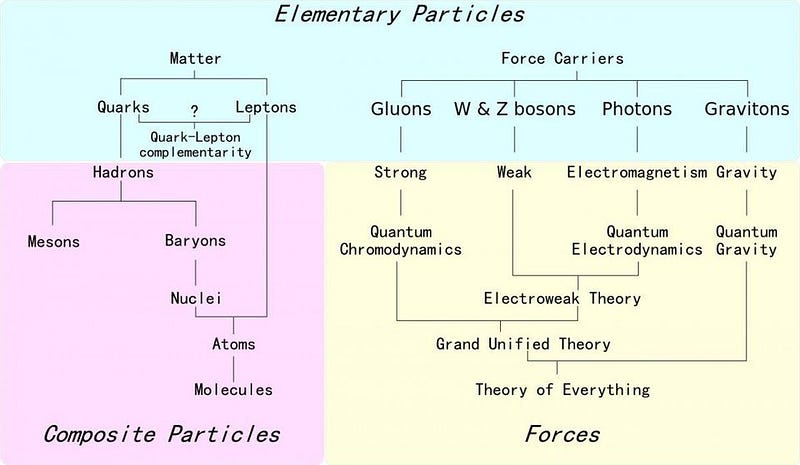
The total amount of information accessible to us in the Universe is finite, and hence, so is the amount of knowledge we can gain about it. There’s a limit to the amount of energy we can access, the particles we can observe and the measurements we can make. That doesn’t mean we’re done, or that we shouldn’t strive to learn everything we absolutely can. Only we can push the frontiers of knowledge back as far as they can go.
There’s a whole lot left to learn and a whole lot that science has yet to reveal. If we continue to look, many of the present unknowns will likely fall in the near future. But what is knowable is finite, and this implies that there are necessarily some things we may never know. The Universe may yet be infinite, but our knowledge of it never will be.
Ethan Siegel is the author of Beyond the Galaxy and Treknology. You can pre-order his third book, currently in development: the Encyclopaedia Cosmologica.