This Is What Will Happen To Our Sun After It Dies
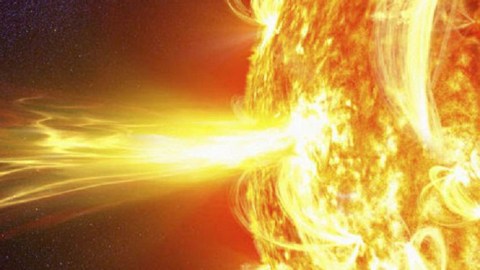
An entire Universe of possibilities await stars like our own, even after they run out of fuel.
One of the most profound rules in all the Universe is that nothing lasts forever. With gravitational, electromagnetic and nuclear forces all acting on matter, practically everything we observe to exist today will face changes in the future. Even the stars, the most enormous collections that transform nuclear fuel in the cosmos, will someday all burn out, including our Sun.
But this does not mean that stellar death — when stars run out of nuclear fuel — is actually the end for a star like our Sun. Quite to the contrary, there are a number of fascinating things in store for all stars once they’ve died that first, most obvious death. Although it’s true that our Sun’s fuel is finite and we fully expect it to undergo a “typical” stellar death, this death is not the end. Not for our Sun, and not for any Sun-like stars. Here’s what comes next.
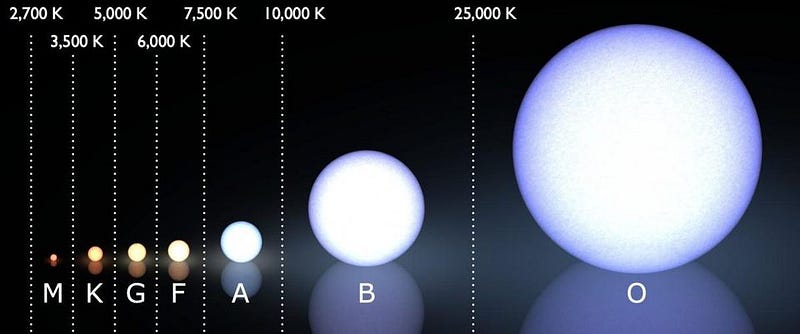
In order to be considered a true star, and not a failed star (like a brown dwarf) or some corpse (like a white dwarf or neutron star), you have to be capable of fusing hydrogen into helium. When a cloud of gas collapses to potentially form a new star, it has a lot of gravitational potential energy in its diffuse state, which gets converted into kinetic (thermal) energy when it collapses. This collapse heats up the matter, and if it gets hot and dense enough, nuclear fusion will begin.
After many generations of studying stars, including where they do and don’t form, we now know they have to reach an internal temperature of about 4 million K to begin fusing hydrogen into helium, and that requires at least ~8% the mass of our Sun, or about 70 times the mass of Jupiter. Being at least that massive is the minimum requirement for becoming a star at all.
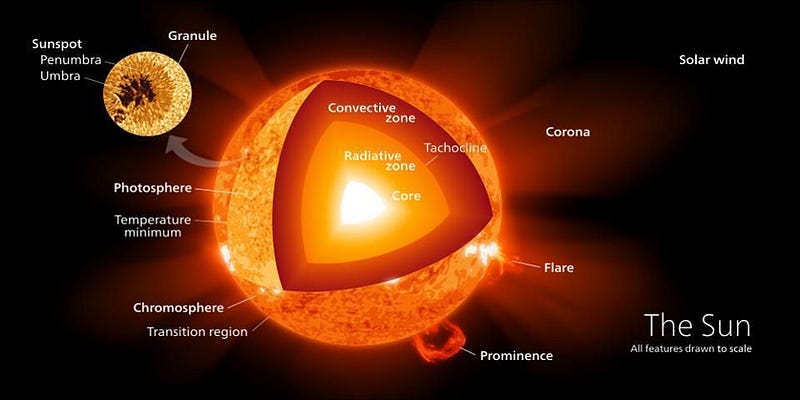
Once that mass/temperature threshold is crossed, the star begins fusing hydrogen into helium, and will encounter one of three different fates. These fates are determines solely by the star’s mass, which in turn determines the maximum temperature that will be reached in the core. All stars begin fusing hydrogen into helium, but what comes next is temperature-dependent. In particular:
- If your star is too low in mass, it will fuse hydrogen into helium only, and will never get hot enough to fuse helium into carbon. A purely helium composition is the fate of all M-class (red dwarf) stars, below about 40% the Sun’s mass. This describes the majority of stars in the Universe (by number).
- If your star is like the Sun, it will contract down to higher temperatures when the core runs out of hydrogen, beginning helium fusion (into carbon) when the star swells into a red giant. It will end composed of carbon and oxygen, with the lighter (outer) hydrogen and helium layers blown off. This occurs for all stars between about 40% and 800% the Sun’s mass.
- If your star is more than 8 times the mass of the Sun, it will not only fuse hydrogen into helium and helium into carbon, but will initiate carbon fusion later on, leading to oxygen fusion, silicon fusion, and eventually, a spectacular death by supernova.
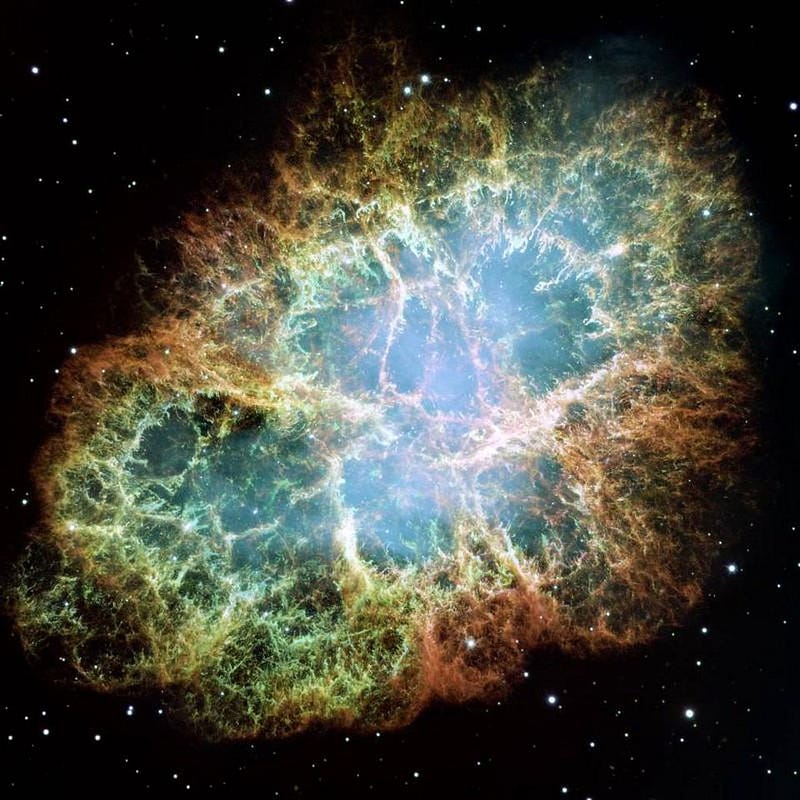
These are the most conventional fates of stars, and by far the three most common. The stars that are massive enough to go supernova are rare: only about 0.1–0.2% of all stars are this massive, and they will leave behind either neutron star or black hole remnants.
The stars that are lowest in mass are the most common star in the Universe, making up somewhere between 75–80% of all stars, and are also the longest-lived. With lifetimes that range from perhaps 150 billion to over 100 trillion years, not a single one has run out of fuel in our 13.8 billion year old Universe. When they do, they will form white dwarf stars made entirely out of helium.
But Sun-like stars, which comprise about a quarter of all stars, experience a fascinating death cycle when they run out of helium in their core. They transform into a planetary nebula/white dwarf duo in a spectacular, but slow, death process.
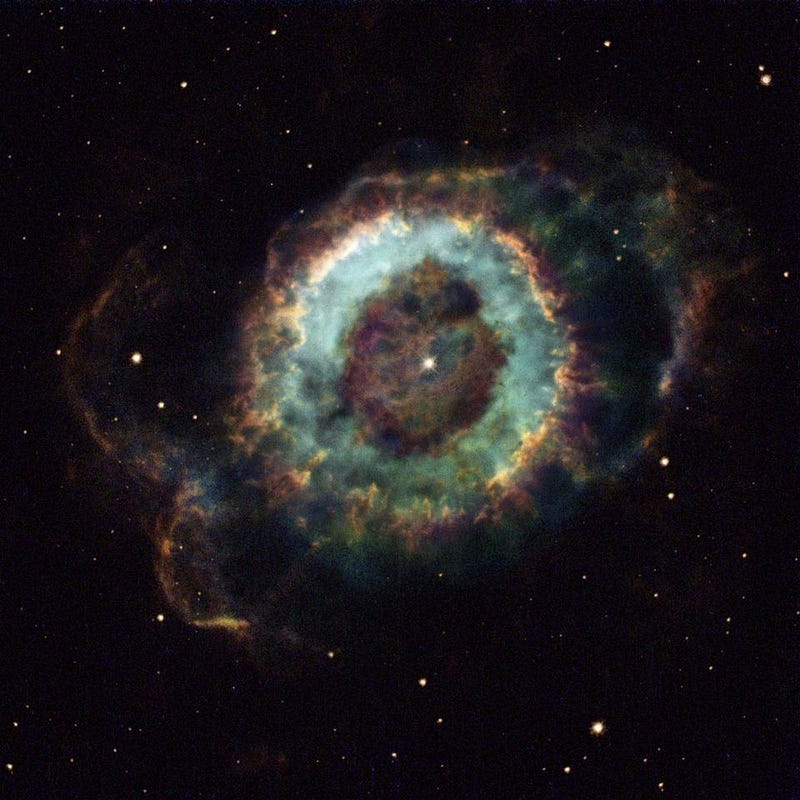
During the red giant phase, Mercury and Venus will certainly be engulfed by the Sun, while Earth may or may not, depending on certain processes that have yet to be fully worked out. The icy worlds beyond Neptune will likely melt and sublimate, and are unlikely to survive the death of our star.
Once the Sun’s outer layers are returned to the interstellar medium, all that remains will be a few charred corpses of worlds orbiting the white dwarf remnant of our Sun. The core, largely composed of carbon and oxygen, will total about 50% the mass of our present Sun, but will only be approximately the physical size of Earth.
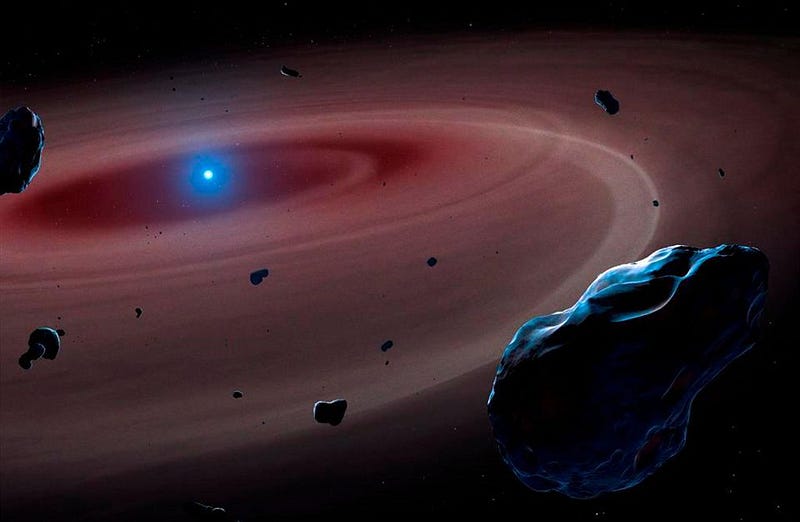
This white dwarf star will remain hot for an extremely long time. Heat is an amount of energy that gets trapped inside any object, but can only be radiated away through its surface. Imagine taking half the energy in a star like our Sun, then compressing that energy down into an even smaller volume. What will happen?
It will heat up. If you take gas in a cylinder and compress it rapidly, it heats up: this is how a piston in your combustion engine works. The red giant stars that give rise to white dwarfs are actually much cooler than the dwarf itself. During the contraction phase, temperatures increase from as low as 3,000 K (for a red giant) to up to about 20,000 K (for a white dwarf). This type of heating is due to adiabatic compression, and explains why these dwarf stars are so hot.
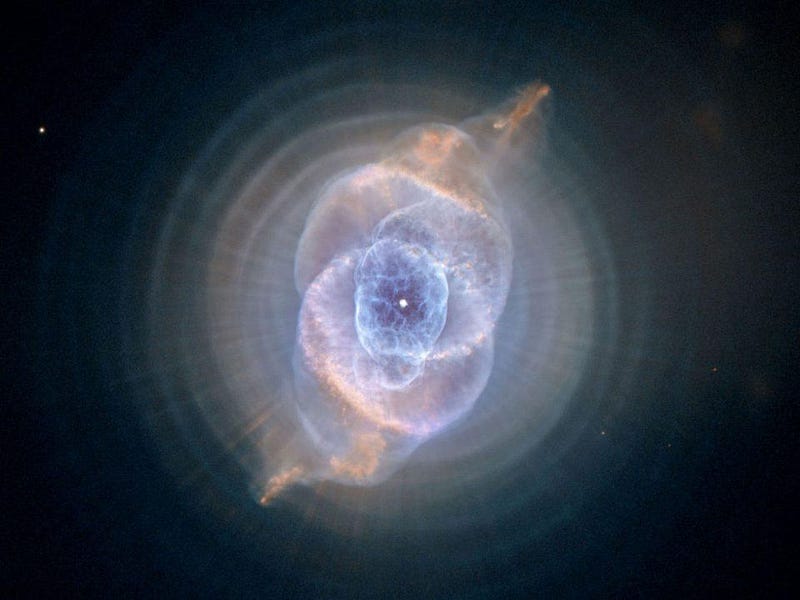
But now, it’s got to cool down, and it can only radiate away through its small, tiny, Earth-sized surface. If you were to form a white dwarf right now, at 20,000 K, and give it 13.8 billion years to cool down (the present age of the Universe), it would cool down by a whopping 40 K: to 19,960 K.
We’ve got a terribly long time to wait if we want our Sun to cool down to the point where it becomes invisible. However, once our Sun has run out of fuel, the Universe will happily provide ample amounts of time. Sure, all the galaxies in the Local Group will merge together; all the galaxies beyond will accelerate away due to dark energy; star formation will slow to a trickle and the lowest-mass red dwarfs will burn through their fuel. Still, our white dwarf will continue to cool.
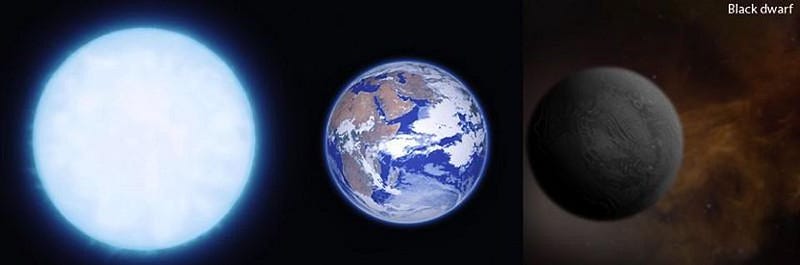
At last, after somewhere between 100 trillion and 1 quadrillion years (10¹⁴ to 10¹⁵ years) have passed, the white dwarf that our Sun will become will fade out of the visible part of the spectrum and cool down to just a few degrees above absolute zero. Now known as a black dwarf, this ball of carbon and oxygen in space will simply zip through whatever becomes of our galaxy, along with over a trillion other stars and stellar corpses left over from our Local Group.
But that isn’t truly the end for our Sun, either. There are three possible fates that await it, depending on how lucky (or unlucky) we get.
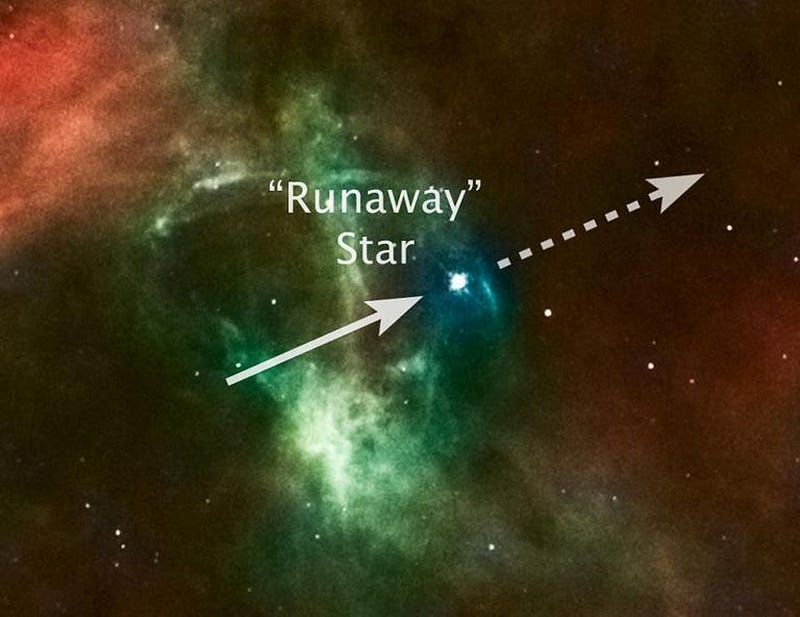
1.) Completely unlucky. About half of all stellar corpses in the galaxy — in most galaxies — originate as singlet star systems, much like our own Sun. While multi-star systems are common, with approximately 50% of all known stars found in binary or trinary (or even richer) systems, our Sun is the only star in our own Solar System.
This is hugely important for the future, because it makes it extraordinarily unlikely that our Sun will merge with a companion, or to swallow a companion or be swallowed by another companion. We’d be defying the odds if we merged with another star or stellar corpse out there. Assuming that we don’t get lucky, all our Sun’s corpse will see in the future is countless gravitational interactions with the other masses, which ought to culminate in what’s left of our Solar System getting ejected from the galaxy after approximately 10¹⁷ to 10¹⁹ years.
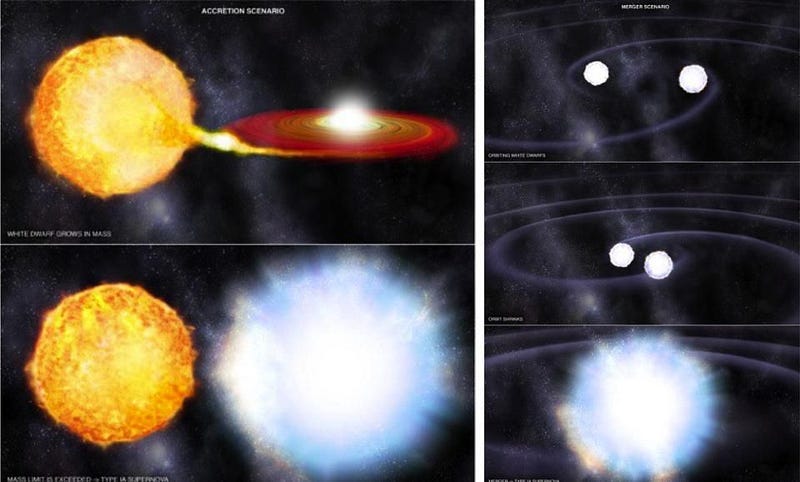
2.) Lucky enough to revitalize. You might think, for good reason, that once the white dwarf that our Sun becomes cools off, there’s no chance for it to ever shine again. But there are many ways for our Sun to get a new lease on life, and to emit its own powerful radiation once again. To do so, all it needs is a new source of matter. If, even in the distant future, our Sun:
- merges with a red dwarf star or a brown dwarf,
- accumulates hydrogen gas from a molecular cloud or gaseous planet,
- or runs into another stellar corpse,
it can ignite nuclear fusion once again. The first scenario will result in at least many millions of years of hydrogen burning; the second will lead to a burst of fusion known as a nova; the last will lead to a runaway supernova explosion, destroying both stellar corpses. If we experience an event like this before we get ejected, our cosmic luck will be on display for everyone remaining in our galaxy to witness.
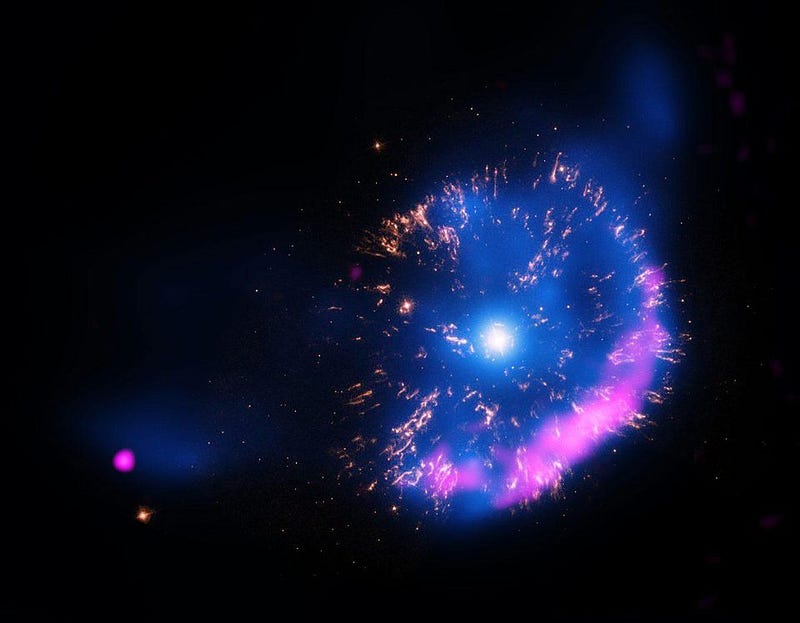
3.) Super lucky, where we’ll get devoured by a black hole. In the outskirts of our galaxy, some 25,000 light-years from the supermassive black hole occupying our galactic center, only the small black holes formed from individual stars exist. They have the smallest cross-sectional area of any massive object in the Universe. As far as galactic targets go, these stellar-mass black holes are some of the hardest objects to hit.
But occasionally, they do get hit. Small black holes, when they encounter matter, accelerate and funnel it into an accretion flow, where some fraction of the matter gets devoured and added to the black hole’s mass, but most of it gets ejected in the form of jets and other debris. These active, low-mass black holes are known as microquasars when they flare up, and they’re very real phenomena.
Although it’s exceedingly unlikely to happen to us, someone’s got to win the cosmic lottery, and those who do will become black hole food for their final act.
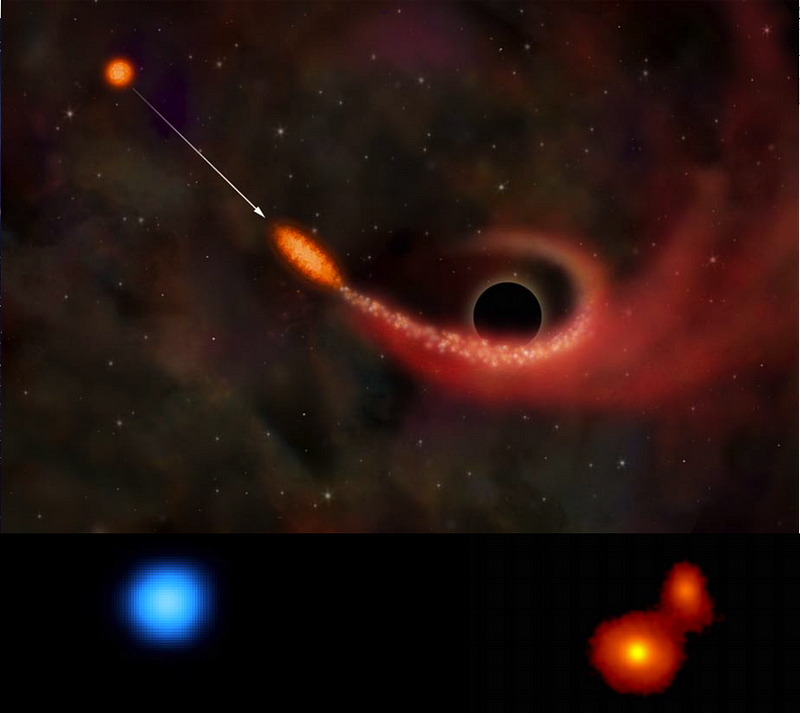
Almost every object in the Universe has a large set of possibilities as far as what’s going to happen to it in the far future, and it’s incredibly difficult to determine a single object’s fate given the chaotic environment of our corner of the cosmos. But by knowing the physics behind the objects we have, and understanding what the probabilities and timescales for each type of object is, we can better estimate what anyone’s fate should be.
For our Sun, we’re going to become a white dwarf after less than another 10 billion years, will fade to a black dwarf after ~10¹⁴-10¹⁵ years, and will get ejected from the galaxy after 10¹⁷-10¹⁹ years. At least, that’s the most probable path. But mergers, gas accumulation, collisions, or even getting devoured are all possibilities too, and they’ll happen to someone, even if it’s probably not us. Our future may not yet be written, but we’d be smart to bet on a bright one for trillions of years to come!
Ethan Siegel is the author of Beyond the Galaxy and Treknology. You can pre-order his third book, currently in development: the Encyclopaedia Cosmologica.