The Universe’s Dark Ages May Hold The Secrets To Dark Matter, Inflation, And Even String Theory
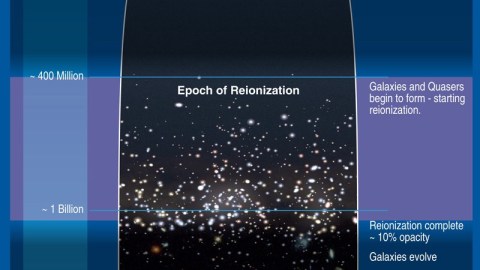
How the future of astronomy — and something we can’t even see — might open up the dark Universe.
This post is written by Sabine Hossenfelder, a theoretical physicist specialized in quantum gravity & high energy physics. She also freelance writes about science.
“When their eyes grew dim with looking at unrevealing dials and studying uneventful graphs, they could step outside their concrete cells and renew their dull spirits in communion with the giant mechanism they commanded, the silent, sensing instrument in which the smallest packets of energy, the smallest waves of matter, were detected in their headlong, eternal flight across the universe.” –James Gunn, on Radio Astronomy
The universe might have started with a bang, but once the echoes faded it took quite some while until the cosmic symphony began. Between the creation of the cosmic microwave background (CMB), where neutral atoms formed for the first time, and the formation of the first stars, there were 100 million years that passed in complete darkness. This “dark age” has so far been entirely hidden from observation, but this situation is soon to change.
The dark ages may hold the answers to many pressing questions. During this period, most of the universe’s mass was in form of light atoms — primarily hydrogen — and dark matter. The atoms slowly clumped under the influence of gravitational forces, until they finally ignited the first stars.
Before the first stars, astrophysical processes were few, and so the distribution of hydrogen during the dark ages carries very clean information about structure formation. Details about both the behavior of dark matter and the sizes of the structures that formed are encoded in these hydrogen clouds. But how can we see into this darkness?
Luckily, these dark ages weren’t entirely dark, just very, very dim. Back then, the hydrogen atoms that filled the universe frequently bumped into each other, which can flip the electron’s spin. If a collision flips the spin, the electron’s energy changes by a tiny amount because the energy depends on whether the electron’s spin is aligned with the spin of the nucleus or whether it points in the opposite direction. This very small energy difference is known as “hyperfine splitting.” Flipping the hydrogen electron’s spin from the aligned state to the anti-aligned one therefore leads to the emission of a very low energy photon. Since high energy means short wavelengths and low energy is long wavelength, you’ll be unsurprised to learn this hyperfine transition produces photons with a wavelength of 21cm. If we can trace the emission of these 21cm photons, we can trace the distribution of hydrogen. But 21 cm is the wavelength of the photons at the time of emission, which was some 13 billion years ago.
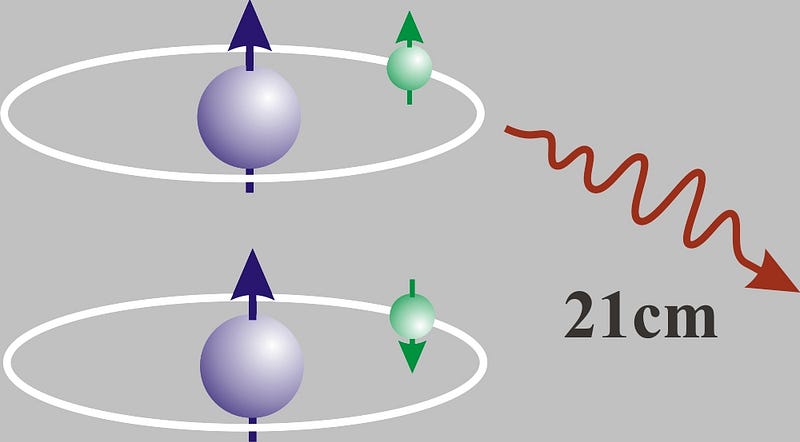
Since that time, the universe has expanded significantly and stretched the photons’ wavelength with it. How much the wavelength has been stretched depends on whether it was emitted early or late during the dark ages. The early photons have meanwhile been stretched by a factor of about 1000, resulting in wavelengths of a few hundred meters. Photons emitted towards the end of the dark ages have not been stretched quite as much — they today have wavelengths of “only” a few meters.
The most exciting aspect of 21cm astronomy is that it does not only give us a snapshot at one particular moment — like the CMB — but allows us to continuously map different epochs during the dark ages. By measuring the red-shifted photons at different wavelengths, we can scan through the entire time period. This would give us many new insights about the history of our universe.
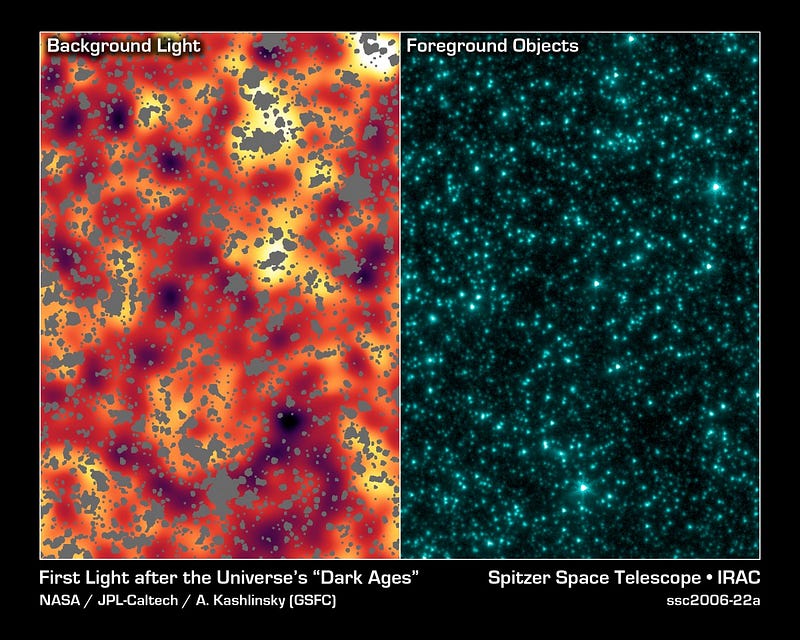
To begin with, it is not well understood how the dark ages end and the first stars are formed. The dark ages fade away in a phase of reionization, in which the intense UV starlight strips the neutral hydrogen of its electrons once again. This reionization is believed to be caused by radiation from the first stars, but we don’t know exactly what the intricacies of this process are. Since ionized hydrogen can no longer emit the hyperfine line, 21cm astronomy could tell us how the ionized regions grow, teaching us much about the early stellar objects and the behavior of the intergalactic medium. 21 cm astronomy can also help solve the riddle of dark matter. If dark matter self-annihiliates, this affects the distribution of neutral hydrogen, which can be used to constrain or rule out dark matter models.
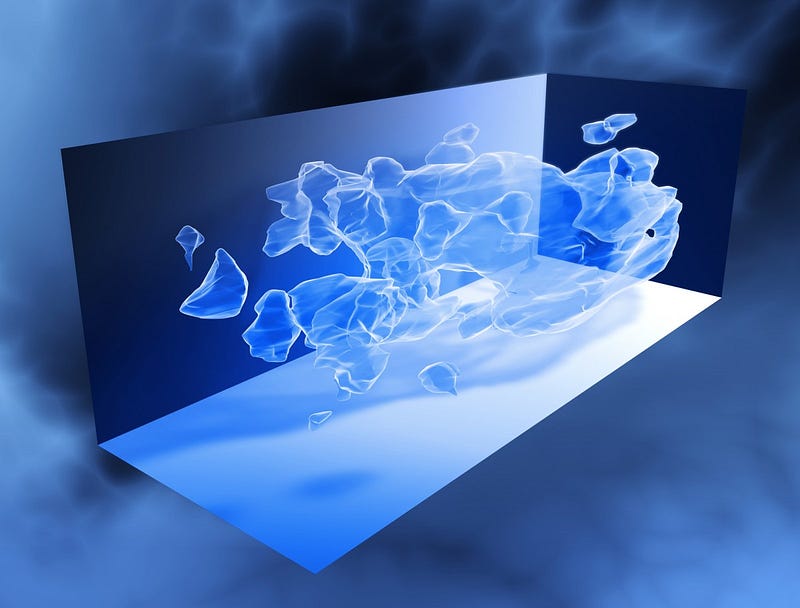
Inflation models too can be probed by this method: The distribution of structures that 21cm astronomy can map carries an imprint of the quantum fluctuations that caused them. These fluctuations in return depend on the type of inflation fields and the shape of those fields’ potentials. Thus, the correlations in the structures which were present already during the dark ages let us narrow down what type of inflation has occurred.
Perhaps most excitingly, the dark ages might give us a peek at cosmic strings, one-dimensional objects with a high density and high gravitational pull. In many models of string phenomenology, cosmic strings can be produced at the end of inflation, before the dark ages begins. By distorting the hydrogen clouds, the cosmic strings would leave a characteristic signal in the 21cm emission spectrum.
But measuring photons of this wavelength is not easy. The Milky Way, too, has sources that emit in this regime, which gives rise to an unavoidable galactic foreground that must be understood and subtracted. In addition, the Earth’s atmosphere distorts the signal and some radio broadcasts can interfere with the measurement. Nevertheless, astronomers have risen to the challenge and the first telescopes hunting for the early universe’s 21cm signal are now in operation.
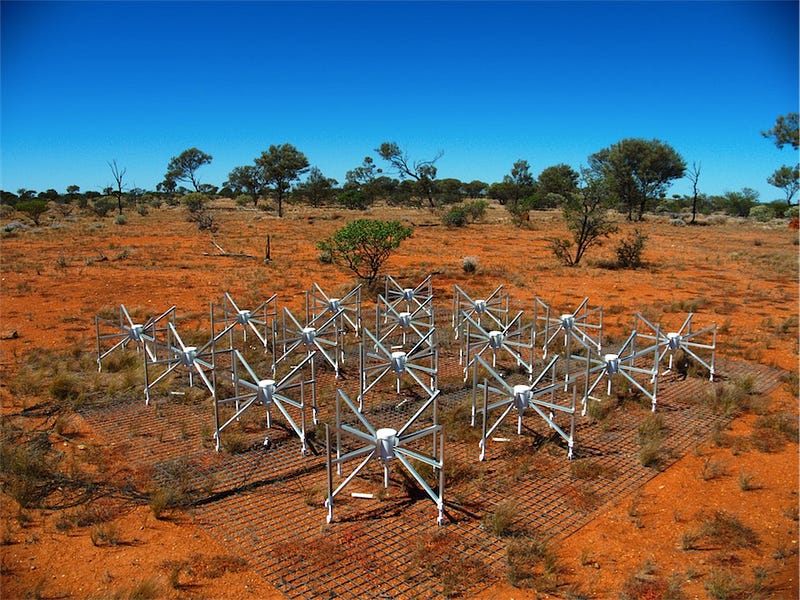
The Low-Frequency Array (LOFAR) went online in late 2012. Its main telescope is located in the Netherlands, but it combines data from 24 other telescopes in Europe, and is sensitive to wavelengths up to 30m in size. The Murchison Widefield Array (MWA) in Australia, which is sensitive to wavelengths of a few meters, has started taking data in 2013. And in 2025, the Square Kilometer Array is scheduled to be completed. This joint project between Australia and South Africa will be the world’s largest radio telescope.
Still, the astronomers’ dream would be to get rid of the distortion caused by Earth’s atmosphere altogether. Their most ambitious plan is to put an array of telescopes on the far side of the Moon. But this idea is, unfortunately, still far-fetched — for not to mention underfunded.
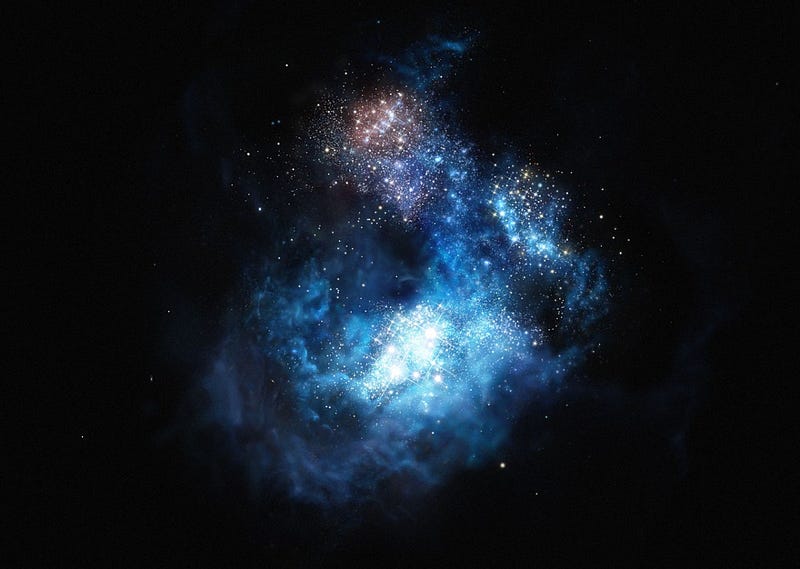
Only a few decades ago, cosmology was a discipline so starved of data that many argued it was closer to philosophy than to science. Today, it is a research area based on high precision measurements with a wealth of data covering the entire electromagnetic spectrum. The progress in technology and in our understanding of the universe’s history has been nothing but stunning, but we have only just begun. The dark ages are next.
Leave your comments on our forum, and check out our first book: Beyond The Galaxy, available now, as well as our reward-rich Patreon campaign!