The Cosmic Gift Of Neutron Stars
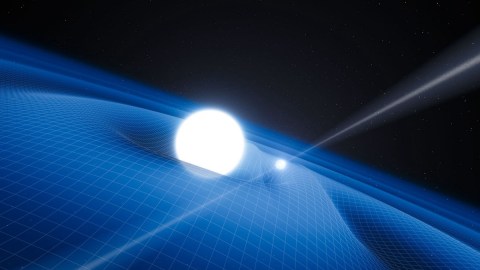
An incredible live-blog of an incredible event.
“It’s becoming clear that in a sense the cosmos provides the only laboratory where sufficiently extreme conditions are ever achieved to test new ideas on particle physics. The energies in the Big Bang were far higher than we can ever achieve on Earth. So by looking at evidence for the Big Bang, and by studying things like neutron stars, we are in effect learning something about fundamental physics.” –Martin Rees
If you take normal matter — something made of protons, neutrons and electrons — and compress it as far as it will go, something incredible happens. At high enough temperatures and densities, something requiring a tremendous amount of mass hundreds of thousands of times as great as planet Earth, nuclear fusion occurs, giving rise to a living star. Burn through all the hydrogen, though, and your star’s core will be made of helium, which will collapse further and heat up to even higher temperatures and densities. Reach a critical temperature and helium will be begin burning, forming carbon. After some time, you’ll run out of helium, too, where your now-carbon core begins to contract, heating up and becoming more dense. At this stage, one of two critical things can occur.
Either your star isn’t massive enough to ignite carbon, in which case it will gently blow off its outer layers and form a white dwarf at the center: a degenerate mass of atoms that’s maybe the mass of the Sun but only the physical size of Earth. This sounds like an incredible state of matter, but it’s still relatively sparse, at “only” a few hundred thousand times the density of our planet. The atoms themselves are sufficient to prevent gravitational collapse from taking things further.
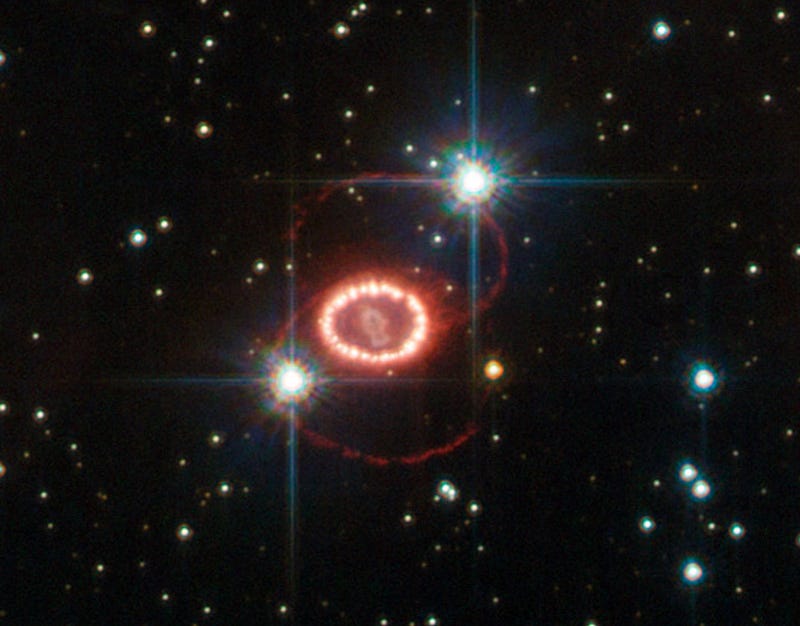
But if your star is massive enough to ignite carbon, the next sequence of events is inevitable:
- carbon will fuse into oxygen until the inner core runs out of carbon,
- the oxygen core will contract, heat up and ignite, fusing into silicon and sulphur,
- the silicon/sulphur core will contract, heat up and ignite, fusing into iron, cobalt and nickel,
- where the iron, cobalt and nickel core can ignite no further, and undergoes a spontaneous collapse event.
Depending on how massive the core is, it will either collapse all the way down to a black hole, or — for the vast majority of stars that undergo this process — the atoms themselves will transition from being protons, neutrons and electrons to being a ball of pure neutrons alone.
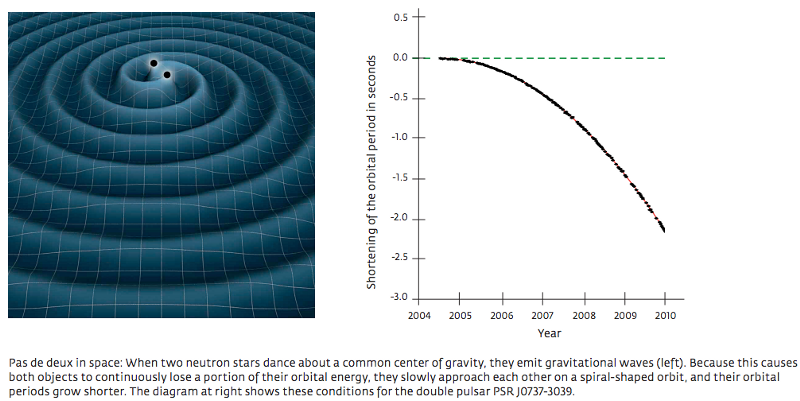
These massive, collapsed entities are neutron stars, coming in at up to three times the mass of our Sun, yet are no bigger than a large city like Washington, D.C. They are some of the most extreme objects in our Universe, and they enable us to explore some amazing things:
- They allow us to examine the limits of special and general relativity: they rotate at up to 2/3 the speed of light!
- These are the densest objects possible before becoming a black hole: more than a solar mass’ worth of material in a volume just a few kilometers wide!
- Some neutron stars pulse, up to nearly 1,000 times per second, creating the most perfect natural clocks in the Universe.
- Neutron stars in binary systems emit gravitational radiation and their orbits decay, one of the most important and stringent tests of strong-field general relativity due to the incredibly curved space around them.
- Colliding neutron stars not only create black holes, but they create the majority of many of the heaviest elements — gold, platinum, mercury and palladium, among others — in our Universe today.
- And they emit over the full spectrum of electromagnetic radiation thanks to magnetic fields more than a trillion times as strong as the Sun’s: from radio waves all the way up to gamma rays, including from sources at the galaxy’s center!
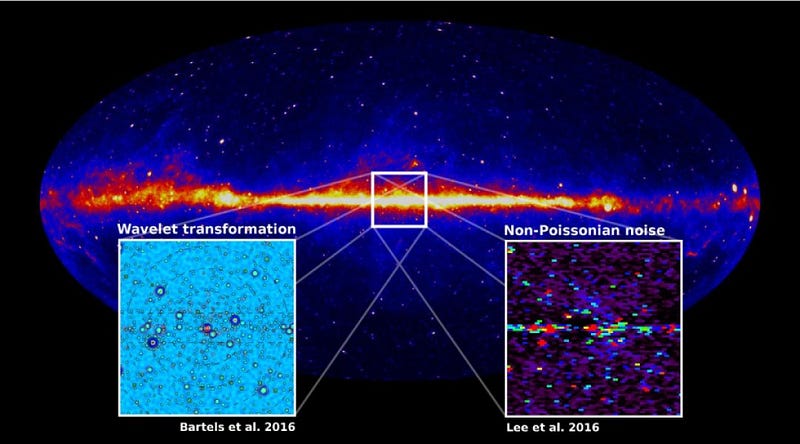
These objects hold clues and possibly the keys to a great many cosmic secrets, which is why I’m delighted that Vicky Kaspi of McGill gave last week’s public lecture at Perimeter Institute.
The lecture started at 7:00 PM ET / 4:00 PM PT last Wednesday, and I live-blogged the entire thing below, as it unfolded. The best way to view it is to view the live stream here:
https://www.youtube.com/watch?v=8YO-_uhhH6Y
and to constantly refresh this page in a separate tab/window and follow along with my commentary. Enjoy!
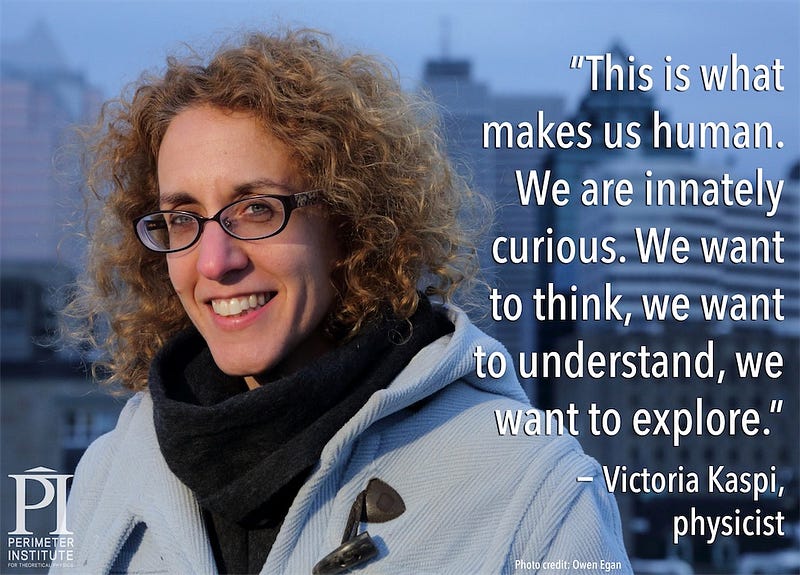
3:46 PM (all times Pacific in the updates to follow; the public lecture starts at 4:00): We are beginning the live-blog in the lead-up to the talk! To begin, I’ll take questions that have come to me through various social media outlets, sharing the answers to the best of our knowledge. If you want your question during the talk answered, either by me or by the speaker, tweet it out using the hashtag #piLIVE for a chance!
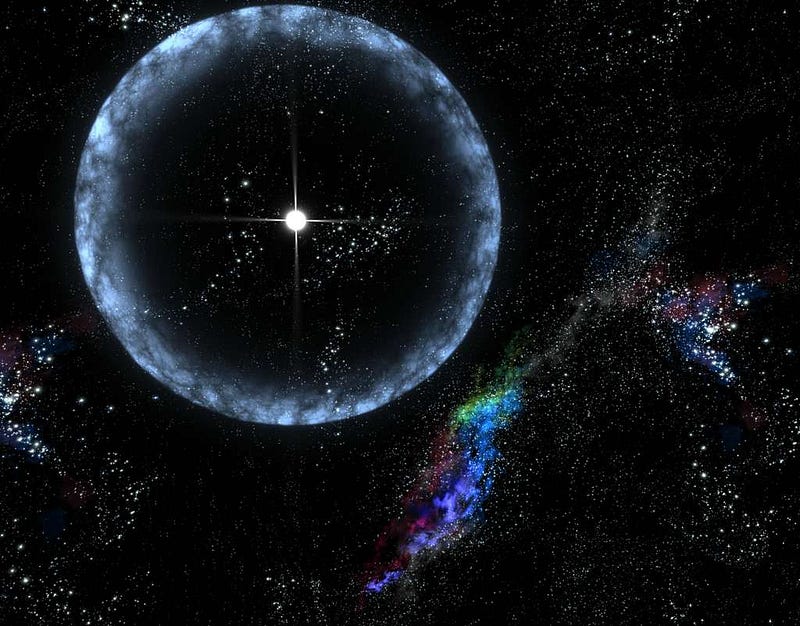
3:50 PM: Why don’t neutron stars decay? A free neutron has a lifetime of only about 15 minutes, yet neutron stars, made almost entirely of neutrons, don’t decay on timescales of at least hundreds of millions of years! The answer is that just like the neutrons in your atomic nuclei don’t decay, thanks to the binding energy of protons and neutrons through the nuclear force, the gravitational force of neutron stars is so great that even the neutrons at the surface don’t have enough energy to decay. You can do the math and ask how little the mass of a neutron star must be for it to decay, and it would have to be under the mass of Saturn. Since the lowest-mass neutron star is still over half the mass of the Sun (and thousands of times Saturn’s mass), we’re safe from neutron star decay.
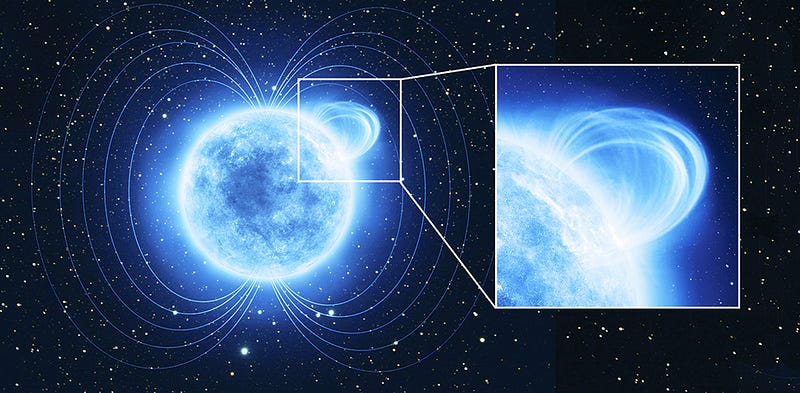
3:55 PM: Why do neutron stars — made of neutral things like neutrons — have such strong magnetic fields? There are three schools of thought:
- The stars they originate from have magnetic fields, and when they collapse down to neutron stars, that flux gets pinned and remains, becoming more intense due to the collapsing and winding up.
- The neutrons themselves have intrinsic magnetic moments (since they’re made of charged quarks), and so their motions account for the magnetic fields.
- The neutrons in neutron stars make up “only” about 90% of the neutron star, with about 9% made up of protons and electrons. (With a bit of “other” in there.) The protons and electrons are free to move, and they create currents and hence, magnetic fields.
The third explanation is most likely correct, but we don’t know for sure!
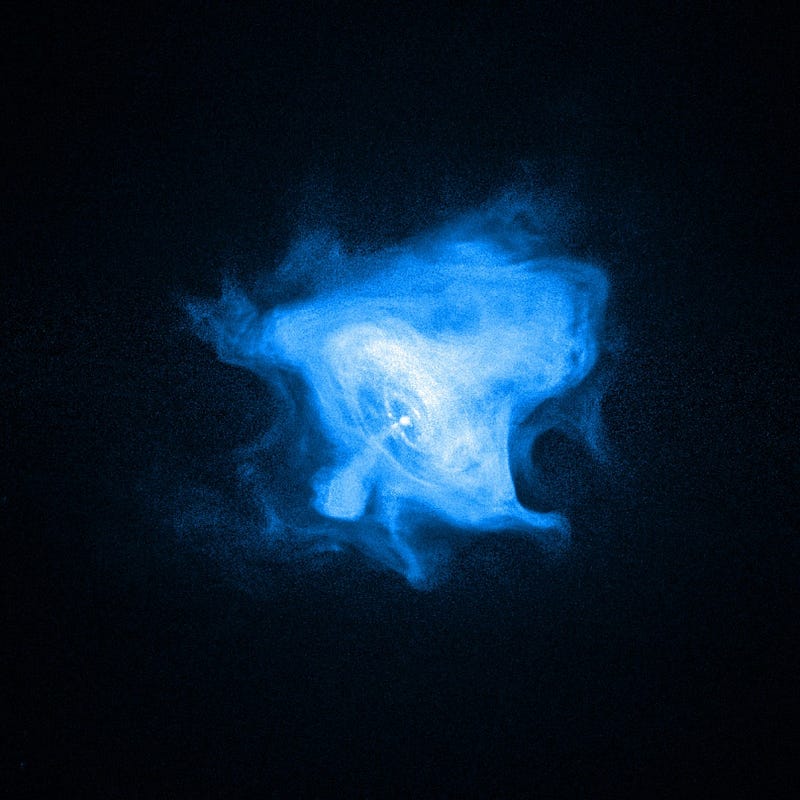
4:01 PM: Why are neutron stars so spherical if supernovae are asymmetric? Because of gravity: you get that much mass in that small a space, and the incredible force of gravitation will “sphericalize” you very, very rapidly. In fact, the need for a neutron star to be spherical is why an initially rapidly rotating neutron star will slow down to “only” about 1/3–1/4 the speed of light through gravitational radiation: a non-spherical shape will gravitationally radiate until it’s spinning slower, and hence becomes more spherical.
4:03 PM: The talk begins! What an on-time start! Way to go, Perimeter Institute!
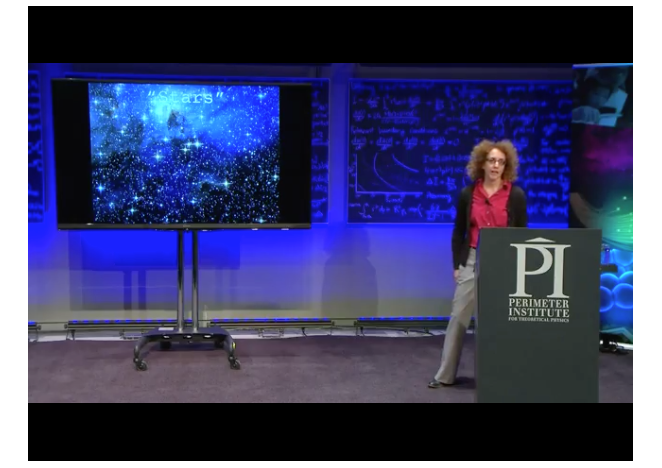
4:04 PM: “Stars” are not Lindsay LNN -0.16% Lohan or Justin Bieber (and not because it isn’t 2009, either), but they’re also not these things, above, that you think of. At least, not for Kaspi! She’s going to be talking about neutron stars, which are very, very different from what we traditionally think of as “stars” overall. They don’t fuse anything, for starters!
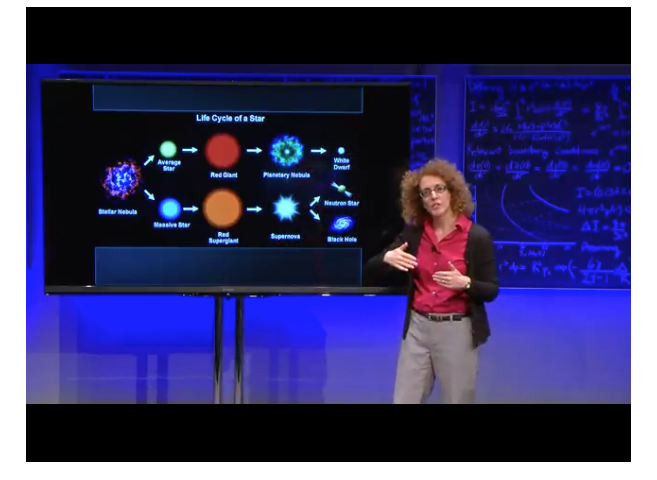
4:07 PM: This is how you get to be a neutron star: a massive star that goes supernova (a type II supernova, from a core collapse) but that isn’t massive enough to become a black hole. Between 8 and 20, 30 or 40 solar masses makes a neutron star, by the way, where the upper limit is uncertain.
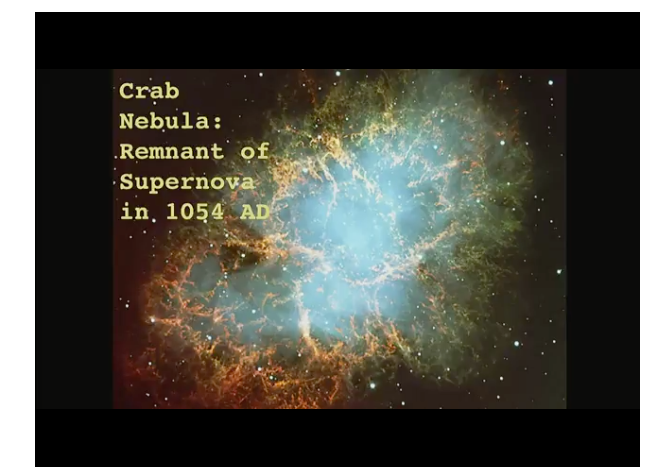
4:10 PM: One of the most fun “neutron stars” is at the center of the Crab Nebula. In 1054, many cultures observed a supernova, as bright as a crescent moon. (Kaspi says the full Moon, but that’s not quite right.) But we haven’t had a supernova in our galaxy in over 100 years. The last one we saw with the naked eye was in 1604 — Kepler’s supernova — but we’ve had at least two since: the one in Cassiopeia in the late 1600s and one in the late 1800s towards the galactic center, that we only discovered a few decades ago!
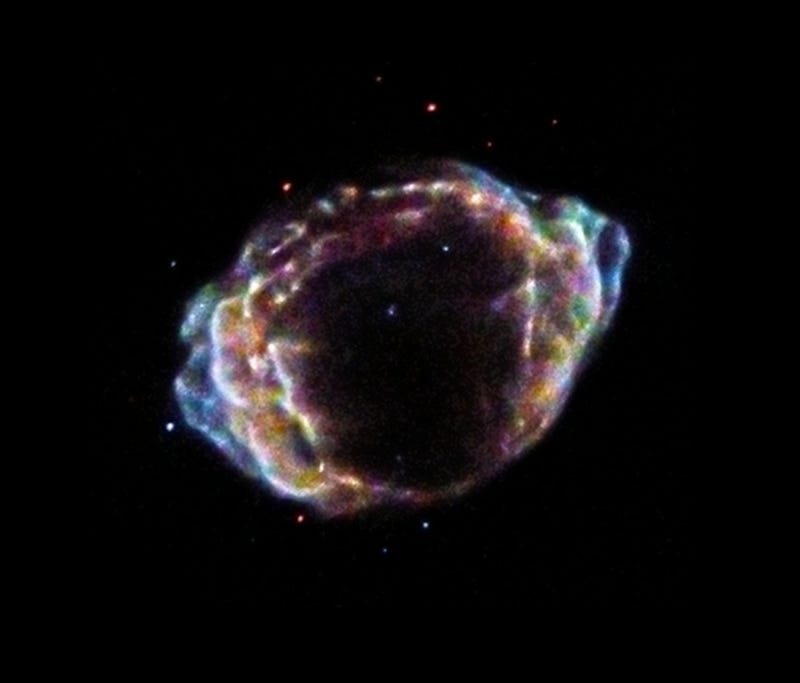
4:12 PM: For those of you who are skeptical, here’s the photo of the supernova from Chandra (above): G1.9+0.3. But this was a type Ia supernova that went off around 1868; you need a type II to make a neutron star!
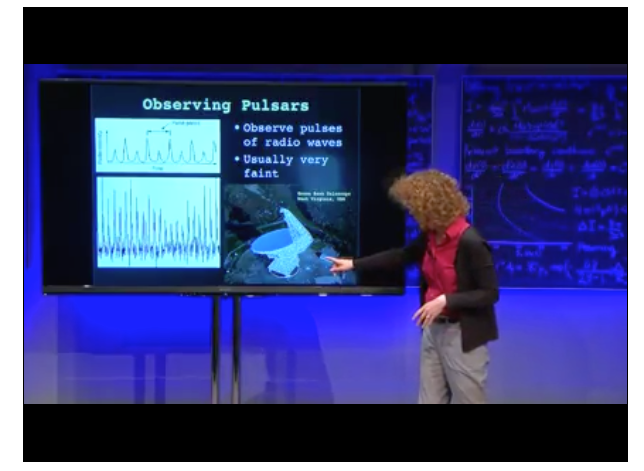
4:15 PM: If you want to find a neutron star, by the way, you don’t look in visible light; they’re too faint compared to other stars. Instead, you normally look in the radio, using a telescope (the Green Bank Telescope, at 100 meters across, is the largest steerable radio telescope in the world), and listen to the “pulses” that get emitted from the neutron star’s pole.
4:18 PM: Here’s what happens: any neutron star that has matter around it — such as from a binary companion — will have that surrounding matter ionized and then accelerated by its magnetic field. It gets emitted “down the poles” of the neutron star, and as the neutron star rotates, whenever that pole “points” at you, you get a radio pulse. That’s why we hear the “ticking” of a pulsar at the regular intervals that we do.
#pilive Is this what would happen to light if Einstein turned on a flashlight on a neutron star? pic.twitter.com/cUf1b1eYzR
— keith (@laughchem) February 4, 2016
4:20 PM: Here’s a fun question from Twitter (tweet your questions using #piLIVE): is this what light would do on the surface of a neutron star? It depends; light can escape from a neutron star’s surface, so it will bend “down”, but not so fast! If you shoot it parallel to the neutron star’s surface, it will clear the neutron star, and although it will be bent severely, it won’t collide with the star’s surface.
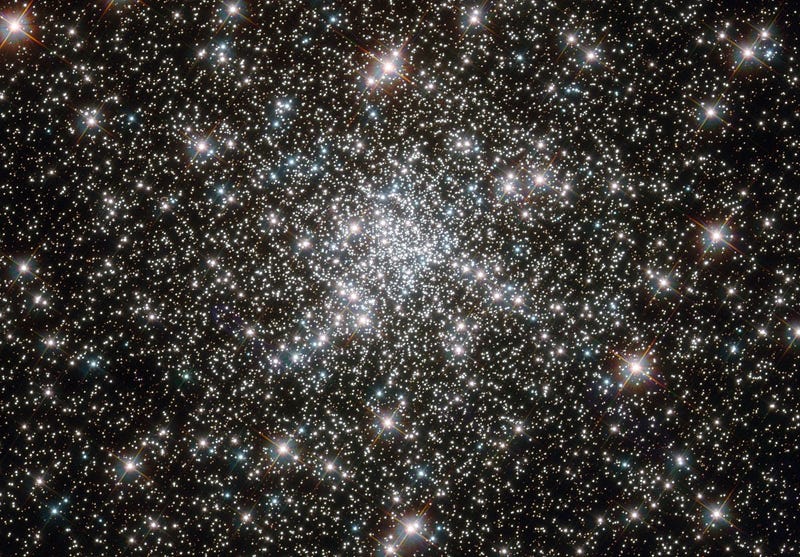
4:23 PM: Do proton stars exist? Yes they do; they’re called “stars.” They’re made out of protons… and also electrons. In fact, by number of atoms, even the Sun, which has been burning for about 4.5 billion years so far, is still about 87% protons by number of nuclei.
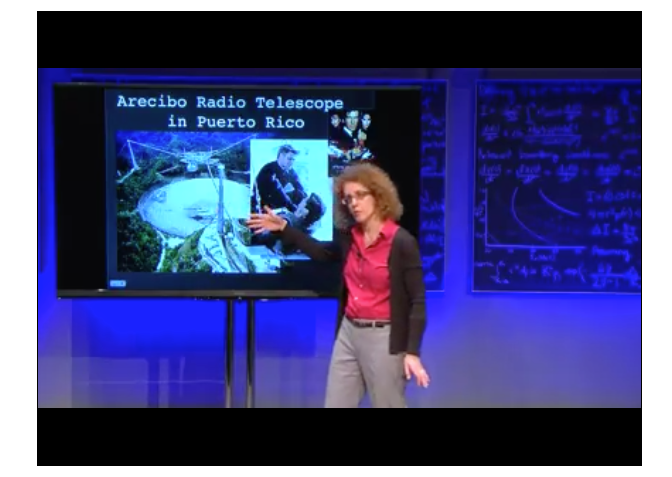
4:26 PM: The biggest non-steerable radio telescope on Earth is the giant one at Arecibo, in Puerto Rico. It’s over 500 meters (nearly a third of a mile) across!
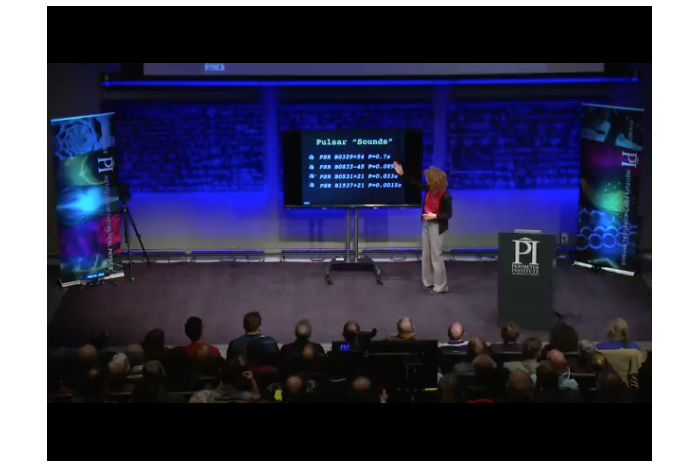
4:28 PM: Mark it down! You can listen to pulsars here, and hear how things go from “ticking” to actual sounds, just like real amplifiers/mechanical vibrators/speakers sound like! (I’m sorry, Nicole Gugliucci, I know this makes you so angry!)
4:31 PM: And if you want to hear the music of Terzan 5, the globular cluster, she’s playing it now. Lucky you that you’re only hearing the pulsars inside one or two at a time, instead of the cacophonous wreck that you’d hear if it were all of them at once! It’d be so inharmonious, it’d make Beck sound like Bach.
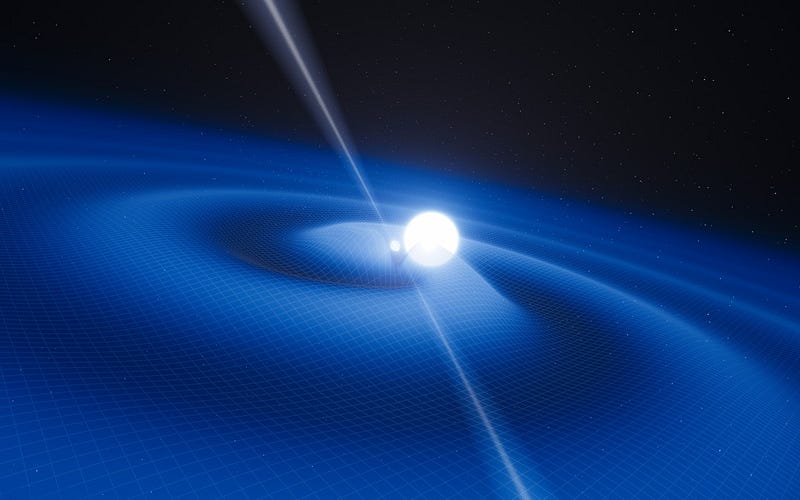
4:37 PM: It’s time, now, to talk about our first extreme system: a binary pulsar. What happens here is truly amazing. Unlike in Newton’s theory, where you orbit something, you return to your initial position eventually, in General Relativity, your orbit decays! For the Earth around the Sun, you’d like to live so long (it takes some 10¹⁵⁰ years), but for these neutron stars, the orbit changes on the timespan of months! Russell Hulse and Joe Taylor saw a binary pulsar — a pulsar orbiting another collapsed object — and found its orbit decayed consistent with Einstein, and won the Nobel Prize in the early 1990s (correction, 1994) for it.
4:41 PM: Another fun thing: because energy must be conserved, and a partially-decayed orbit is in a lower-energy state from the original one, there must be gravitational radiation coming from it. The hope of current and future gravitational wave observatories — LIGO and LISA — hope to find these!
4:44 PM: A lucky double pulsar: we are oriented less than 1 degree inclined to the orbital plane of a binary pulsar that we discovered; how serendipitous!

4:45 PM: Einstein predicts that these pulsars magnetospheres should eclipse the other, and that the pulses of one should be hidden by the other for about 30 seconds every few hours. In addition, the orbits and the magnetosphere eclipses should precess by a specific rate, also predicted by General Relativity. Did you bet on Einstein? You should have!
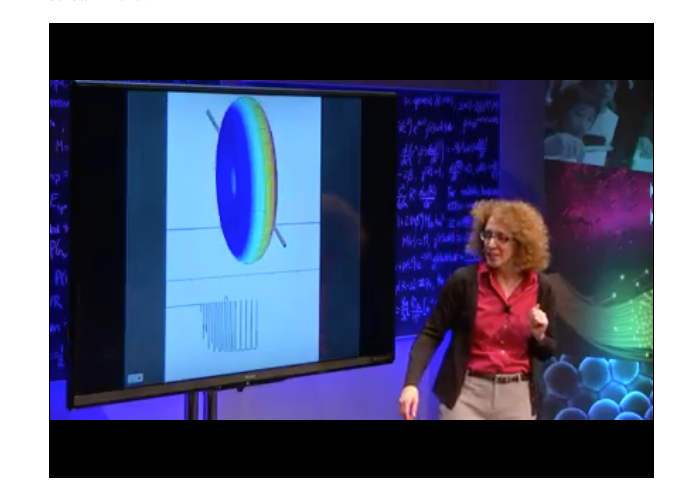
4:48 PM: And one of the fun things that was a bit unexpected: during the eclipse, a little bit of flux from the background pulsar sneaks through! This was a surprise, so Kaspi and her collaborators modeled the magnetosphere and saw what came through. Did you bet on Einstein again? This is a good theory, is my point: it’s celebrating its 101st anniversary this year, still being tested in new ways, and still coming up correct!
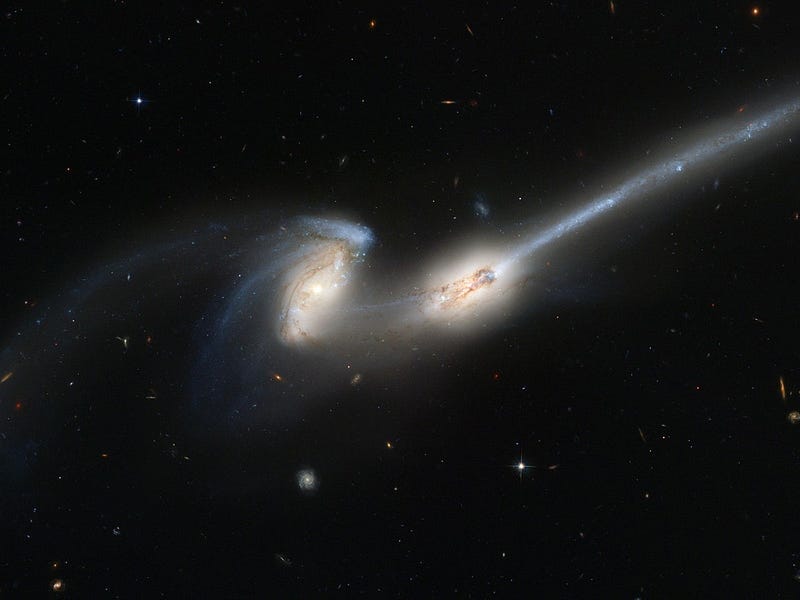
4:54 PM: The “Mice” galaxies (because they have tails) have merging black holes, and as the gravitational ripples pass through pulsars, we’ll be able to create a pulsar timing array and see how space is bent by these waves, and hence how the pulsar timing changes as the waves pass through them!
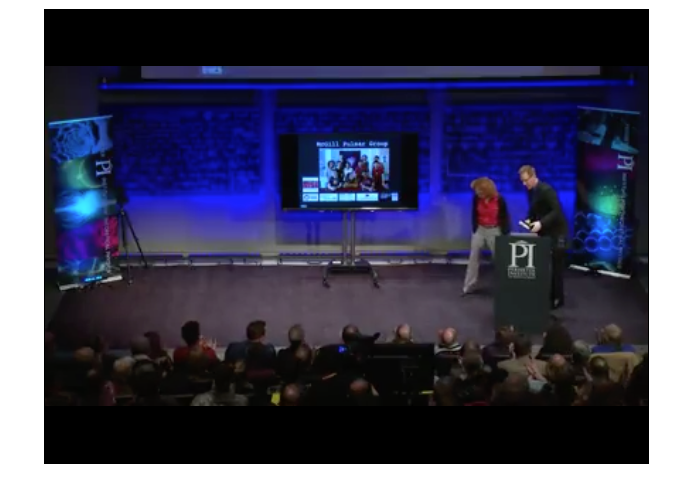
4:56 PM: A first! This is the very first Perimeter public lecture I’ve ever seenthat didn’t just end on time, but actually ended early! It was a good talk, and now it’s time for questions. Way to go, Vicky; this was great!
4:58 PM: There was a question about observing muons from pulsars or behind pulsars, and there’s a reason the answer is no that she missed: muons have a mean lifetime of 2.2 microseconds, but the closest pulsar to us is hundreds if not thousands of light years away! Even at ultra-high energies — even at some 10²⁰ eV of energy (at the GZK-cutoff) — each muon would still have a 99.99999%+ chance of decaying before it reached you. Stick with stable particles!
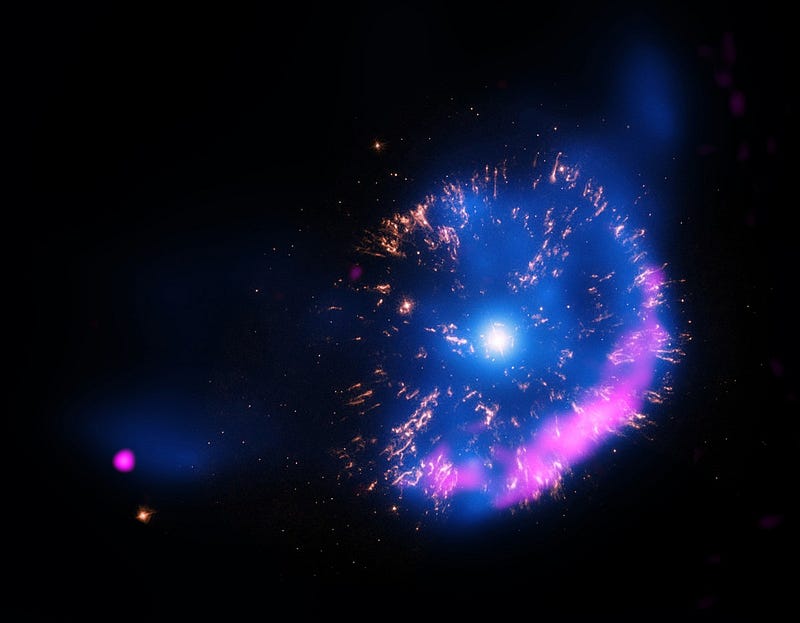
5:02 PM: Neutron stars don’t need to be in binary systems, but they need to have something to accrete. We know of ~2,500 pulsars, but only about 4% are in binary systems. You have to be lucky, because supernovae are catastrophic, and so only a small percentage of binary systems survive. The binary systems are the ones you hear about because we can learn so much more from them!
5:05 PM: Why aren’t all neutron stars pulsars? It’s tough! Because if you find a neutron star that doesn’t pulse, it might not be pulsing at you! In the double pulsar, one of them, in fact — the one they call ‘Pulsar B’ — isn’t pointing at us anymore. In other words, as long as there’s material to accelerate, you’ll get a pulsar. So they’re probably all pulsing, but perhaps just not for you. You’ve got to be lucky with pulsars!
5:08 PM: We’ve reached the end, but this was great! Thanks for joining me on the live blog, and I hope you enjoyed it and found the talk educational, and the live blog a great complement to it!
Leave your comments on our forum, and check out our first book: Beyond The Galaxy, available now, as well as our reward-rich Patreon campaign!