Nuclear fusion explained
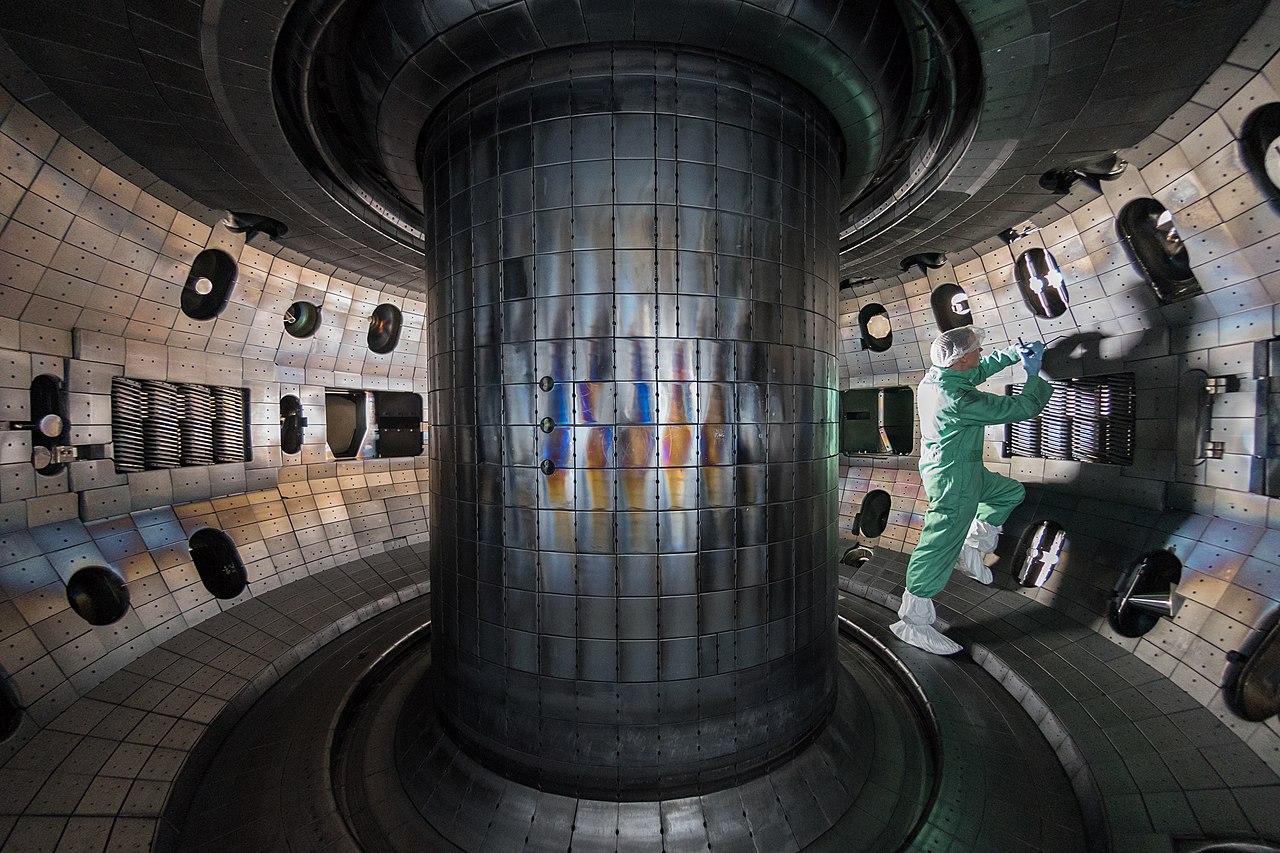
- Nuclear fusion is the most promising source of energy in the entire Universe, from massive stars all throughout space down to tiny reactors here on Earth.
- There are currently three major approaches being studied, with all three edging ever closer to the holy grail of energy generation: the breakeven point.
- However, enormous challenges still remain, and basic research remains grossly underfunded. Despite numerous companies making outlandish promises, we’re unlikely to see commercial fusion anytime soon.
When it comes to generating power and energy, physics gives us plenty of options. With simple mechanics, the energy of an object’s motion is put to work: using weights under the influence of gravity, flowing water, or moving air to turn a wheel or turbine. That motion is then used to generate electrical energy or other forms of power. There are chemical reactions as well, reliant on electron transitions in how atoms and molecules are bound together: where some sort of fuel is metabolized or combusted to generate energy, and that energy is then harnessed and similarly put to work. And finally, there are nuclear reactions, where the bonds between neutrons and protons inside an atomic nucleus are either broken apart or forged together to release energy, and then that energy is put to work.
While mechanical work freely takes advantage of already-existing environmental resources, like hydroelectric and wind power, it also has reliability and scalability issues, as well as its own environmental impacts. Chemical reactions are leveraged by all forms of life, including photosynthesis in plants and metabolic pathways in animals, but as a fuel source, combustion reactions are a finite, limited resource with significant consequences for pollution.
However, nuclear power is unique. It is literally hundreds of thousands to millions of times more efficient, in terms of the fraction of mass converted into energy, than all chemical reactions. Here’s what nuclear fusion is, and why it’s the future — but not the present — of power generation here on Earth.

Credit: Fastfission/Wikimedia Commons
It’s a strange idea to consider: that a tiny building block of matter, the atomic nucleus, holds the greatest potential for energy release. And yet, it’s true; while electron transitions in atoms or molecules typically release energy on the order of ~1 electron-Volt, nuclear transitions between different configurations release energies a million times as great, on the order of ~1 Mega-electron-Volt. In general, there are two ways you can release energy through a nuclear reaction:
- by splitting heavy nuclei apart in fission reactions,
- or by fusing light nuclei together in fusion reactions.
Both types of nuclear reactions, fission and fusion, can each release energy, as the most stable elements are located around the elements 26 through 28 (iron-cobalt-nickel) in the periodic table. Lighter elements release energy through fusion; heavier elements release it through fission.
Although nuclear fission and fusion are both associated with atomic bombs, as they can both undergo runaway reactions that continue to release energy so long as reactions can continue, they both have other applications as well.
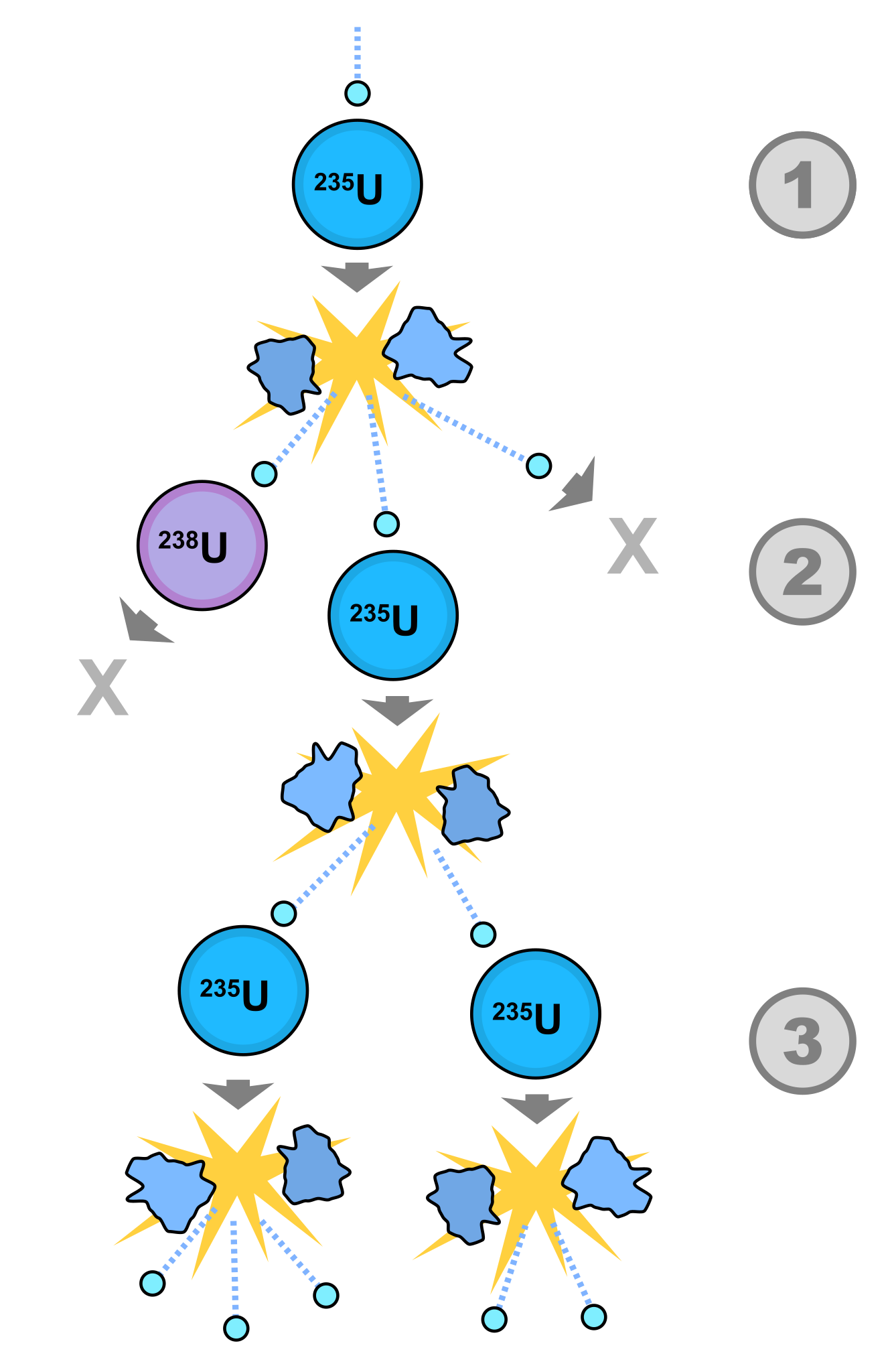
(Credit: Fastfission/Wikimedia Commons)
Nuclear fission typically relies on the absorption of a particle, like a neutron, by an unstable nucleus. When the right nucleus absorbs a neutron, like Uranium-235, for instance, it will split apart, releasing even more neutrons, allowing a chain reaction to take place. If the reaction rate is uncontrolled, you’ll get a bomb; if the reaction rate can be controlled, however, by absorbing neutrons and setting up conditions that restrict their speed, it can be used for controlled power generation. This is the basis for how all current nuclear (fission) reactors work.
Fusion, on the other hand, offers the potential of liberating even more energy than fission does. It occurs in all stars with core temperatures in excess of ~4 million K, and is the primary reaction powering our Sun. When you create a fusion bomb, its energy yield is far in excess of any fission bomb; the former is usually measured in megatons, while the latter is measured only in kilotons.
In principle, if we can control a nuclear fusion reaction with the same efficiency that we can presently control fission reactions, extracting energy at whatever rates we choose, it will stand to replace all other forms of energy generation as the dominant source for power on planet Earth.
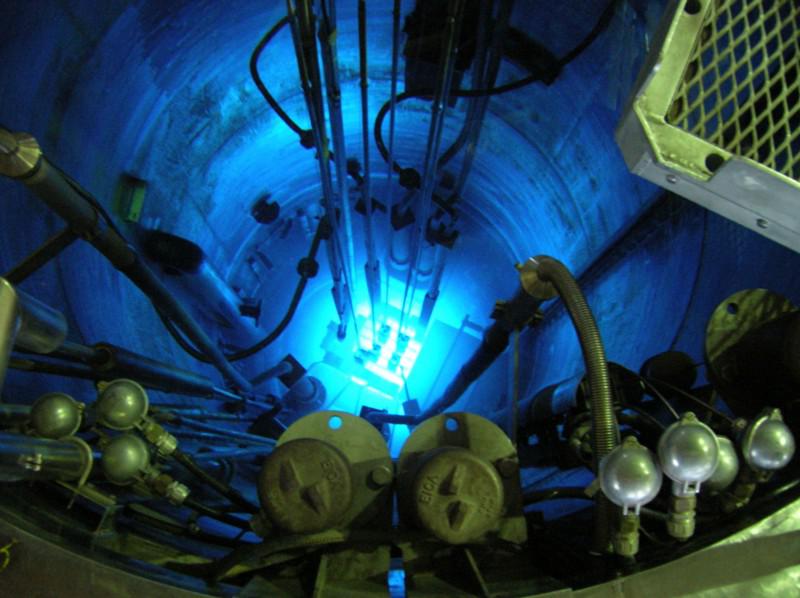
(Credit: Centro Atomico Bariloche/Pieck Dario)
There are presently three main concerns when it comes to the sources of energy and power that we consider.
- Availability. We want this energy to be there on-demand; when we need more of it, we want it accessible; when we need less of it, we don’t want to be wasteful. If we control the availability, like we do with fossil fuels or hydroelectric dams with reliably steady water flow, we have more flexibility than if we rely entirely on whimsical resources like solar and wind.
- Ubiquity. For our purposes on Earth, wind and solar power are ubiquitous; the winds and the Sun will always exist. Fossil fuels, however, will not; there’s a finite amount of coal, oil, and natural gas present in the Earth. Nuclear fission is also a finite resource, albeit a more abundant one than fossil fuels. Still, the mining and processing needs of uranium and other fissile elements means that the more we use, the harder new, pristine sources will be to come by.
- Sustainability. When we burn fossil fuels, we release planet-affecting pollutants into the atmosphere. When we produce nuclear fission reactions, we produce radioactive by-products, some of which have short half-lives and others which will persist for many generations of humans into the future. It’s no laughing matter that our energy consumption, as a species, has altered the climate substantially on Earth since the dawn of the industrial revolution; a problem which worsens with each passing year.
These three reasons emphasize why nuclear fusion power is the dream of sustainable energy. If we can control the rate of a fusion reaction, we can leverage it to produce energy on demand, with virtually no waste. Its fuel, hydrogen and its isotopes, are incredibly abundant here on Earth. There is no “running out” of nuclear fusion fuel, not for billions of years. And while nuclear fusion may produce slight amounts of radioactive products like tritium, there’s never a risk of a reactor meltdown or of long-term environmental damage. Compared to even solar power, which requires the mining of rare elements and the use of chemicals and scarce resources to create solar panels, nuclear fusion is the most sustainable energy choice.
Of course, all of this relies on one assumption that we, as a species, have not yet achieved: that we can reach the breakeven point when it comes to nuclear fusion power. The holy grail of energy is to have a self-sustaining nuclear fusion reaction that produces more usable energy out of its reactions than required to be inputted into the system/device to initiate the fusion reactions in the first place.
The reason this is such a hard problem is twofold. First off, it isn’t a trivial matter to create a nuclear fusion reaction. So long as you restrict yourself to working with materials like hydrogen, deuterium, helium-3, and other stable light elements and isotopes, it requires tremendous temperatures and energies to get a nuclear fusion reaction to occur at all. Controlling and sustaining these environments is no easy task, and it requires tremendous energies even at the outset to create the conditions necessary for fusion.
And secondly, you can’t simply approach this with the goal of creating more energy through fusion than you put into the system to get the reaction going: that’s what’s known as a bomb. Instead, what you need to do is produce energy at a slow enough rate that you can use it to produce useful quantities of power: energy over time.
Reaching the vaunted breakeven point requires both producing more energy from your reactions than you put into the system to initiate those reactions and also extracting that energy and putting it to use. So far, both problems remain unsolved in tandem, but there are three main approaches researchers are taking as they attempt to revolutionize humanity’s relationship with energy.
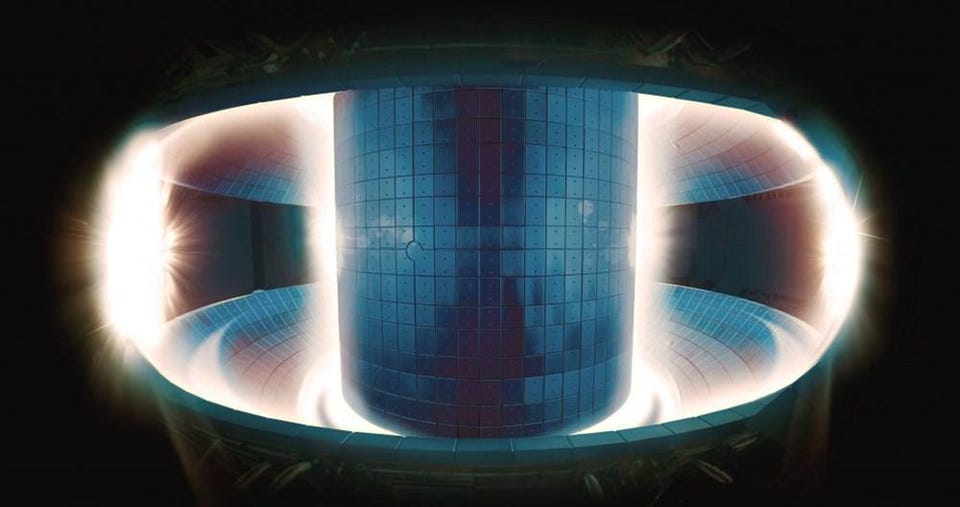
Credit: National Fusion Research Institute, Korea
Approach #1: Magnetic confinement fusion. Nuclear fusion fuel, remember, isn’t merely atoms, but the atomic nuclei at the cores of atoms. One approach to nuclear fusion is to fully ionize atoms, stripping their electrons away, until only the atomic nuclei remain. By creating this superheated plasma of atomic nuclei that can fuse together, the idea is then to bring those nuclei together, overcoming the electrically repulsive force between them, to initiate fusion reactions.
The most successful approach here has been to confine this superheated plasma using powerful electromagnets, bringing the atomic nuclei together inside a cavity known as a Tokamak. Tokamaks have been researched for decades, and have been producing fusion reactions inside of them for the entire time.
The key difficulties with this approach lie in keeping the plasma confined (otherwise it collides with the walls of the device) and extracting the energy produced from the reactions to create usable power. Although this approach has long been viewed as the most promising route toward nuclear fusion, it’s received a pittance of funding relative to the proverbial “moonshot” levels that will be needed to have a legitimate chance of long-term success.
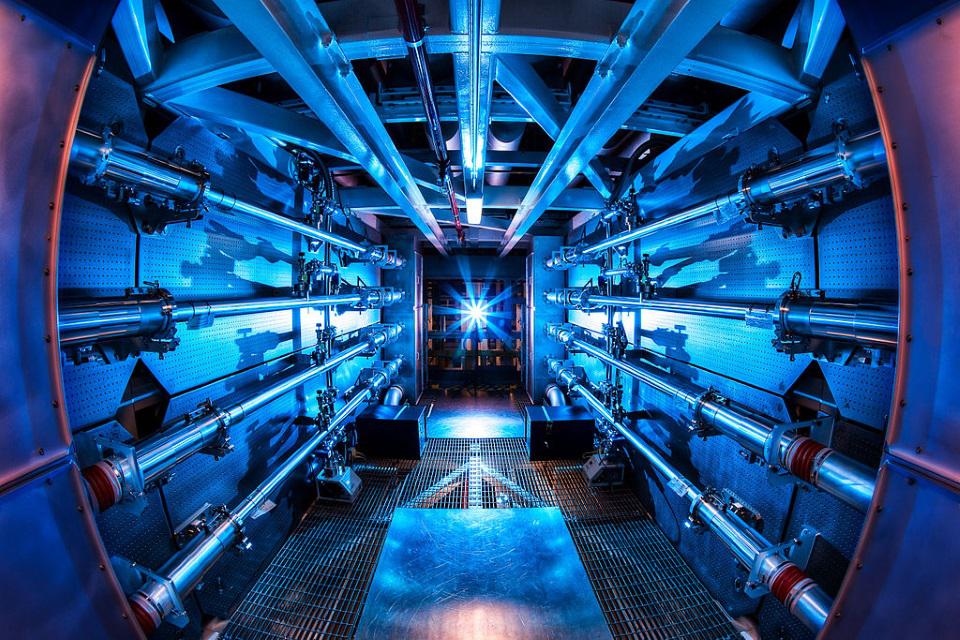
Credit: Damien Jemison/LLNL
Approach #2: Inertial confinement fusion. Instead of messing around with magnetic fields, why not just try the brute force approach? That’s what inertial confinement fusion attempts to do. By taking a pellet of material that can be fused, a series of high-powered lasers on all sides are fired at the target pellet, rapidly increasing its temperature and density until a nuclear fusion reaction can be triggered. Although it requires storing up a tremendous amount of energy for the “laser shot” that compresses the pellet, it’s possible that the fusion reaction generated will release even more energy, allowing us to someday surpass the breakeven point.
This approach, like that of magnetic confinement fusion, has also been around for decades, producing fusion reactions the entire time. Despite recent advances inching us closer to the ultimate breakeven goal, the same two problems remain. Even though we’re producing ever-greater amounts of energy through this method, it requires us to first store up an enormous amount of energy in a series of capacitor banks, and then release that energy all at once. We don’t have a self-sustaining reaction; just a single burst, and then we struggle to gather and harness that generated energy.
Even though we’re getting closer to the ultimate goal, once again, we are many orders of magnitude away from the breakeven point, and because funding is relatively scarce relative to the hundreds of billions of dollars we should be investing in this technology, progress remains slow.
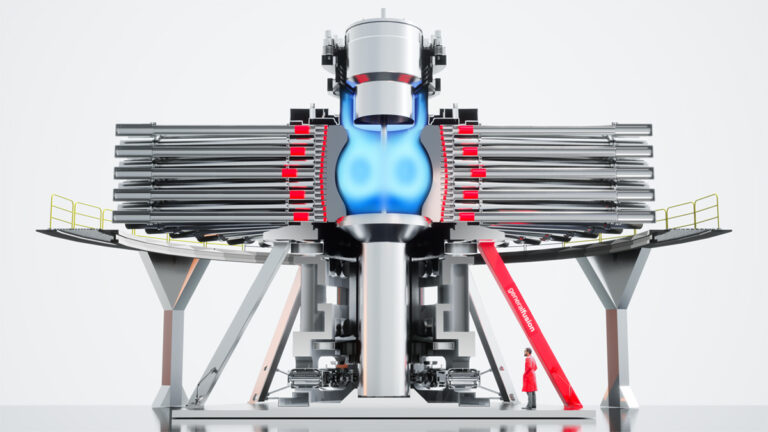
(Credit: General Fusion)
Approach #3: Third-way approaches. This is where a lot of private initiatives — some legitimate, some suspicious, and others that are unquestionable charlatans — are getting involved. There are two main alternative approaches to the mainstream methods, and both of them can actually create fusion reactions. As it turns out, it’s not all that difficult to get fusion going, but it is remarkably difficult to get as close to the breakeven point as either inertial confinement or magnetic confinement fusion does. You can either:
- Attempt magnetized target fusion, where a superheated plasma is created and confined magnetically, but then surrounding pistons compress the fuel inside at the critical moment. This creates a small burst of fusion, like a combination of the earlier two approaches, and is the most mainstream of the third-way alternatives.
- Or you can attempt subcritical fusion, where you attempt to trigger a fusion reaction with a subcritical (i.e., zero chance of a meltdown) fission reaction. This approach has many novel players in the space, but right now its the farthest away of all such methods from the breakeven point.
As with most endeavors on the fringes of mainstream science, there are legitimate researchers working on the technologies behind these dreams, but there’s also a lot of wishful thinking and a lot of promises that are highly unlikely to pan out. Some of the players in the game are taking approaches similar to Solyndra; they are doing some basic research while counting on an unlikely route to success. Others are more like Theranos, where the technologies they are counting on leveraging do not exist. Quite simply put, the nuclear fusion space really is a jungle out there.

Credit: Sarang/Wikimedia Commons
So far, unfortunately, nobody is particularly close to the breakeven point, and that is the one question you should always ask when it comes to the viability of nuclear fusion technology to supplant other sources of energy for humans to rely on.
- “Can you produce more energy than you have to put in to get the reaction going?”
- “How much of the produced energy can you harness to produce usable power?”
- “And how close are you, quantitatively, to reaching the breakeven point?”
These are the questions we need to be asking every time a facility or company makes a claim that nuclear fusion power will be available and ready to use in just a few years. As retired plasma physicist Daniel Jassby unforgettably put it in his 2019 essay, Voodoo Fusion Energy:
“The permanent fusion R&D organizations, mainly government-supported labs, are the silent spectators of the parade of naked emperors, only occasionally challenging their insupportable assertions and predictions. One feature that voodoo fusion schemes do share with their neutron-producing rivals is that while they will never put electricity onto the grid, all of them take plenty of energy from the grid. The voracious consumption of electricity is an inescapable feature of all terrestrial fusion schemes.”

(Credit: Sandpiper at English Wikipedia)
All of this underscores the true problem we’ve created for ourselves, as a society, when it comes to the energy sector. The current primary method we have for producing energy across the globe remains the 18th century technology of combusting fossil fuels, which is easily the largest cause of rising CO2 levels in our atmosphere, ocean acidification, and our rapidly and catastrophically changing climate. The best present technology we have for replacing that ancient and consequence-laden technology, nuclear fission, has been globally maligned by many owing to the fear of nuclear waste, fallout, war, and more. Despite the scientific case for nuclear fission, it still produces only a small fraction of our global energy.
And despite the dire need for basic research in the energy sector, and the severely underfunded promise of nuclear fusion, there hasn’t been even one year in the history of the United States where we’ve invested even $1 billion, even adjusted for inflation, toward the goal of nuclear fusion. Unless we make a sustained large-scale investment in the actual, legitimate technology that’s right at our fingertips, we’re going to doom ourselves to being taken in by two types of charlatans:
- the well-meaning ones who wind up fooling themselves that they can succeed where the laws of physics stand in their way,
- and the snakes-in-the-grass who know that they’re lying to you about the promise of their technology, but are happy to take your money anyway.
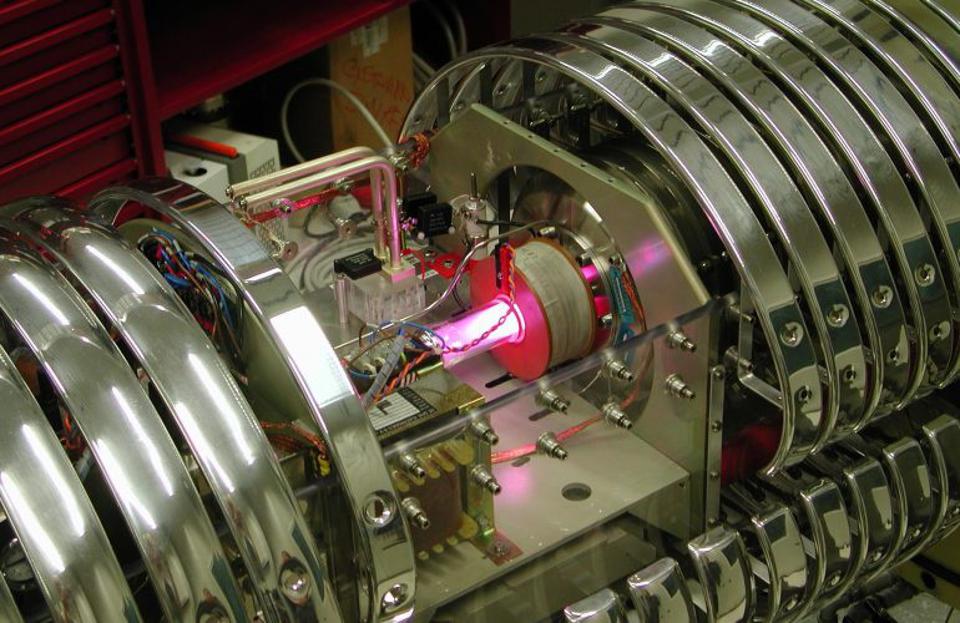
Credit: LUNA Experiment/Gran Sasso
When it comes to the Universe, there is no reaction more life-giving or life-sustaining than nuclear fusion. It is literally at the heart of not only every star, but of the countless brown dwarfs — i.e., failed stars — that undergo deuterium fusion during their lives. When light elements bind together, the new element they produce is lighter in mass than the initial reactants, and that fusion reaction then gives off energy proportional to the mass difference: via Einstein’s E = mc2. On metrics of energy availability, fuel source availability, and environmental impacts, nuclear fusion is far and away the best choice of every option available to generate power.
Unfortunately, a 60+ year underinvestment in this technology has set us egregiously further behind on this important scientific front, and now the vultures have gathered: full of big dreams and empty promises, with nothing to show for it other than token amounts of fusion that are many orders of magnitude away from even approaching the breakeven point. If there’s one promising technology deserving a moonshot-level investment, it’s nuclear fusion power. It is the most promising path toward mitigating the current climate and energy crisis. But it is not today’s technology, and it is unlikely to become tomorrow’s unless we dramatically revolutionize how we fund and conduct basic research and development here on Earth.