No, The COVID-19 Coronavirus Is Not Actually Red
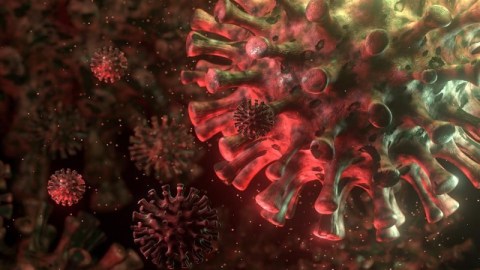
In reality, it has no color at all. Here’s why.
Every time you see an illustration of the COVID-19 coronavirus, it’s shown with a red color to it. Sometimes it’s red throughout; sometimes it’s red on the tips; sometimes it’s shown with a color gradient that gradually transforms from red-to-white as you move from the central core to the spike-like tendrils at the end. A few other colors are sometimes used: yellow or purple, either in lieu of red or in addition to it.
Although most of these illustrations get the shape of the virus correct, the colors are all wrong. In reality, even if you used the most powerful magnifying glass or microscope you could dream up, the COVID-19 coronavirus would not only not have a color at all, it would be entirely invisible to human eyes. Here’s the reason why.
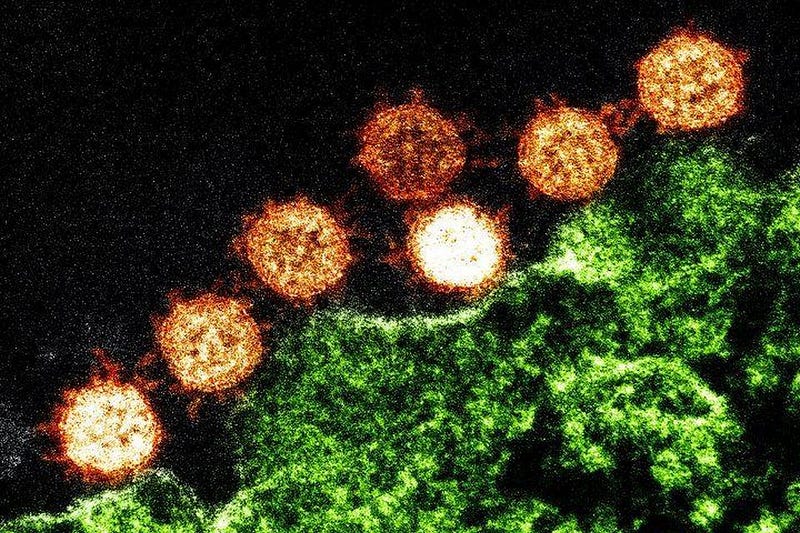
The only reason the objects in our world are visible to us is because of how they interact with light. Objects that absorb all forms of light perfectly are black (as long as they’re not hot enough to begin glowing due to their own radiation); objects that reflect all forms of light perfectly are white.
So why do certain objects have a color? It’s because they absorb certain colors of light but fail to absorb, and hence reflect, light of other colors. Plants are green because the chlorophyll molecules in their leaves absorb red and blue light efficiently, but fail to absorb green light. In the fall, the chlorophyll molecules are broken down, allowing the pigments of the other molecules in the leaves to show. Some leaves are dominated by carotenoids, which fail to absorb yellow and/or orange colors; others are dominated by anthocyanins, created only in the fall, which fail to absorb red or purple colors.
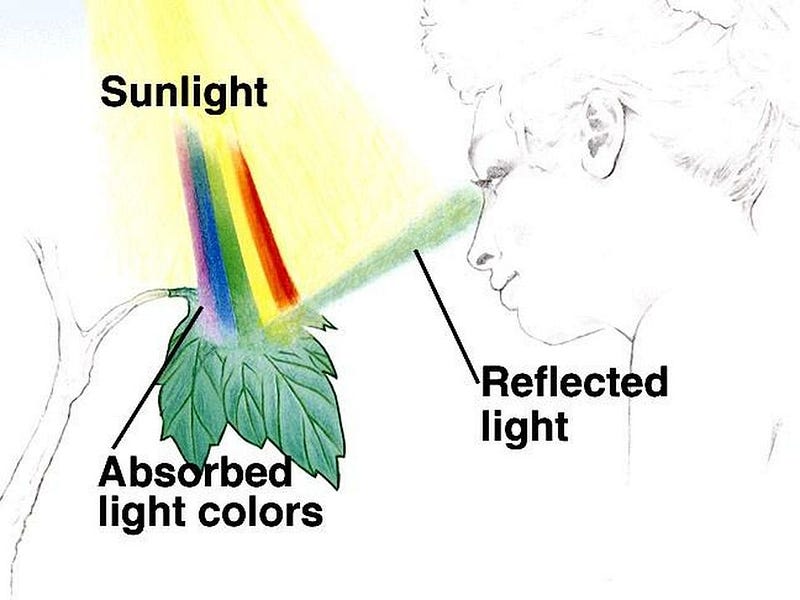
The reason that colors exist at all is because of the shapes, sizes, and other microscopic properties of the particular molecules that interact with this light, and then how that light gets interpreted by our eyes. Most of the natural light on our planet comes from the Sun, meaning that it’s “white light,” or light from all the different colors combined.
In reality, there’s even more light than we can see, as the Sun emits everything from short-wavelength X-rays to longer-wavelength ultraviolet, visible light (from violet to red, through the full spectrum), infrared, microwave and even radio waves. In the visible part of the spectrum, our eyes have rods, which measure only a monochrome response, as well as cones, which can measure sensitivity to three different ranges of colors. Our brains then interpret those signals as colors, and that’s what we see.
But materials can do something else besides absorb or reflect light: they can also transmit it. Some materials aren’t absorptive or reflective, but transparent: allowing light to freely pass through it. As light travels through a transparent material, the individual molecules inside are largely insensitive to the light passing through it. The molecules don’t absorb the light, nor do they reflect it away. Instead, the light simply passes through as though the individual molecules posed no obstacle to the light propagating at all.
What’s even more interesting is that the transparency of anything is wavelength-dependent. A glass window or lens can be transparent to visible light, but can have a thin coating on it that either absorbs or reflects ultraviolet light. Human beings absorb and reflect visible light, but are transparent to radio waves. And Earth’s atmosphere, which allows visible sunlight to pass through it, absorbs a wide variety of other wavelengths, which is why we need space telescopes to do infrared astronomy.
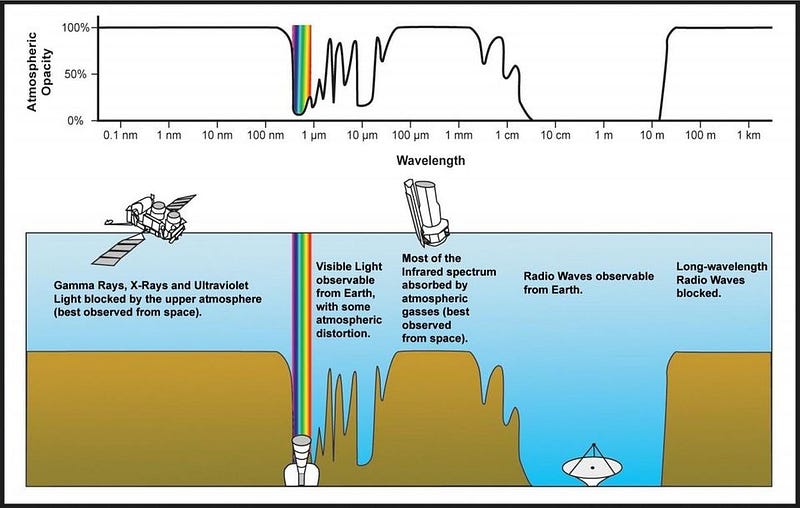
So what does all of this have to do with the COVID-19 coronavirus?
The answer is simple: light is defined by its wavelength, and the way that any type of matter interacts with light is dependent on the physical size of that matter. COVID-19 is a small virus, only about 50 nanometers (about 1/1000th the width of a typical human hair) across. For comparison, all of the light we can see, from the shortest-wavelength violets to the longest-wavelength deep reds, only span from 400 to 700 nanometers.
Think about what that means. You have a very small piece of matter — the COVID-19 coronavirus — and a comparatively much longer wavelength of light. Although your intuition might tell you that these light waves could still interact with a much smaller piece of matter, the fact of the matter is that they don’t. Perhaps the best demonstration of this, believe it or not, is your microwave oven.
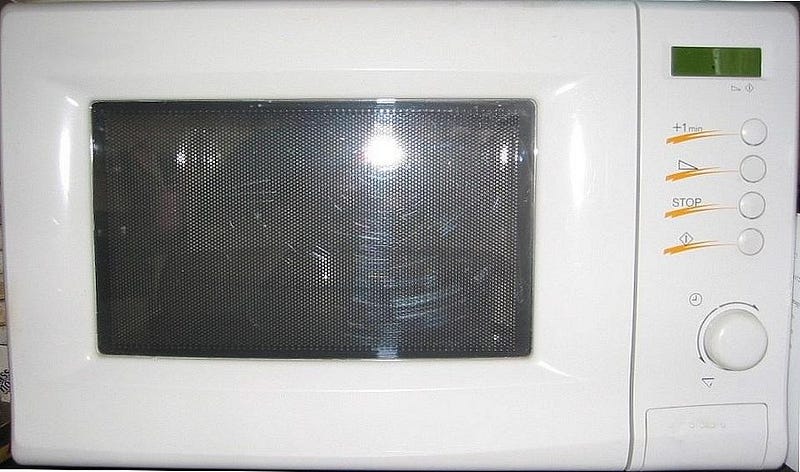
When you look at the door of your microwave oven, you’ll notice there’s a type of screen on the door with little holes: holes about 1 millimeter (about 0.04 inches) in diameter. A millimeter is about 2,000 times larger than the wavelength of visible light, so when you press “start” on the microwave and the light goes on, you can see what’s going on inside. The mesh door on your microwave is transparent to visible light.
But these holes are much, much smaller than the wavelength of the microwaves being generated to cook the food inside the oven. Typical microwave ovens cook food with a wavelength that’s about 12 centimeters (around 5 inches) long, which is far too large to fit through the holes. As a result, the microwaves get reflected and bounce around inside until they cook your food, all while the visible light freely passes through to your eyes.
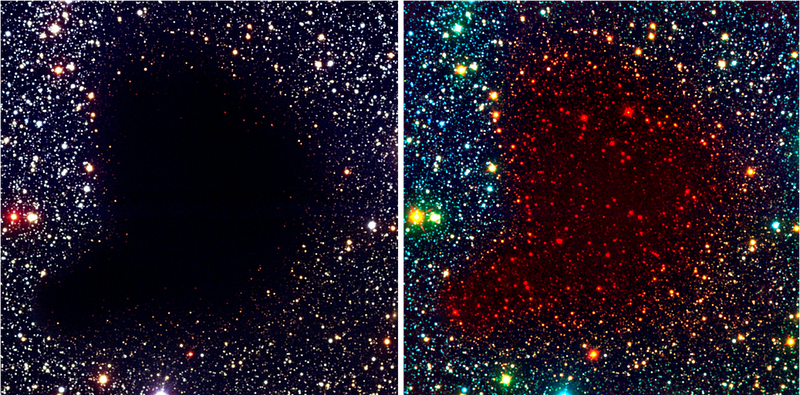
The idea that whether a material absorbs, reflects, or transmits light is extraordinarily familiar to scientists. Astronomers in particular leverage this all the time by looking at the Universe in different wavelengths of light. The Universe itself is full of dust grains that absorb visible light, which is the reason why the Milky Way has those dark features silhouetted against the billions of stars behind it.
But those dust grains are of a particular small size. They absorb blue (short-wavelength) light better than red (long-wavelength) light, and that means we can look at even longer (infrared) wavelengths to “see through” that dust. The reason? Longer-wavelength light is transparent to those dust grains, enabling us to see the Universe beyond that. And that brings us full circle back to the COVID-19 coronavirus.
At just 50 nanometers in size, visible light is far too long in wavelength to be sensitive to COVID-19; it just passes through it. So do all longer wavelengths of light: infrared, microwave and radio. But when you get into the ultraviolet part of the spectrum, which spans from 10 nanometers to 400 nanometers, the shorter end of the ultraviolet spectrum can definitely interact with the virus. If we want to image COVID-19 with light, ultraviolet radiation can do the job.
But the best job of all is accomplished by electron microscopes, which leverage the principle of wave-particle duality to use electrons of a specific energy to image particles this small. By doing so, we can construct a three-dimensional map of what COVID-19, or any small particle, actually looks like in terms of its shape. Electron microscopes give us the best resolution imaging of particles down to this tiny size, even for an individual virus.
It’s true that the COVID-19 coronavirus has a roughly spherical shape with tendrils sticking out of it: that’s the actual physical shape that gets revealed through a variety of imaging techniques. The individual spikes give it the crown-like appearance that gives the virus its name: “corona” comes from the Latin word for crown.
The name “coronavirus” was first used in 1968, after scientists using these revolutionary microscope techniques saw a visual similarity between the Sun’s corona (as revealed during solar eclipses) and the appearance of this class of viruses. But these techniques can only reveal the actual physical shape of the virus; they cannot reveal its color, and for good reason. In the wavelengths of light where “color” is meaningful to humans, the COVID-19 coronavirus is nothing but invisible.
In this Universe, everything is subject to the laws of physics. In physics, light is defined by its wavelength, and visible light occurs in a narrow range between 400 and 700 nanometers. Particles that are too small to interact with these wavelengths of light — particles like the 50 nanometer wide COVID-19 coronavirus — neither absorb nor reflect that light, but simply don’t interact with it at all. To the types of light we can see, COVID-19 is completely transparent.
COVID-19 still interacts with light, but only the shorter-wavelength light like ultraviolet and X-ray light. We can still probe its structure with techniques like electron microscopy, revealing its shape and structures. But COVID-19, like any matter particle of such a small size, has no actual color at all. You are free to visualize it however you like, but we must realize, in the fight against this disease, it truly is the invisible enemy.
Ethan Siegel is the author of Beyond the Galaxy and Treknology. You can pre-order his third book, currently in development: the Encyclopaedia Cosmologica.