Landing at the End of the Solar System
We’ve just landed our first-ever probe on the surface of a comet. Here’s what it means, and what we’ll learn.
“I must trust that the little bit of love that I sow now will bear many fruits, here in this world and the life to come.” –Henri Nouwen
When you think about the Solar System, you very likely think about the Sun, the inner, rocky planets in close orbit around it, the gas giant worlds a little bit farther out, the belt of asteroids that separates them, and the small, icy worlds out beyond the gas giants. If you think of these five separate classes of objects: the Sun, the rocky worlds, the intermediate asteroids, the outer gas giants, and the icy worlds beyond them, you’ve really hit on all the major components of what all solar systems are made up of.
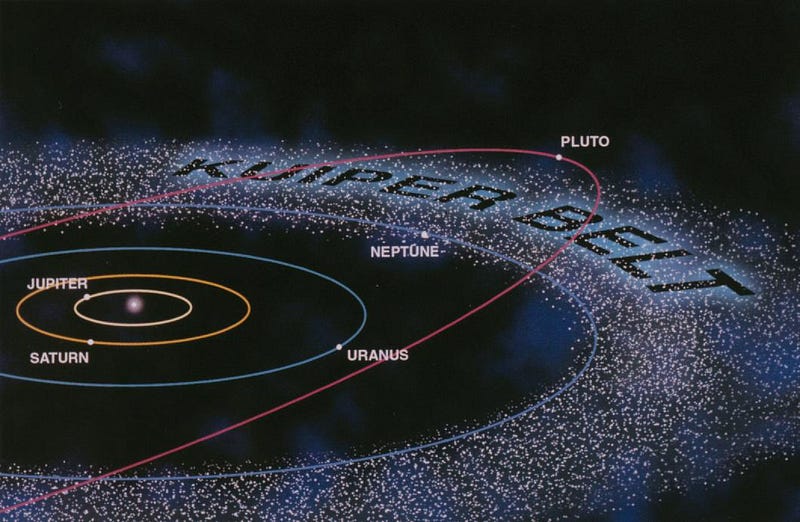
There’s an awful lot that we know about each of these types of objects, both from remote observing via telescopes and orbiting space probes, but also from actual visits to these distant worlds, small and large. We’ve sent landers to the Moon, Venus and Mars, to Saturn’s giant moon Titan, and recently to the tiny rubble-pile asteroid, 25143 Itokawa.
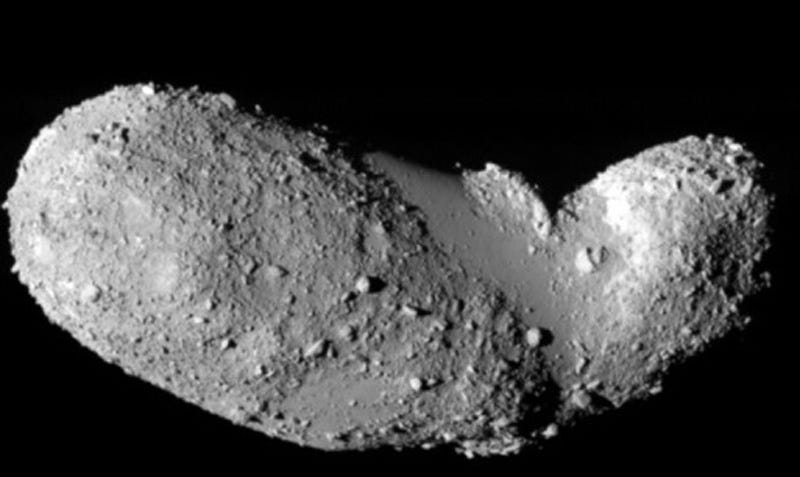
Landing on a distant object like this is a tremendously difficult task, because these objects we’re landing on are so far away that we — as humans — don’t have the ability to adjust on-the-fly to conditions, since we’re limited by the speed of light. This isn’t so bad for an object with a large gravitational pull, for a number of reasons:
- We have a lot of experience both entering and leaving the window of a strong gravitational body.
- So long as you know the mass of the object and your distance above its center, it’s very easy to calculate the ideal trajectory.
- You have a landing window: if you miss your target by a few meters or a few kilometers, you’re still safely going to land on it.
- And, corrections to the orbit can be done with very small maneuvers well in advance.
But most importantly, the object is trying to pull you towards it, and that helps an awful lot.
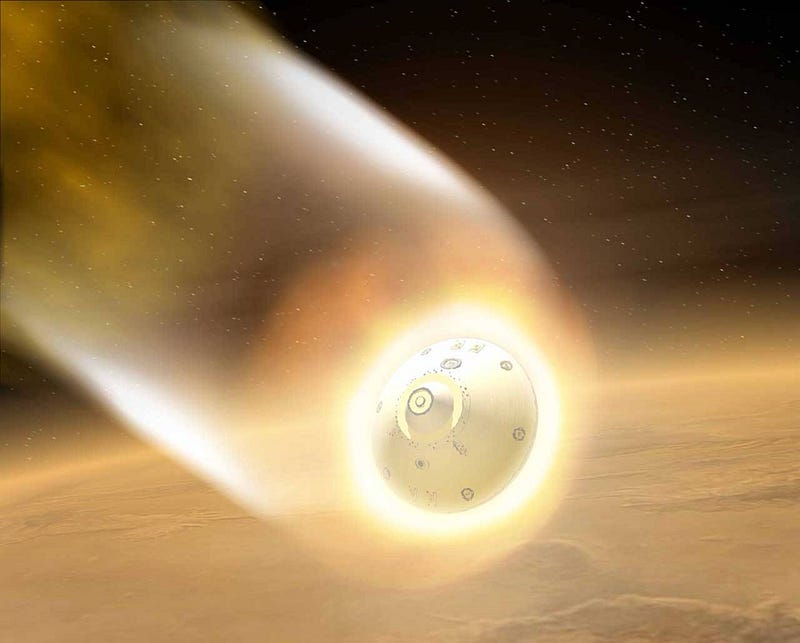
Now imagine, instead of trying to land on an object that’s attracting you, you’re trying to land on, basically, a speck of dust. Not that a comet or asteroid is as small as a speck of dust, but it has virtually no gravitational attraction at all. In the case of asteroid Itokawa, for example, it isn’t even a solid body, but rather a pile of rubble, as gravitation can’t even pull it into a single giant rock!
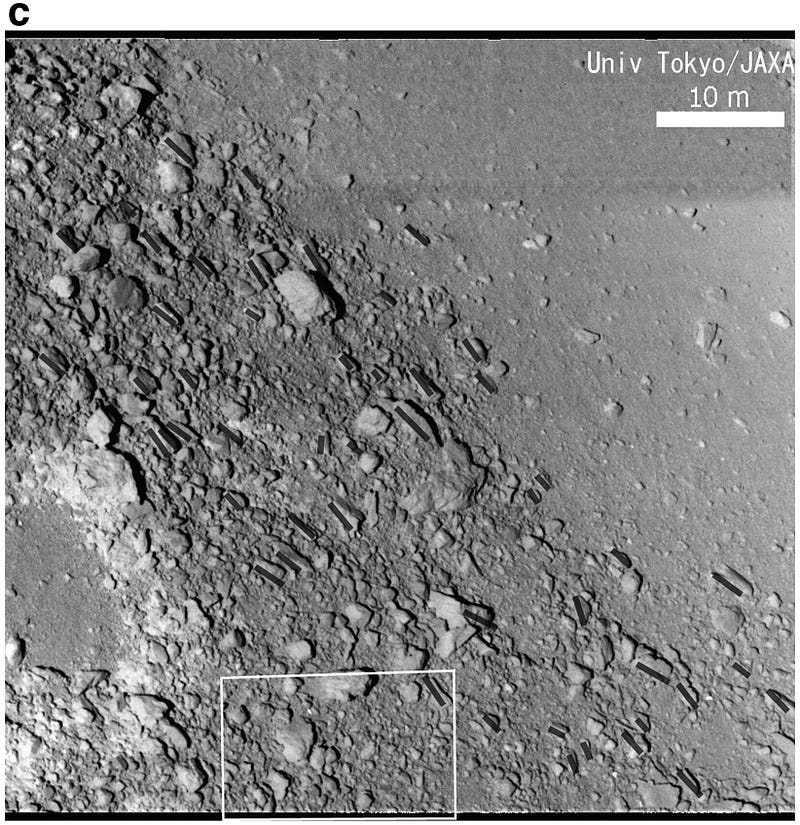
Now imagine going after a comet, an icy body that’s made, on average, of lighter elements than anything else in our Solar System. With the exception of the gas giants — which managed to hang on to large amounts of leftover hydrogen and helium gas from the Solar System’s formation — these objects are made up of the fewest heavy elements, and rather than being mostly rocky materials, are made up of icy materials! Not just water ice (H2O), mind you, but also dry ice (solid CO2), methane ice (solid CH4), and potentially even ammonia ice (solid NH3).
When we check out a comet, that’s what we’re anticipating finding.
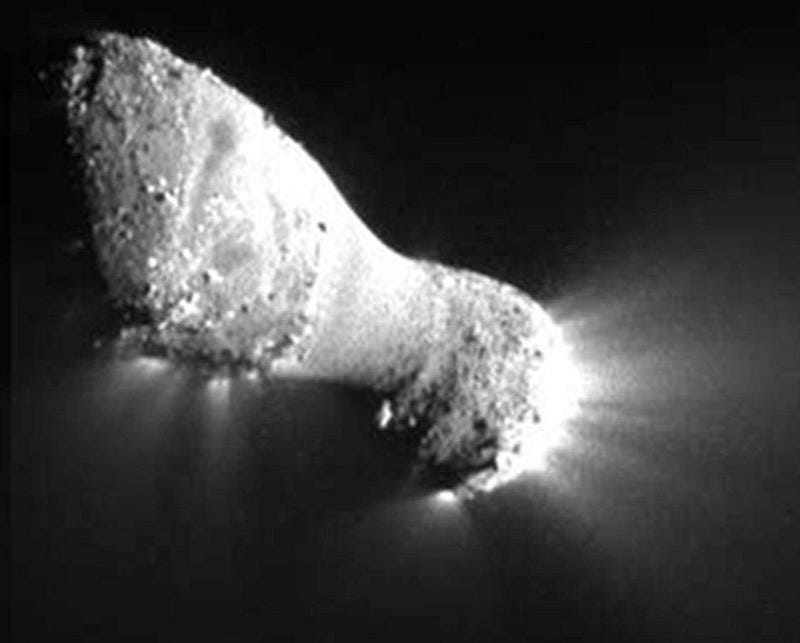
The problem is, comets start out really far away from both the Sun and from us: not just millions of miles away but many billions of miles away: at least thirty times as far away from the Earth as our world is from the Sun! It moves very slowly out at those great distances, and very quickly when it comes near the Sun: on the order of hundreds of kilometers per second.
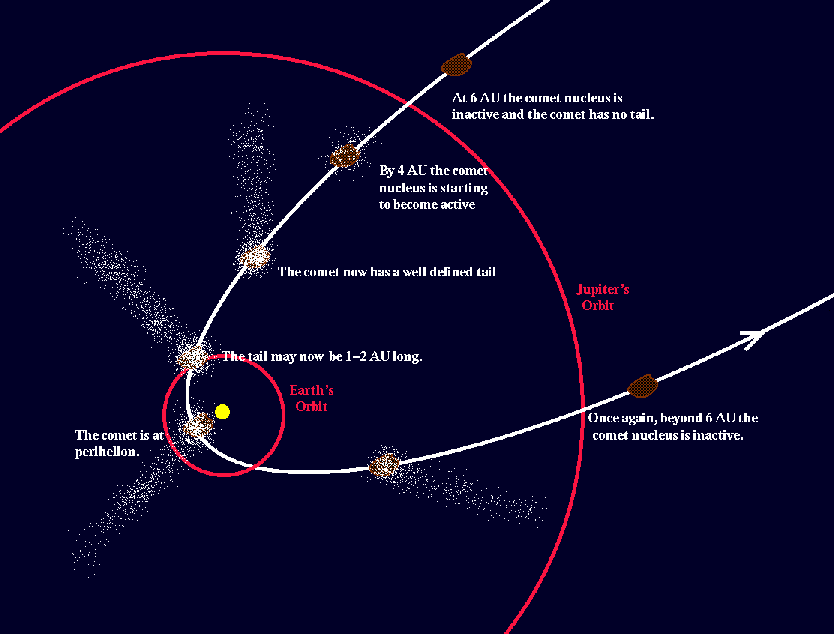
So if we want to land on it, we have to:
- Match its position and its velocity to the comet’s position and velocity.
- Find a suitable, soft landing site that won’t post a danger to the spacecraft.
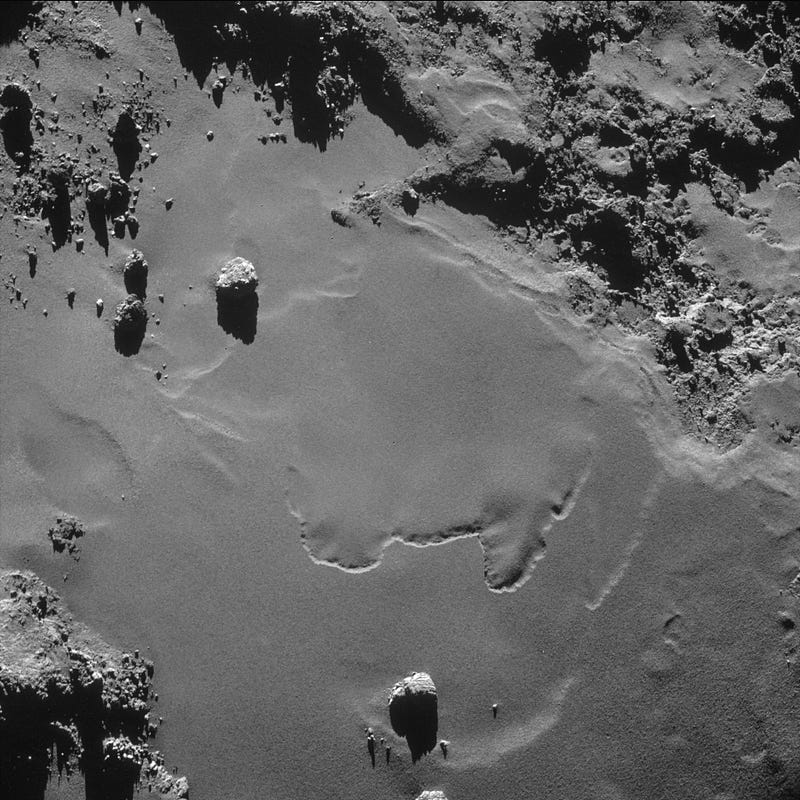
- Find a way to lock in to the comet, so that, as it passes near the Sun and starts to emit particles, the probe doesn’t dislodge.
- And finally, match the comet’s rotation, so that you don’t get “thwacked” by the nucleus’ rotation about its center of mass.
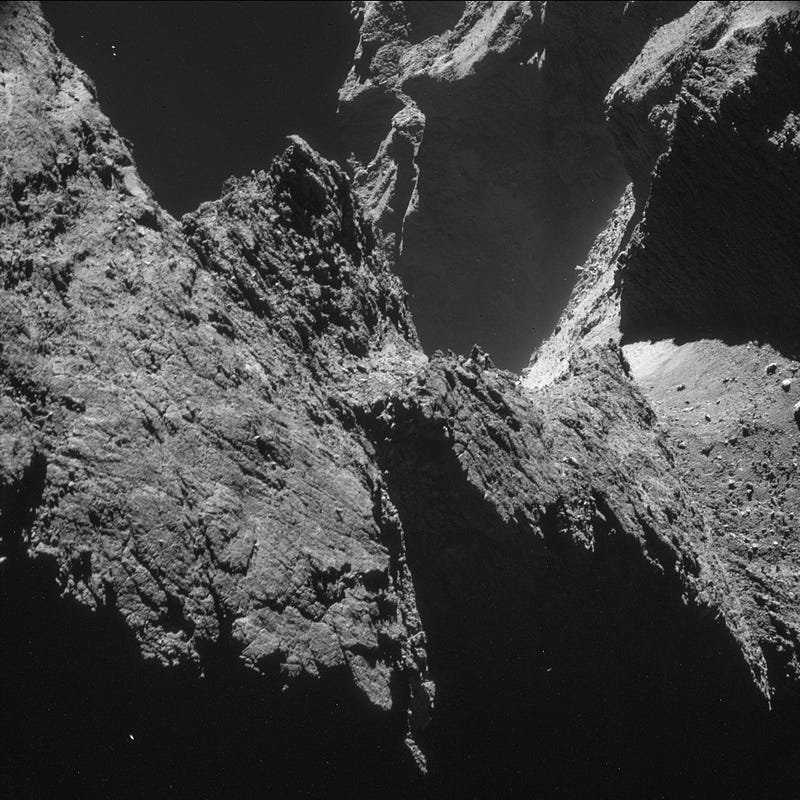
The reason Rosetta’s Philae probe is so impressive is because it’s been preparing for a decade to do exactly all of those things! Its plan, all along, was to have the Rosetta spacecraft track a comet, match its orbital trajectory, and then to approach it ever so slowly. At just the right moment, Philae — basically a sophisticated metal box the size of a dishwasher with scientific tools and instruments — would deploy from Rosetta, leaving its parent satellite behind.
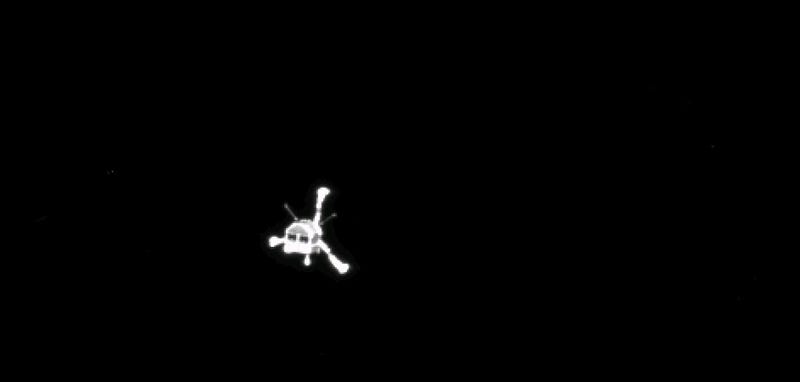
It would then approach the comet’s nucleus — at a relative speed of under one meter per second, or just 0.001% the comet’s orbital speed around the Sun — over a period of seven hours. The landing site would have been carefully chosen months in advance, to ensure that the plan was foolproof. And after landing very softly and gently on it, it then attach itself to it through a twofold series of actions:
- Harpoons shoot out of the probe, attaching it to the nucleus of the comet.
- A series of screws on each of the (three) legs attach them to the comet itself.
This anchors the probe into the comet, preventing it from dislodging as the most climactic part is yet to come. Getting the timing right was incredibly important, because the probe actually bounced when it first landed! Fortunately, it settled in nicely, and held.
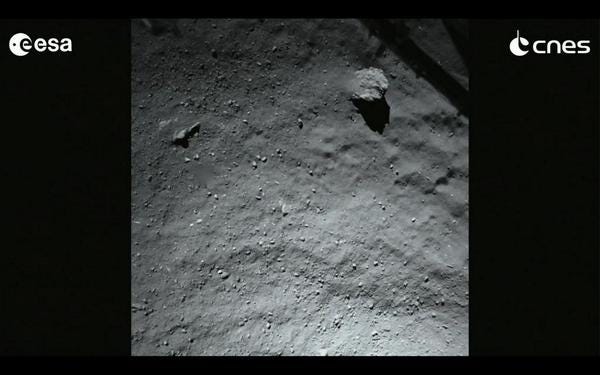
Because the comet is going to approach the Sun, developing a set of tails as it heats up. And when that happens, it will accelerate, losing a maximum of hundreds of kilograms of material every second. The goal of Philae, remember, is scientific, designed to measure how the comet breaks apart, offgasses, loses mass and how it changes phase as well. There are a total of nine instruments on board to do this, an impressive feat in a space probe weighing only as much as about an average American man.
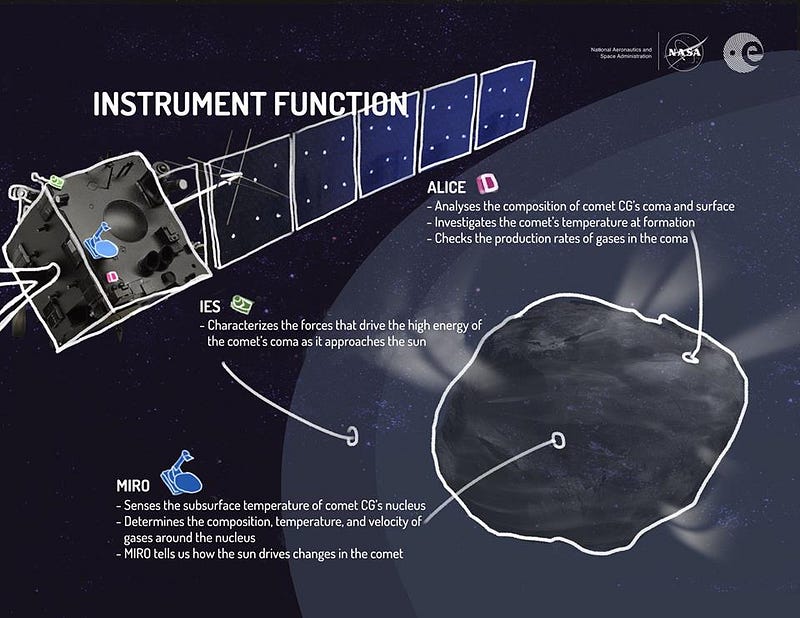
To make these measurements is an amazingly ambitious goal, because the best way to measure this is also the most dangerous to the probe itself: from the comet’s surface!
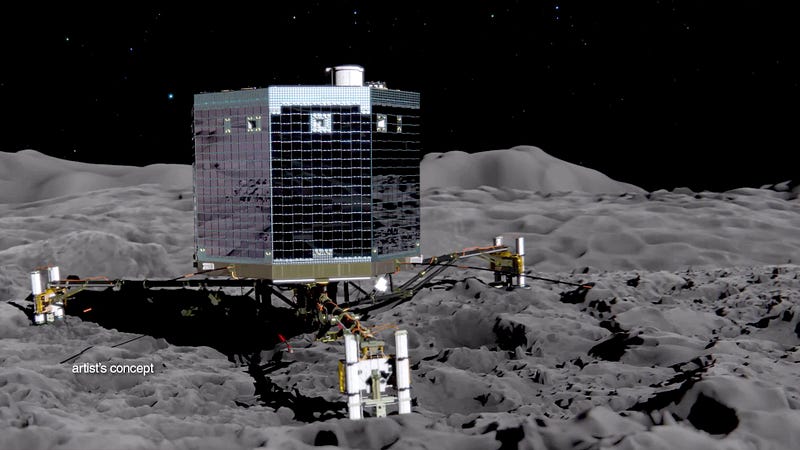
As you’ve no doubt already heard, the landing was successful, and now the comet in question — 67P/Churyumov-Gerasimenko — will hurtle towards the Sun, where it will reach perihelion (or closest approach) in August of next year. Learning how a comet offgasses, and exactly what it emits, how and when, would simply be amazing.
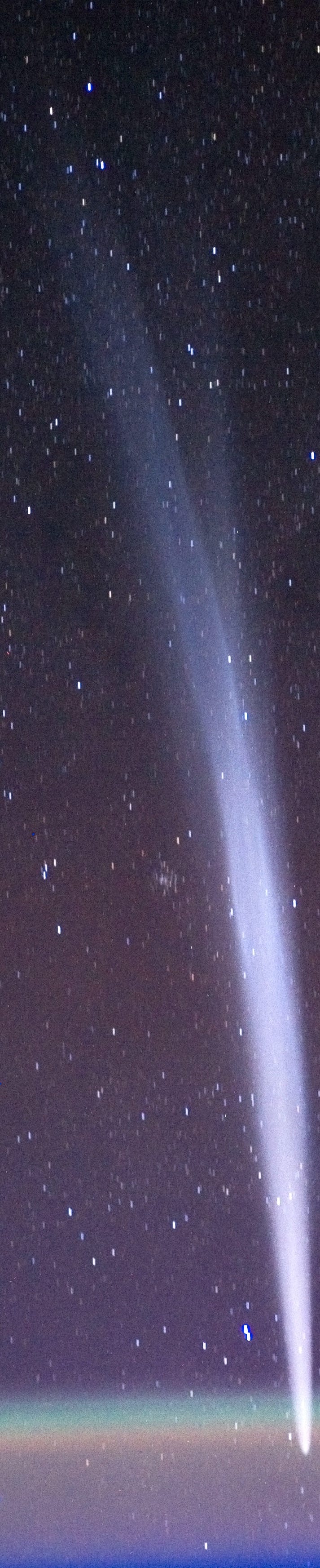
This is a great day for humanity, but the greatest day for science, at least from this mission, will happen a little less than a year from now, when we learn more about not only this one comet than ever before, but about this entire class of objects: the icy worlds in our Solar System from out beyond Neptune.
Congratulations, everyone at ESA, NASA and on the Rosetta/Philae team; the best is yet to come!
Leave your comments at the Starts With A Bang forum on Scienceblogs!