JWST, at last, answers our biggest cosmic questions
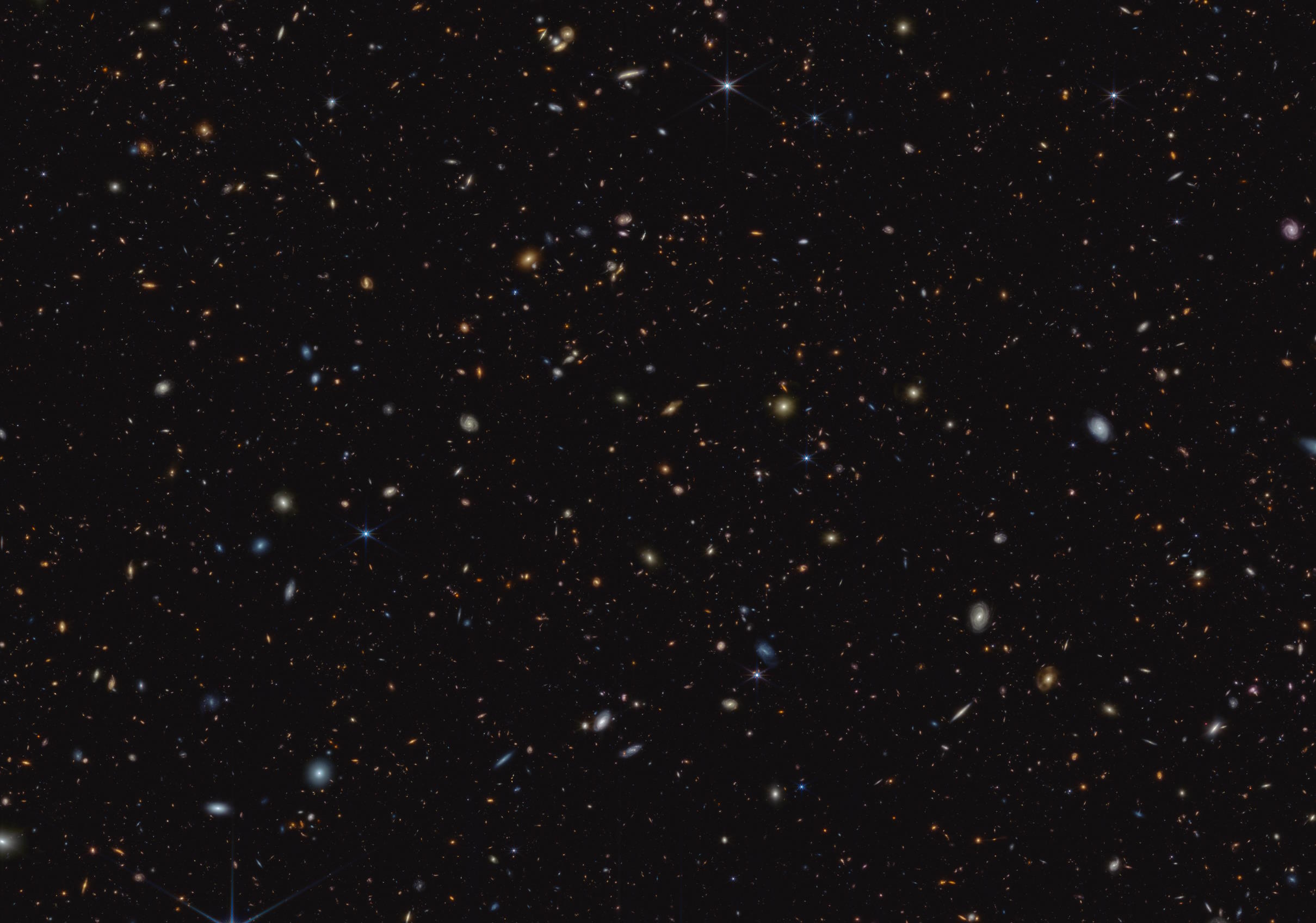
- The Hubble Space Telescope, 33 years into its lifetime, has shown us what the Universe looks like today, with its farthest galaxy reaching back to just 400 million years after the Big Bang.
- But since 2022, we've entered the JWST era: with a larger, more powerful, and better-optimized telescope for seeing back farther into the early Universe than ever before.
- The lessons we're learning are still in their early stages, but all early indications are that we're seeing a Universe that grew up quickly, violently, and full of surprises.
If you want to know what the Universe looks like, all you have to do is look. The better your eyes, the better you’ll see, which is why many of our advances in astronomy have overlapped with advances and improvements in our optical telescopes. We’ve built them progressively larger in size, with 8-10 meter class telescopes presently leading the world from the ground, and with 30 meter class telescopes on the way. We’ve outfitted these telescopes with better, more sensitive instruments that make the most out of each quantum of light they gather, leveraging different wavelength filters, spectroscopy, and a variety of other advanced techniques.
On the ground, we’ve developed unprecedented adaptive optics systems to “un-blur” the distortions imposed by the atmosphere, and in some cases, have even gone up above the atmosphere and into space. With better eyes, the more the Universe comes into focus.
But there’s a limit to what you can do with optical telescopes, and those limits are set by the very Universe itself. As the Universe expands, the wavelength of light that travels through it gets stretched, and this stretching becomes very severe the farther away an object is. Ultraviolet light gets stretched into the optical and then beyond: into the infrared. That’s where the James Webb Space Telescope (JWST) comes in. With its infrared eyes and its position far away in space, it’s truly revealing the Universe as we’ve only dreamed of seeing it, rife with tremendous advances and surprises.
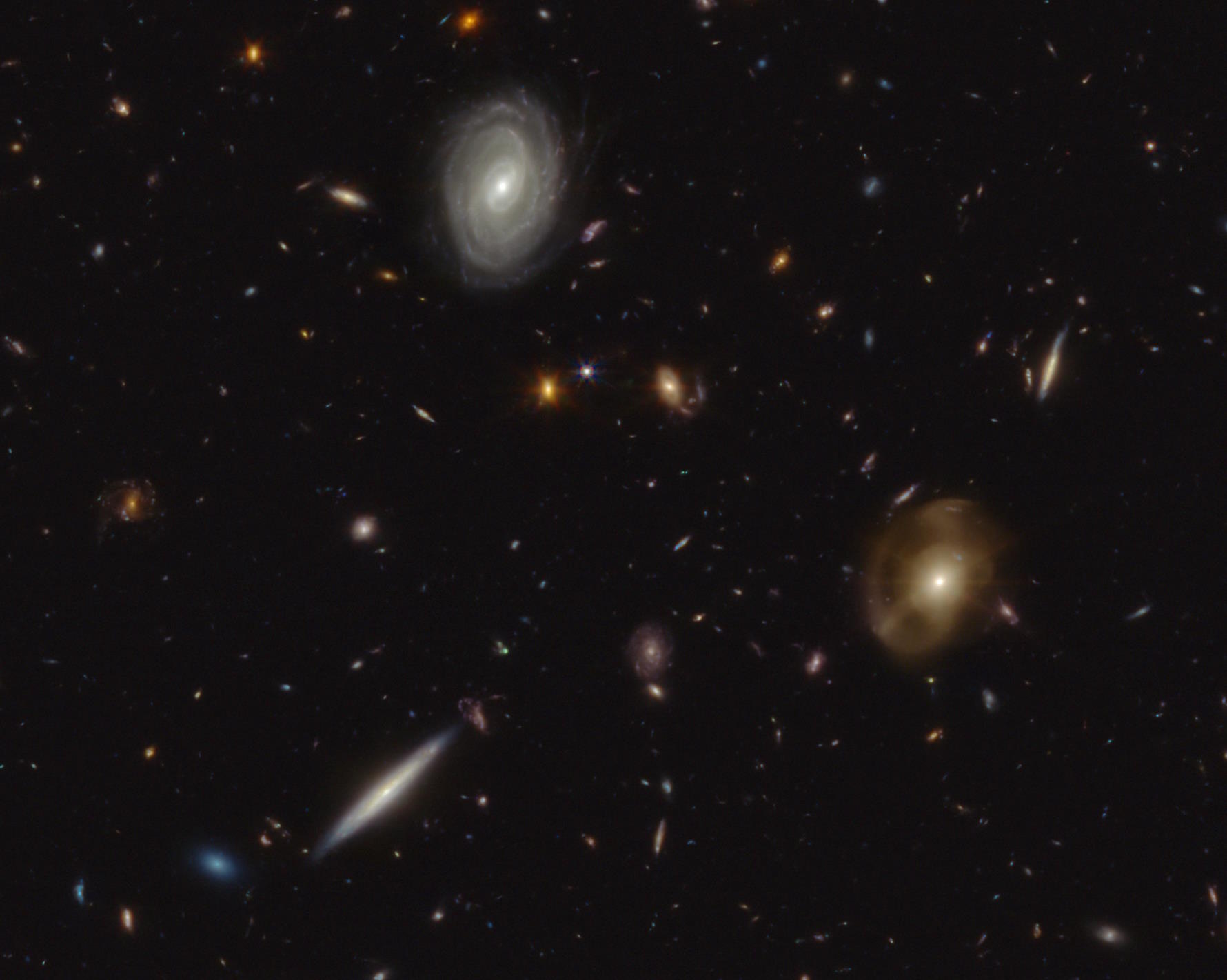
From on the Earth, we’re severely limited by what sorts of light can be transmitted through the atmosphere. We can see optical light very well, but only small fractions of the ultraviolet and near-infrared portions of the spectrum. We can see almost no X-ray or gamma-ray light, and almost no mid-infrared, far-infrared, or microwave light, before things become clear in the radio once again. That’s the huge advantage of space: not only do you remove the blurring effects of Earth’s atmosphere, but some very important wavelengths of light are completely unobservable from the ground.
Hubble provided a remarkable wealth of data, not only in optical wavelengths, but at near-infrared wavelengths as well. The reason Hubble looks like a “tin can” from up close is because we want it to be cold: to reflect as much of the light and heat that encounters it as possible. Infrared light is what we experience as heat, and we know that if things get hot enough they’ll glow in visible light: red, orange, yellow, or even white-to-blue if it’s hot enough. Even if you can’t see it glowing in visible light, objects like the Hubble Space Telescope do emit substantial amounts of light in the infrared. As a result, despite the reflective efforts that have been made with Hubble, it’s only capable of observing out to about ~2 microns in wavelength before thermal noise overwhelms the instruments.
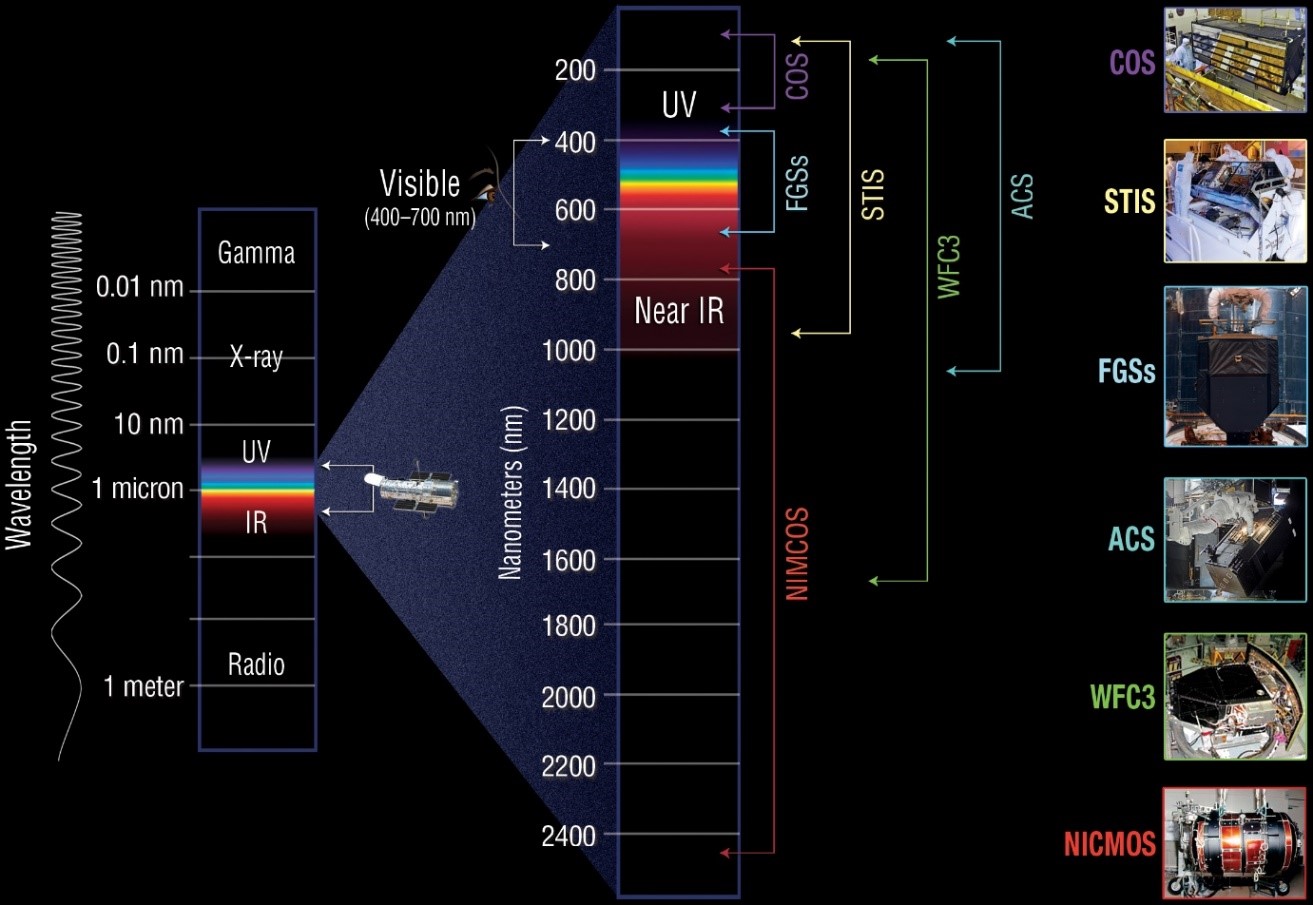
That’s why JWST is so remarkable in a number of ways, at least, from an astronomer’s point of view.
- Instead of being located in low-Earth orbit, it’s located at the L2 Lagrange point: 1.5 million kilometers from Earth. (This way, it isn’t constantly bathed in the direct infrared glow of Earth’s heat.)
- Instead of reflective material, there’s a custom-built 5-layer sunshield that shields the telescope-and-instrument side from the Sun, passively cooling things down to around ~40 K. (As opposed to ~200 K for Hubble.)
- And instead of a mirror configuration and instrument suite optimized for observing at ultraviolet, optical, and shallow near-infrared wavelengths (from about 100-2000 nm), JWST was built to cover a little of the optical, all of the near-infrared, and much of the far-infrared over many wavelength ranges (from about 600-28000 nm), plus the ability to perform spectroscopy on the entire near-infrared range of wavelengths (600-5000 nm).
In order to probe those mid-infrared wavelengths, the instrument that uses that data (MIRI: the Mid-InfraRed Instrument) has to be cooled even farther; it’s the one system that’s actively cooled aboard JWST, all the way down to ~6-7 K. JWST, armed with these capabilities, is capable of seeing galaxies that are too distant, too faint, and whose light has been stretched to too long a wavelength by the expanding Universe to be seen by Hubble.

But it wouldn’t be clear just how good JWST would be, relative to its predecessors, until we looked. The reason is because we’re using it to probe the yet-unobserved Universe: where we don’t have any data yet. Sure, we have expectations for what we think should be there, but the Universe has been full of surprises before, and questions like:
- what were the earliest galaxies like,
- how massive were they,
- how big and bright are the brightest ones,
- at what time do the first pristine stars (that formed from material that’s making stars for the first time) arrive,
- and how quickly, through mergers and accretion events, do these galaxies grow,
are all questions that JWST would be capable of answering for the very first time.
There are five major first-year proposals that sought to answer these questions by looking, deeply, at significant regions of the extragalactic Universe. Two of them, Panoramic and COSMOS-Web, have yet to release any results. Two others, GLASS and CEERS, have found many ultra-distant galaxies, including examples of galaxies that surpassed the earlier Hubble record of GN-z11: a galaxy whose light came to us from just 400 million years after the Big Bang.
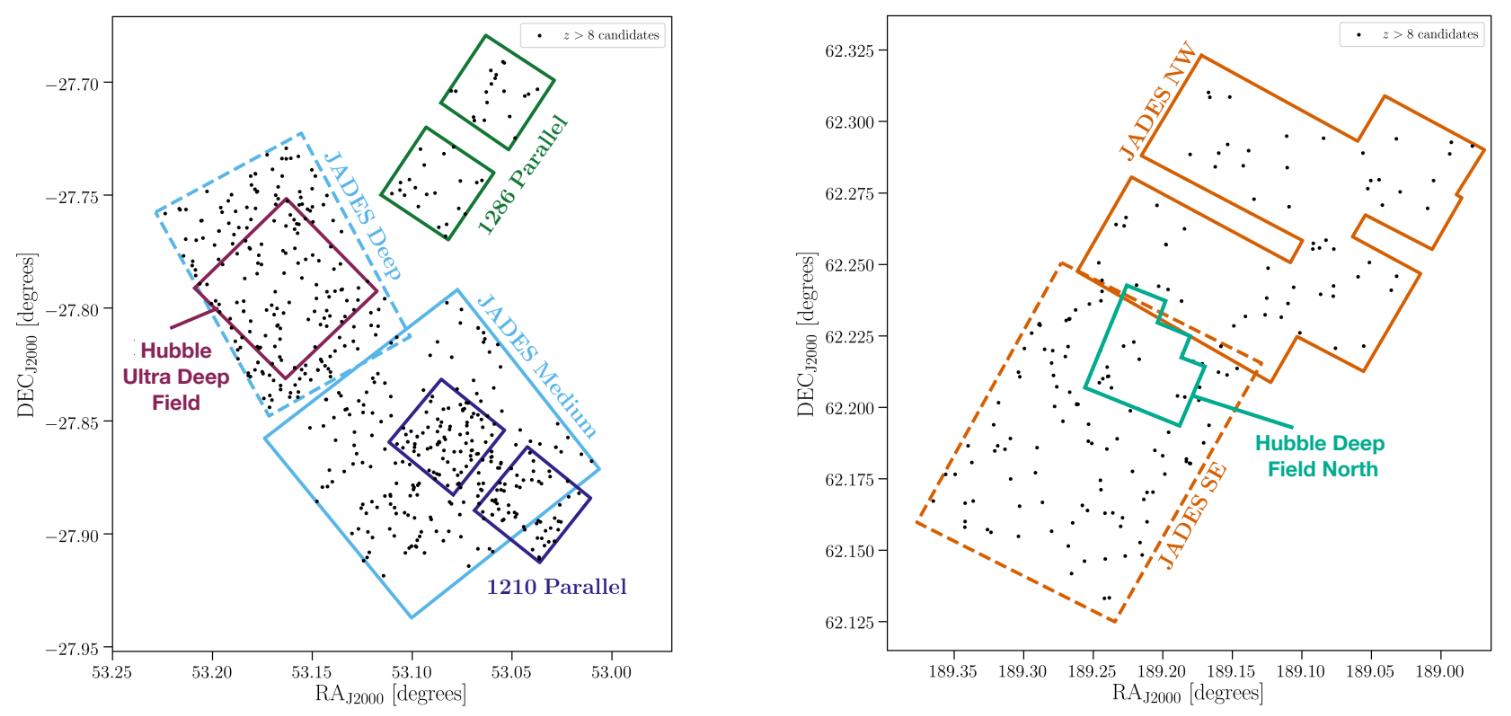
But one of the most interesting regions of space of all comes courtesy of the survey that gave us the current cosmic record-holder for distance (a record that surely will be broken by the end of 2023): JADES. Standing for the JWST Advanced Deep Extragalactic Survey, it combines a total of 770 hours of NIRCam, MIRI, and NIRSpec imaging across a total area of 125 square arcminutes: just under one-millionth (0.0001%) of the total night sky. But that region of sky included two of the most importantly imaged regions in all of history: the original Hubble Deep Field and the Hubble Ultra and eXtreme Deep Fields.
Within these regions of space, there had previously been a few ultra-distant galaxy candidates identified by Hubble: about 40 candidates arising from the first 650 million years of cosmic history, including about 4 from the first 500 million years. The problem is that these are only galaxy candidates: we identify galaxy candidates by looking at their light, but the only way to be certain that these galaxy candidates really are galaxies at the actual distances we think they’re at is to perform spectroscopy: to break their light up into all the different wavelengths that makes it up, and to identify where certain specific features appear. It’s only through spectroscopy that we can promote a galaxy candidate to the status of “confirmed galaxy.”
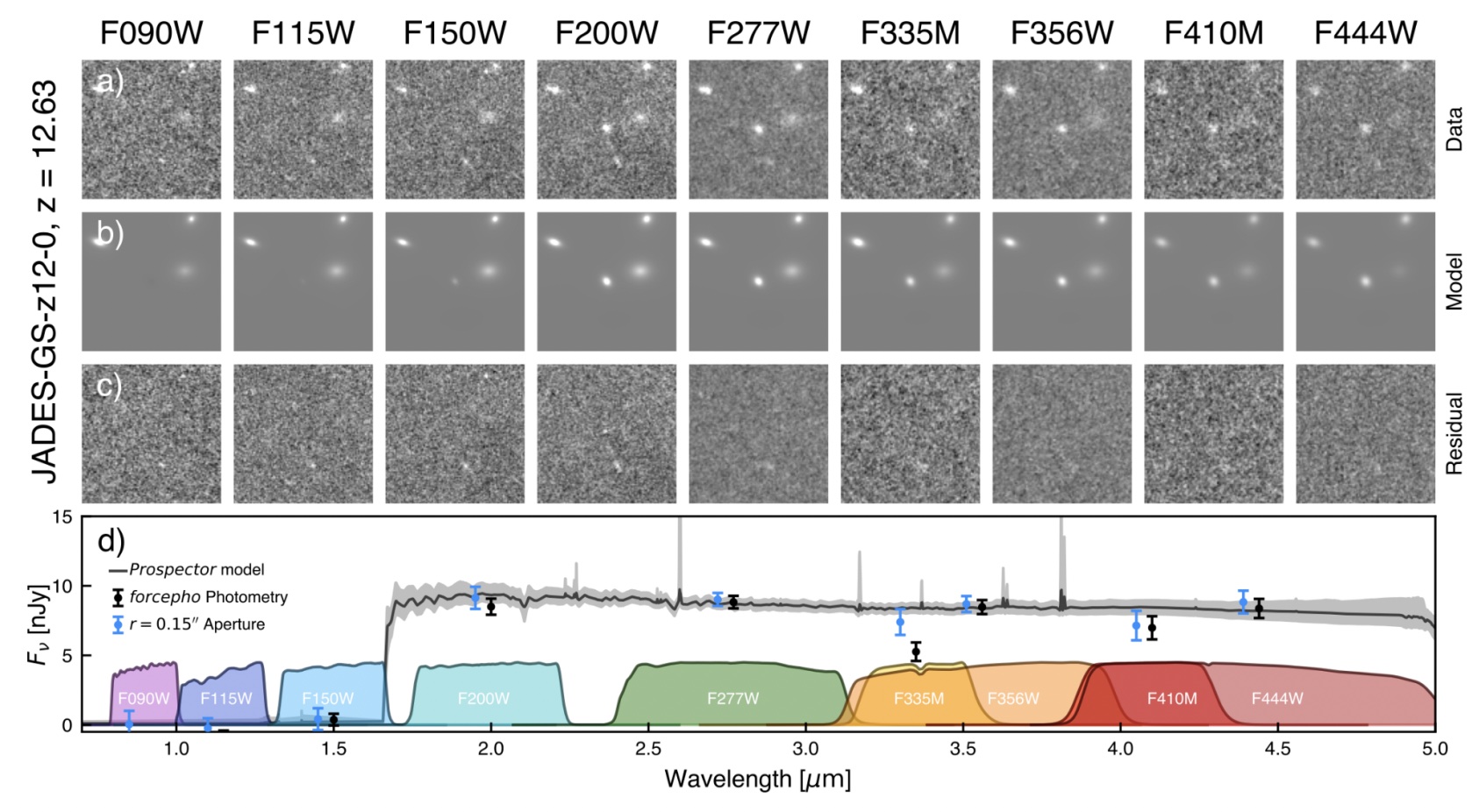
The underlying science is as follows. When you image a galaxy using photometry, the standard way of gathering light over a set of wavelength ranges, you know how that light will be distributed depending on whether that galaxy is primarily composed of young stars, a mix of young-and-old stars, or primarily older stars. (In the late-time Universe, all types of galaxies exist, but early on, we primarily expect galaxies to be made of young stars.) Below a certain wavelength — the ultraviolet limit where electrons transition down to an atom’s ground state — you know that no light will arrive, whereas at longer wavelengths, you should see plenty of light.
That transitional point is key and is known as the “Lyman break” for galaxies: where the transition down to the n=1 state of hydrogen (if you remember the Lyman series) occurs. As the Universe expands, the wavelength of that Lyman break stretches. Therefore, for JWST, if you see no light from the short wavelengths, but plenty of light from longer wavelengths, you have an excellent ultra-distant galaxy candidate.
But in order to make sure that:
- this really is a galaxy,
- that it isn’t a closer, intrinsically red or intrinsically dusty object,
- and that it really is at the redshift/distance combination you think that it is,
you have to perform a spectroscopic follow-up.
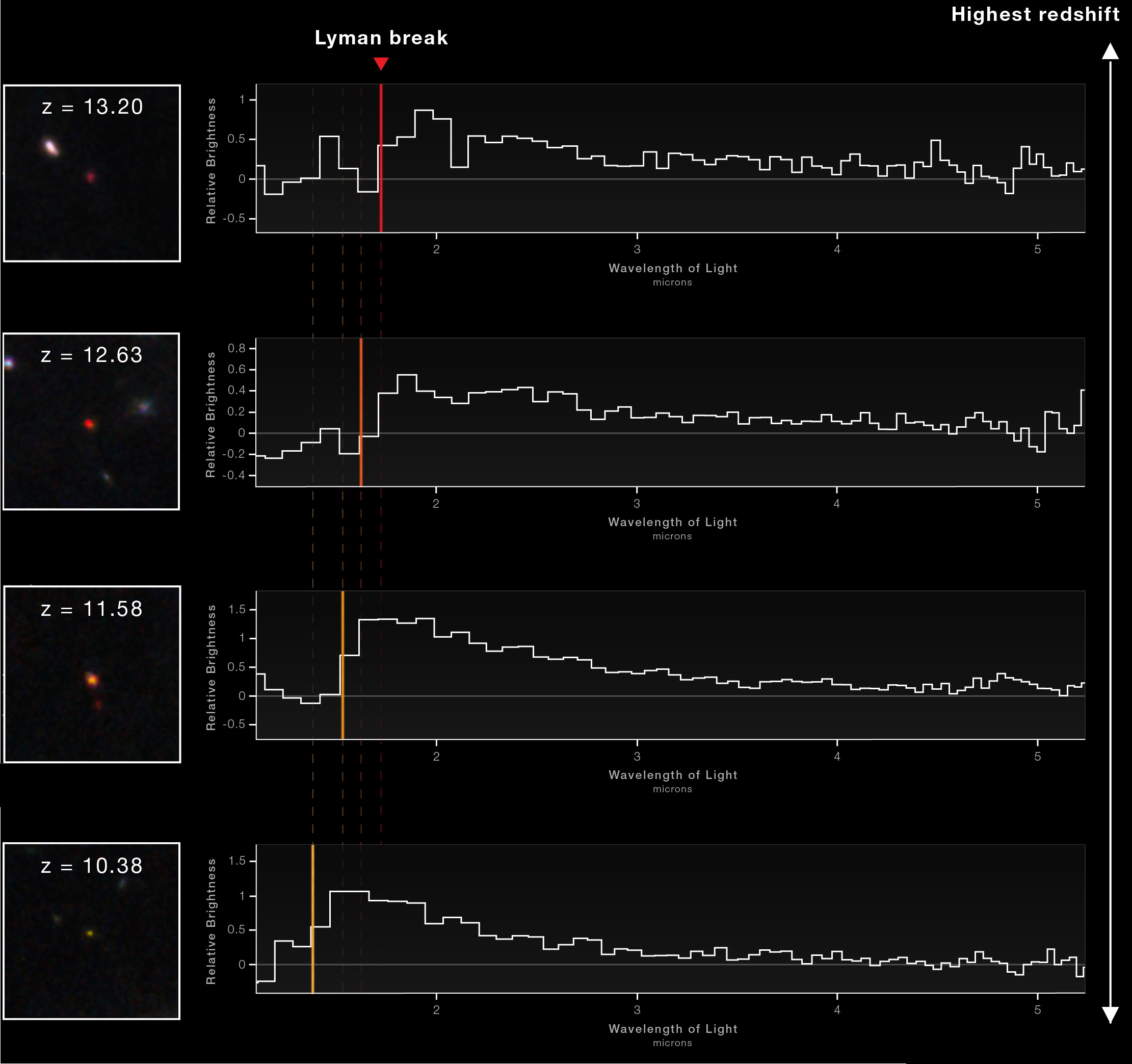
Photometry is relatively easy to perform; you can perform it for thousands of objects all at once with the same sets of observations. Spectroscopy, on the other hand, is expensive: you have to observe for much longer amounts of time, per object, to get the necessary amount of light to determine how much light at each different wavelength there is.
The payoff is tremendous, however: instead of estimating key properties of your galaxy like how far away it is, how much its light is stretched, and how strong its hydrogen, oxygen, and other elemental signatures are, you can measure them directly.
That’s what’s so remarkable and powerful about JADES and other surveys like it performed with JWST: you can view a large area of the sky with an instrument such as NIRCam, obtaining photometric estimates for a galaxy’s properties, relatively easily. You can then pick out the most interesting objects that you’ve identified, through photometry, to perform spectroscopic follow-up observations on, using the NIRSpec instrument, for example. We generally know how our Universe, presently 13.8 billion years old, looks today. But those first few hundred million years — that first 5% of our cosmic history — remains the big question mark that we hope JWST can provide us with answers to.
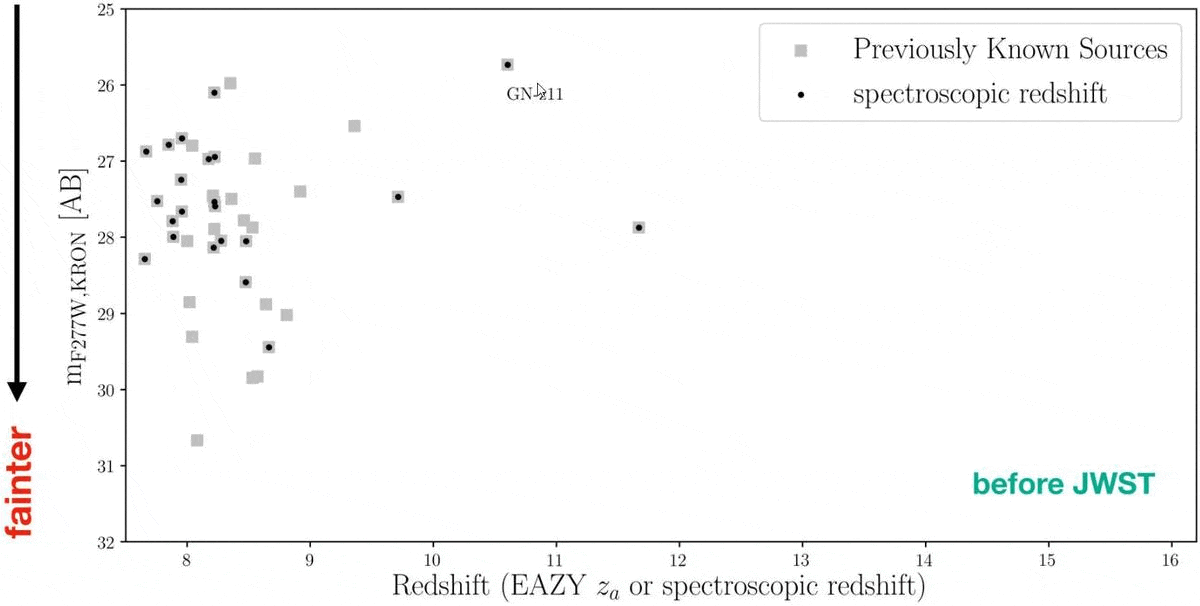
Well, JADES just announced, at the 242nd American Astronomical Society meeting, some of the most remarkable science we could’ve hoped for. First off, across their 125 square arcminutes of observing area, they identified a whopping 717 galaxy candidates from the first 5% of our cosmic history: an incredible improvement over the “about 40” that Hubble had previously seen. In fact, of these 717 candidates that were photometrically identified, a whopping 93% of them had never been seen before — not by Hubble and not by any other observatory — indicating to us that they’ve only been revealed because of the unprecedented capabilities of the JWST observatory.
But the story gets even better. Of these 717 galaxy candidates, spectroscopic follow-up was performed on 42 of them. When the spectra came in, an incredible 41 out of the 42 were confirmed to be at or nearly at the redshift/distance combination suggested by photometry. Even more remarkable was this: the one that wasn’t confirmed turned out to actually be two objects right on top of the other: one close by and one much farther away. When the light from the close-by object (“only” about 11 billion light-years away) was subtracted out, the 42nd object — the farther one — was right in line with the photometric data, too. 42 spectra collected, 42 confirmed ultra-distant galaxies. It’s hard to do better.
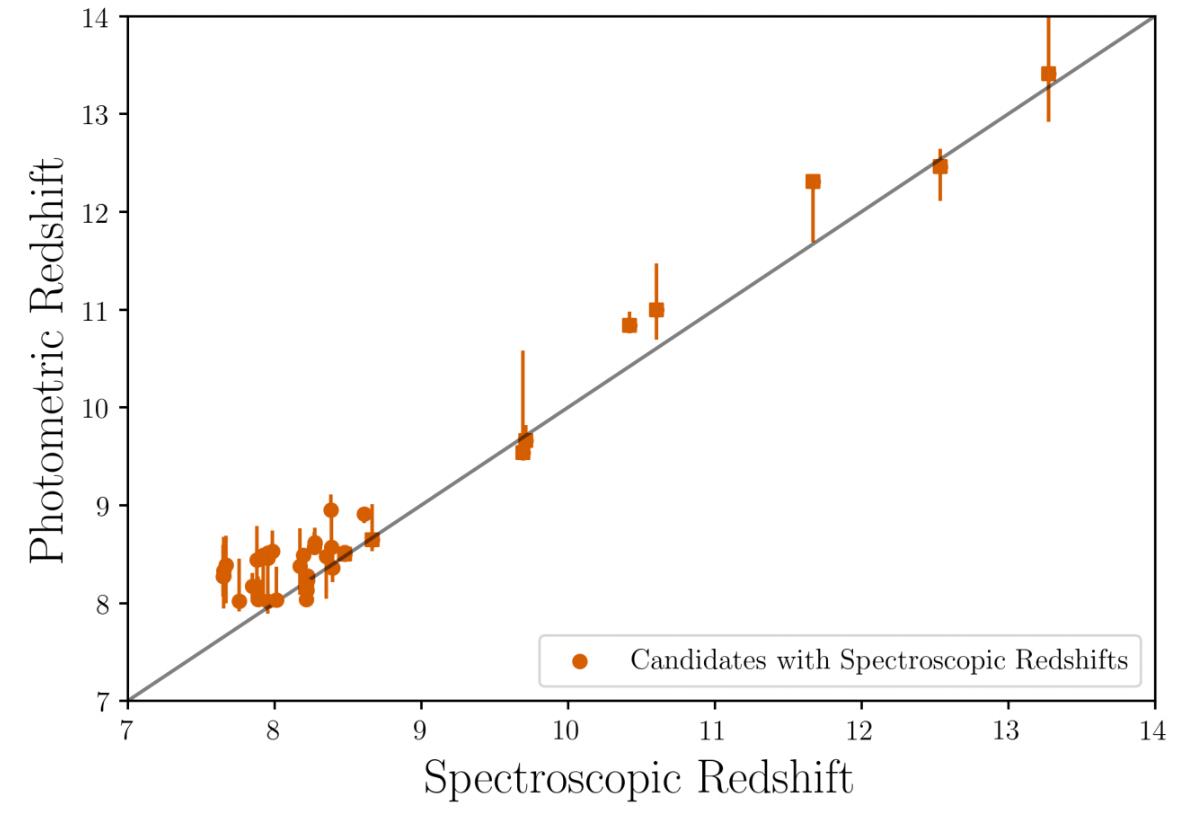
And that’s only getting started. The most distant galaxy confirmed spectroscopically is known as JADES-GS-z13-0, and its light comes to us from just 320 million years after the start of the hot Big Bang. Within just the JADES field-of-view, there are 17 additional galaxy candidates — all of which don’t have spectra just yet — that have greater photometrically-inferred distances than the current cosmic record holder. Not only that, but COSMOS-Web, whose data is all still unreleased (and about 50% of which remains to be taken as of June 2023), will be surveying a much larger area on the sky than JADES ever will.
But because of the combined effects of the unprecedented size and resolving power of JWST, we can learn a tremendous amount about the Universe by looking at these galaxies; they’re not simply “points” or “smudges” to JWST like they were to an observatory like Hubble.
These galaxies reveal major bursts of star-formation within them. The hot, massive stars that occur during these bursts are enormous contributors to the process of cosmic reionization: where the neutral atoms in the intergalactic medium become reionized thanks to ultraviolet photons. The emission lines within these galaxies are extremely strong. And finally, these galaxies come in an enormous variety of sizes, from only a few hundreds of light-years wide to tens of thousands of light-years wide, demonstrating that many of the objects in our Universe grew up quickly: perhaps more quickly than many astronomers expected.
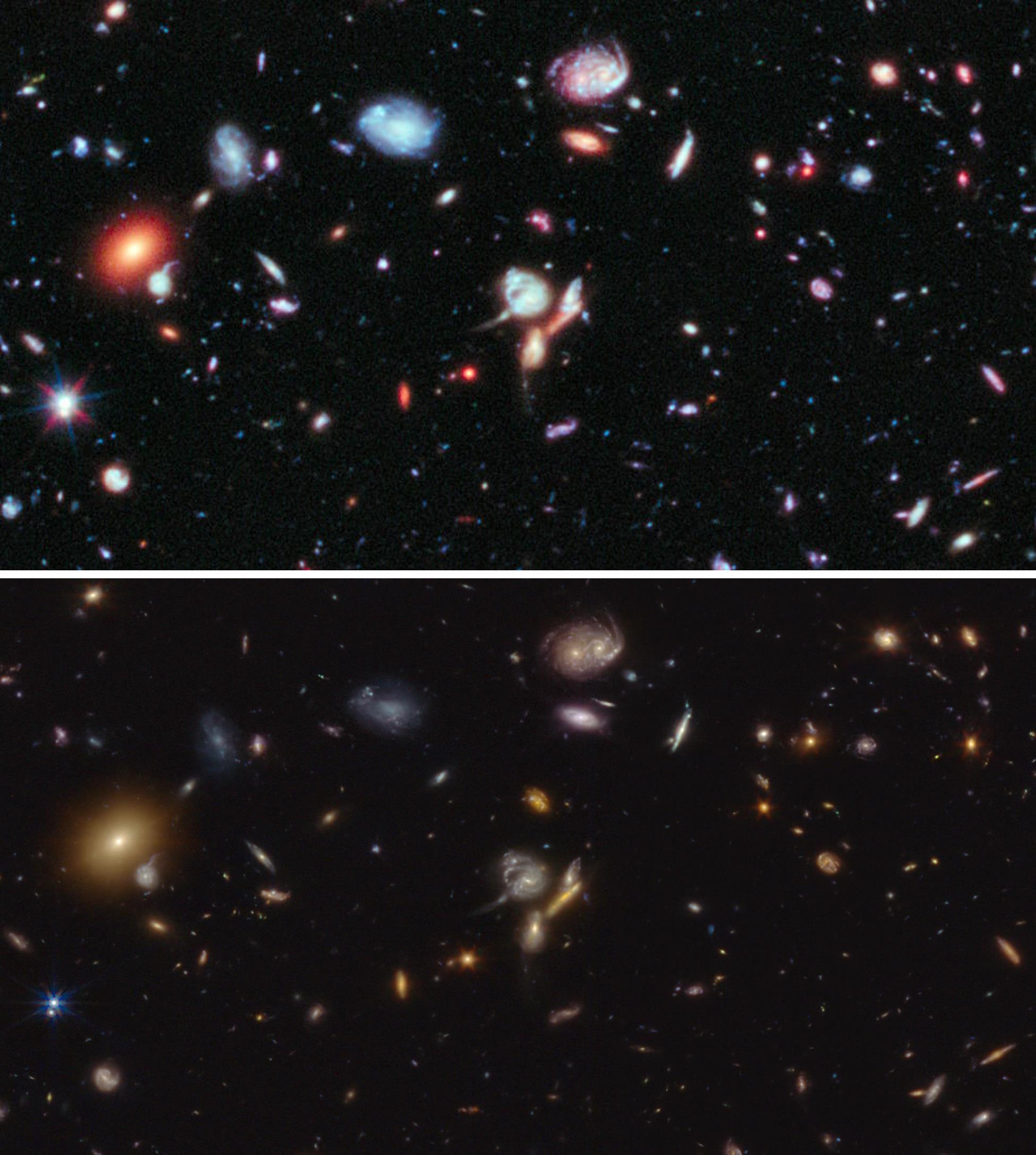
We’ve come far enough to put together the broad strokes of how our Universe grew up, and it’s looking like a story that will lead to decades of additional research to robustly put all of the pieces together.
- The very first stars must’ve formed long before JWST is observing: likely within a time period just 100-200 million years after the Big Bang.
- The earliest galaxies that we see are likely the brightest and most massive ones from their time, and they exist in great numbers ~500 million years after the Big Bang, and quite abundantly even 300-400 million years after the Big Bang.
- Many galaxy candidates exist from when the Universe was only 250-300 million years old, and there’s every reason to hope that many of them will truly turn out to be confirmed galaxies once all is said and done.
- And that the technique of photometric redshifts is remarkably successful for the galaxies it’s been applied to so far; whether it still works for the most distant galaxy candidates of all still remains to be tested, however!
All of this JWST science that we’re incorporating into our set of knowledge is, for all of it, less than a full calendar year old. As more data continues to pour in from the telescope, and as different teams using different observational schemes publish their results, we’ll learn how to use JWST even more efficiently and effectively. It’s that glorious case where whenever we learn something new, the whole community benefits. With an expected lifetime that will take it well into the 2040s, we have decades of new science, new discoveries, and a new understanding of how the Universe grew up to look forward to with great optimism.