Is It Dark Matter? Mystery Signal Goes ‘Bump’ In World’s Most Sensitive Detector

You never know what you’re going to find when you look somewhere new for the first time.
Approximately 4600 feet (1400 meters) underground, beneath the Italian mountain known as Gran Sasso, scientists from the international XENON collaboration have built the world’s most sensitive dark matter detector. For years, the XENON collaboration has searched for any and all evidence of a mysterious particle that goes beyond our Standard Model, setting numerous records for humanity’s most stringent limits on what dark matter can (and cannot) be.
With more data than ever before, a surprising signal has emerged above the expected background in an unexpected place: at low, rather than high, energies. There are three possible explanations that we know of:
- it could be an unaccounted-for contaminant, like tritium,
- it could be that neutrinos have a surprising property, different than what the Standard Model predicts,
- or, most excitingly, it could be our first evidence for a special type of light dark matter, such as an axion-like particle.
The science behind this mysterious signal is remarkable, regardless of the cause.
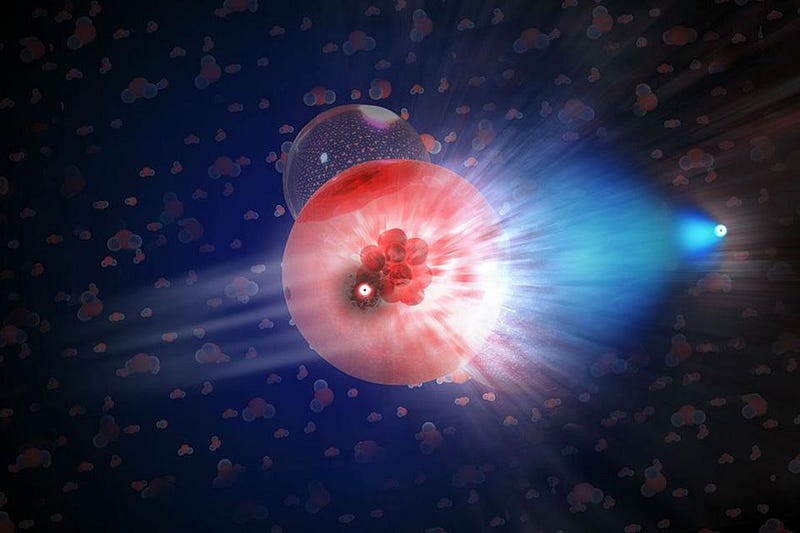
If you want to find something that’s elusive, you have to be a very clever detective. You cannot just build a detector capable of observing the events you’re seeking; you also have to shield that detector from every other source that could possibly create a contaminating signal. In order to see anything meaningful, the desired signal has to rise above the noise of the experiment, and that’s the tricky part.
The XENON collaboration, for more than a decade, has been working on exactly this. Their experiment is performed underground beneath a mountain, to shield it from cosmic particles originating from space and the atmosphere. It’s filled with more than 3 tonnes of ultra-pure liquid xenon, which serves as the “target” for the experiment. It’s surrounded by photomultiplier tubes to pick up the signals of even single, charged particles, and has an enormous water tank to capture any stray muons. In short, it’s a remarkable feat of engineering.
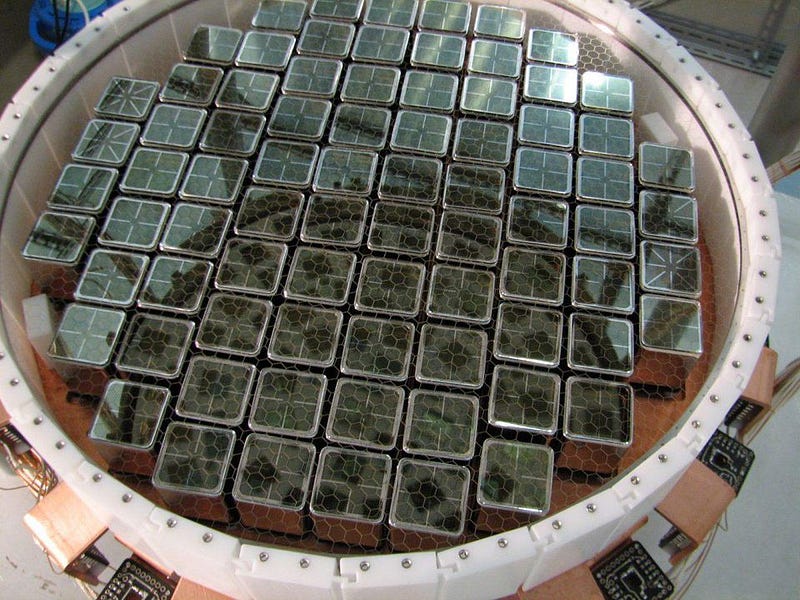
All told, there are some ~10²⁸ xenon atoms that serve as possible targets within the current iteration of the XENON detector. (This has been scaled up by more than a factor of 100 from the original version of the experiment, dating back to 2006 or so.) Whenever a particle — regardless of its source — enters the detector, it has a finite probability of interacting with one of the xenon atoms.
Unfortunately, most of these interactions occur from particles already known to exist, including:
- radioactive decays,
- stray neutrons,
- cosmic rays,
- muons,
- and neutrinos,
all of which constitute the background signal that cannot be removed. In other words, that’s the noise that’s present. If you want to observe a signal, it needs to be strong enough to be visible over and above this noise.
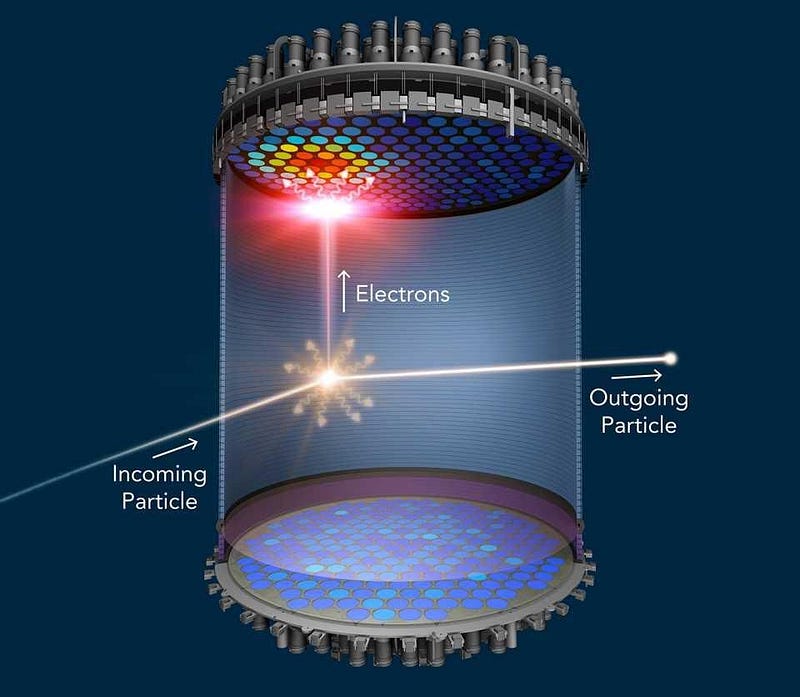
Experiments like XENON, although they’re primarily designed to search for WIMP-like particles, are actually sensitive to a wide variety of energy ranges. Although the most highly anticipated signals were expected to occur in the ~GeV range of energies (where 1 GeV corresponds to 1 billion electron-volts), what XENON actually saw — according to the new release — was a tiny but significant excess of events at just a few ~keV in energy: thousands, rather than billions, of electron-volts.
Because of how well-shielded and well-calibrated the XENON detector is, they expected only 232 background events from the entire experiment in the relevant low-energy (1-to-7 keV) range. And yet, when they examined their results, they found a total of 285 events: 53 more than expected. This might be a tiny amount, but it’s incredibly significant. For the first time ever, at such a high level of confidence, the XENON collaboration has seen something that goes beyond what’s expected from the Standard Model.

Regardless of the source, this is an incredible technical and scientific achievement. Over the years, many experiments have claimed to see an excess of dark matter particles at a variety of energies, and the XENON collaboration has always provided a sanity check on all of them. If those claims had been correct, there should be a corresponding signal in the XENON detector. Despite all the claims made in the media, XENON has only ever returned “null results” before; no new signal had ever been found.
But this time, it’s a different story. For the first time, this detector has revealed an excess of events above and beyond the expected background from all known sources. It’s possible (but statistically very unlikely) that this is just an uncommon random fluctuation, but the excess is too large for that to be a compelling explanation. Instead, there are three plausible scenarios that could be responsible for this.

1.) Contaminated tritium. One of the problems with backgrounds in the XENON experiment arises from unstable cosmic particles — muons (the heavier cousins of electrons) — that interacts with or decays inside the XENON apparatus. These muons cannot be avoided, but they can be understood and subtracted out by building a large water tank around the XENON detector: something the collaboration has already done.
However, water contains hydrogen, and hydrogen comes in three different isotopes: a single proton, a deuteron (which includes a neutron), and tritium (which includes two neutrons). Tritium is radioactive, and just a tiny amount of it in either the XENON target or in the surrounding water tanks — corresponding to just a few thousand tritium atoms, total — could account for the entirety of the excess. There is not yet an independent way to measure such a small amount of tritium, but it’s an important (although mundane) possibility to keep in mind.
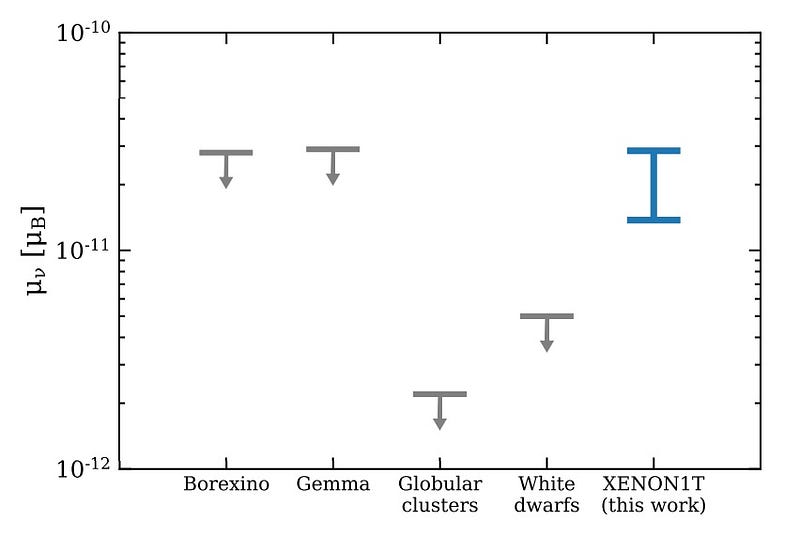
2.) Neutrinos have a magnetic moment. If you placed a neutrino in a magnetic field, it shouldn’t respond at all. According to the Standard Model, neutrinos, as uncharged point particles, should have a negligible magnetic dipole moment, some ~20 orders of magnitude less than the electron’s dipole moment. But if they did have a large enough magnetic dipole moment — perhaps a billion times greater than the Standard Model’s predictions — this could explain the excess of events seen by XENON.
Unfortunately, this explanation is already disfavored by two independent sources: by the Borexino experiment, which has placed direct constraints on the neutrino’s dipole moment, and the cooling of both globular clusters and white dwarf stars, which place indirect constraints that are even tighter. Unless something is amiss with these prior studies, the explanation involving a neutrino magnetic moment cannot stand on its own.
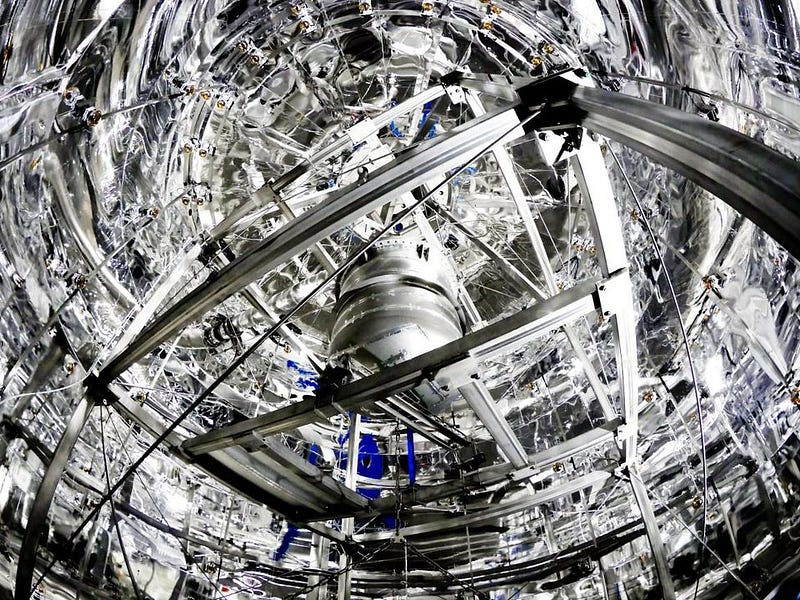
3.) Axions produced in the Sun. One of the more exciting options for dark matter is a particle called the axion: a very light particle produced in the transition that enables protons and neutrons to stably form from a sea of quarks and gluons. Although this is where the overwhelming majority of axions will come from — if they exist and if they make up the dark matter — there are two other places axions are produced: in the Big Bang and in the interiors of stars.
That last source includes our Sun, of course. And if axions exist and make up (at least some of) the dark matter, these solar axions could be arriving in the XENON detector. They’re a remarkable and plausible explanation for this signal, and this could be the very first hint of their existence. (The ADMX experiment, which searches for them directly, has so far come up empty.) If this mysterious “bump” in the XENON data is connected to dark matter, solar axions is the most likely mechanism to explain how.
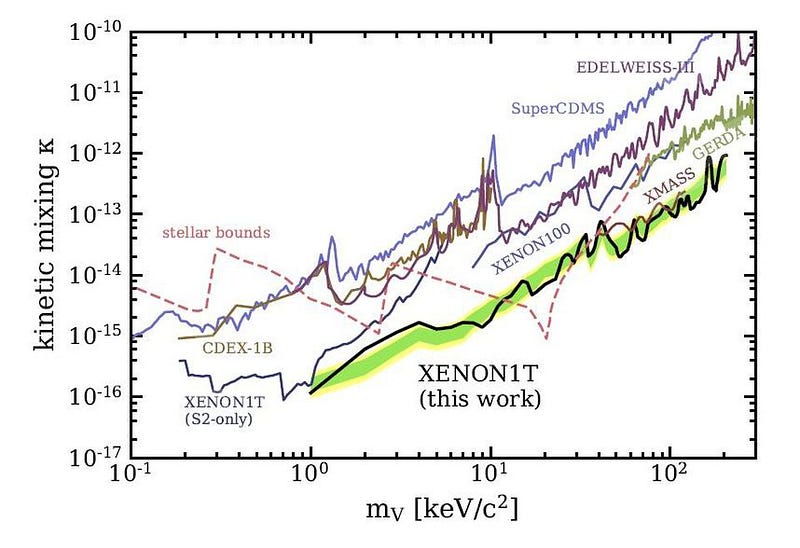
What’s not up for debate, however, is the idea that XENON has directly seen evidence for light dark matter: a pseudoscalar particle or a vector bosonic dark matter scenario, for instance. Even if they allow the mass of the candidate particle to vary wildly, there is no significant signal that emerges against the background for these models. Something else — maybe tritium, maybe neutrinos, or maybe solar axions — must be at play to explain the observed excess.
Instead, the new results from the XENON collaboration place the strongest constraints ever on these two models of dark matter, outstripping constraints from all other experiments as well as astrophysical observations. Only in one narrow mass range are stellar bounds more restrictive; the XENON collaboration has now directly constrained numerous options for dark matter more stringently than ever before.
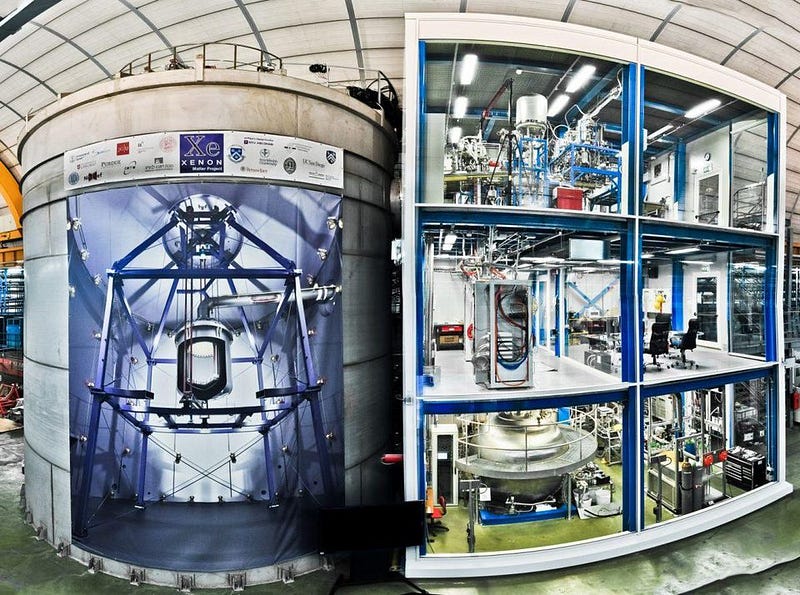
It’s a remarkable feat that the XENON collaboration has achieved by collecting so much high-quality data in such a pristine environment, a triumph for experimental physics regardless of the results. It’s a happy surprise, however, that something is definitively causing an excess of events in a very specific low-energy range (from 1-to-7 keV) in the detector itself.
It might just be tritium in the water; a few thousand tritium atoms in the entire apparatus could be the culprit. It could be that the neutrino has a large magnetic moment, but other observations conflict with that interpretation. Or, it could be axions — a specific dark matter candidate particle — produced by the Sun that are confounding the detector.
Either way, there’s a new mystery afoot. Something just went “bump” in the world’s most sensitive detector experiment, and it might be our first direct clue as to the nature of the Universe’s most elusive source of mass: dark matter.
Ethan Siegel is the author of Beyond the Galaxy and Treknology. You can pre-order his third book, currently in development: the Encyclopaedia Cosmologica.