Ask Ethan: How will the Universe end?
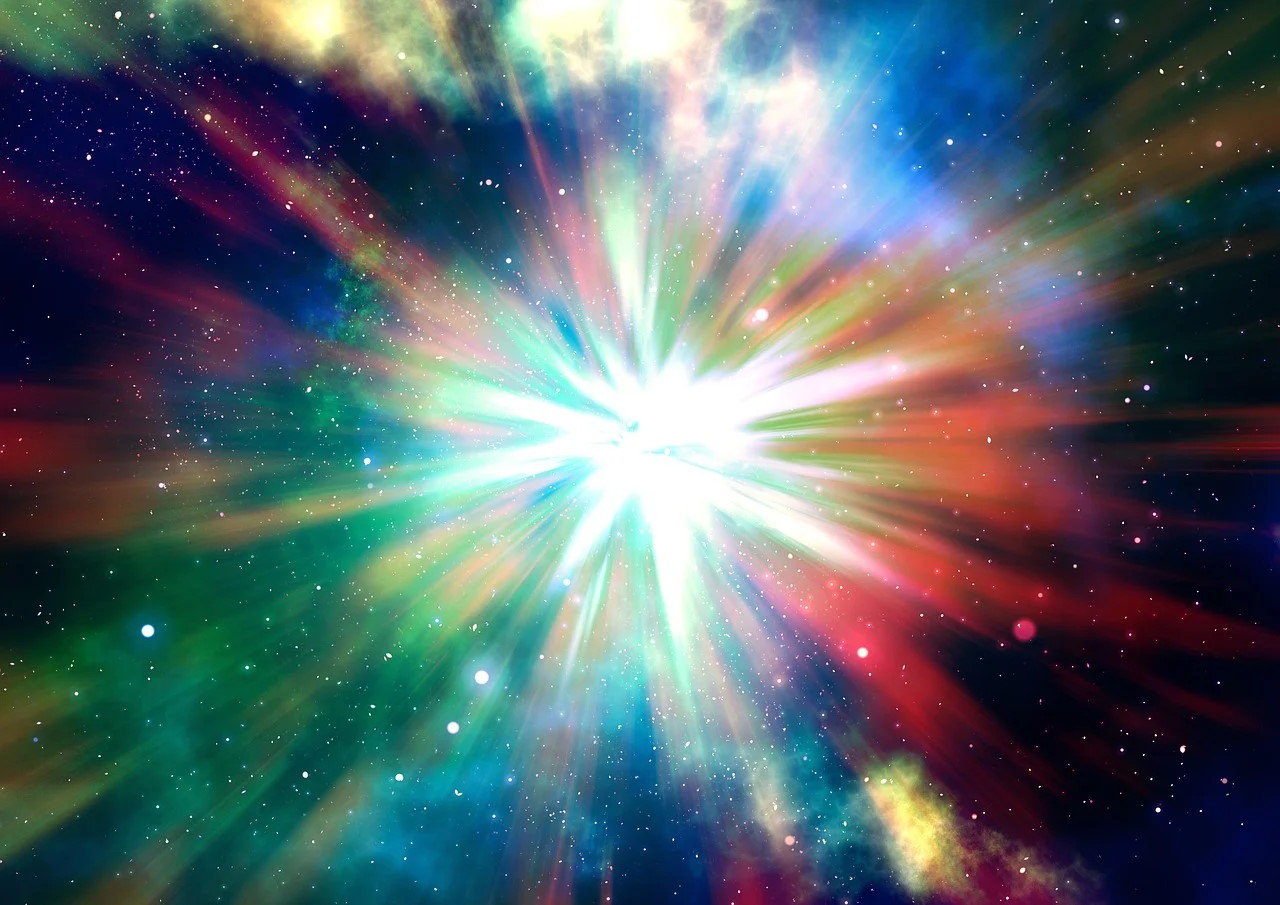
- 13.8 billion years ago, the hot Big Bang occurred, giving rise to an initially hot, dense, uniform, matter-and-radiation filled, and rapidly expanding state: the start of our Universe as we know it.
- But of the possible fates that exist, including a recollapsing Universe, a coasting Universe, or one that relentlessly expands forever, the data firmly supports the last option.
- If that's our ultimate fate, then how will the rest of our cosmic history unfold? Find out what happens as time proceeds to infinity in this edition of Ask Ethan!
For countless generations, humanity has pondered the grandest questions of all about our very existence, with no sign of sufficient evidence to guide us toward the answers.
- What is our Universe?
- Where did it come from?
- How did it get to be the way it is today?
- And what will its ultimate fate be?
Historically, questions like these fell to the philosophers, poets, and theologians to answer. But starting in the 20th century, all of that began to change, as we compiled a large suite of scientific evidence from the Universe itself that pointed toward verifiable solutions. Today, we can confidently state the answers to many aspects of these questions and more.
But what does that mean for the journey, from here to eternity, of how the rest of our cosmic history will unfold? That’s what Tom Kronmiller wants to know, noting that our Universe:
“Is an expanding universe which won’t collapse back later into a new Big Bang. So what does happen as time proceeds to infinity? Eventually our galaxy is the only thing we can see? Do the stars all die and drift in the dark? Does the central black hole eventually swallow everything? If so, does it eventually decay to nothing?”
There are many, many events that we can confidently predict will happen as time marches forward. Based on our current understanding, here’s how it will all come to an end.

Right now, we have tremendous confidence in what the current status of the Universe is. Yes, there are some uncertainties in there at the ~few percent level, at most, but we know that our Universe is:
- expanding,
- uniformly in all directions,
- at a rate of around 70 kilometers-per-second for each megaparsec of distance we cover,
- with its energy contents divided among its various components, including dark energy (68%), dark matter (27%), normal matter (4.9%), neutrinos (0.1%), and photons (0.01%).
Our Universe is also filled with structure: planets, stars, galaxies, groups-and-clusters of galaxies, and cosmic filaments that create a web-like structure on even grander scales. The objects that have accumulated enough matter — the ones that have gravitated significantly enough over long enough periods of time — are no longer expanding; they’ve created bound structures. But individually bound structures that aren’t bound to one another will continue to expand away from one another as time goes on.
The Local Group, the M81 Group, the Leo Group, and the Virgo Cluster are all impressive examples of nearby groups and clusters of galaxies that are all individually bound, but that continue to expand away from one another as the Universe continues its expansion.
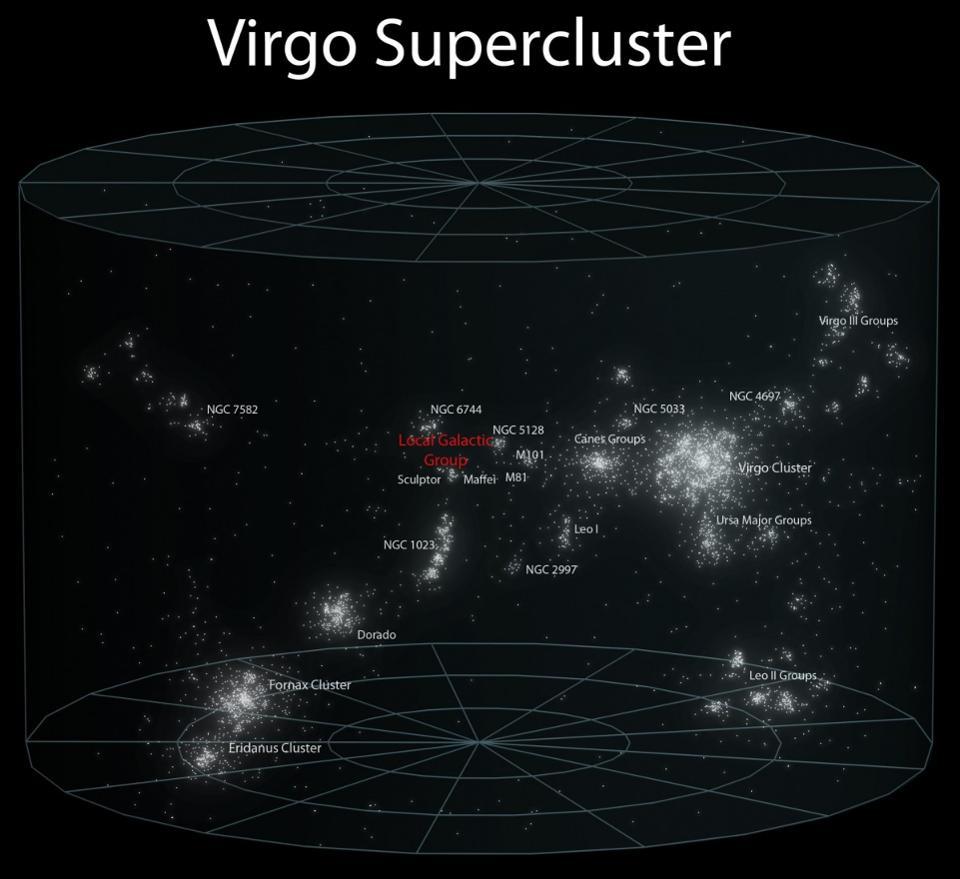
This tells us that our Local Group will hold together as the Universe continues to expand; the galaxies within about ~4-5 million light-years of the Milky Way will remain gravitationally bound together. However, the galaxies beyond the Local Group, while they may remain bound to their own galaxy groups or galaxy clusters, won’t become part of our Local Group or become gravitationally bound to us in any way. Each individual collection of bound galaxies can remain as a collection of bound galaxies, but — other than the galaxy groups and clusters that are already bound, and are in the process of merging together — larger-scale structures will split apart due to dark energy and the accelerated expansion of the Universe.
On timescales of a few billion years, we’re pretty confident of what will happen, both locally and cosmically. The Sun will heat up, swell, boil Earth’s oceans, and eventually become a red giant, dying shortly thereafter. The Local Group will remain bound, and the galaxies within it will attract and merge together, triggering new star-formation and depleting our gas. Four billion years from now, the Milky Way and Andromeda will merge together, creating a new galaxy known as Milkdromeda.

By the time another 10-to-15 billion years have passed, all of the galaxies in the Local Group should experience a gravitational encounter with Milkdromeda, where they’ll be devoured and cannibalized. As the gas within these galaxies forms new generations of stars, it also gets depleted; over time, the star-formation rate will continue to plummet. Without the fuel for forming new stars in great numbers, old stars will die faster than new ones are being born; the number of living stars in our galaxy will start to drop.
Meanwhile, all the other groups of galaxies will accelerate away from our own. Just as the Local Group becomes more concentrated, so will the other groups and clusters, but only individually. On larger scales, they’ll not only continue to speed away from one another, but the rate at which they appear to recede will increase over time. This is what is meant by the accelerated expansion of the Universe: each individual galaxy that’s not gravitationally bound to our own will appear to recede from us faster and faster as time goes on. Eventually, all of the galaxies that aren’t part of the Local Group will recede from us faster than the speed of light; with each passing moment that goes by, additional stars and galaxies become forever unreachable. 94% of the galaxies within the observable Universe, believe it or not, are already there.

But within our Local Group, the decline of stars, planets, and life will be very slow. After only a few billion years, all stars more massive than the Sun will burn out, leaving only the cooler, redder, lower-mass but longer-lived stars behind. As the Sun-like stars die, they blow off their outer layers into planetary nebulae, which returns large quantities of hydrogen and helium gas back into the interstellar medium. As new gas begins to populate the galaxy, it will form molecular clouds that gravitationally grow. After enough time, those clouds will gravitationally contract, leading to a continuing trickle of star-formation, after all.
Meanwhile, both stars and stellar remnants will gravitationally interact within the galaxy. Over time, the heavier masses will be driven to the central region of Milkdromeda, while the lighter masses will receive high-velocity kicks, hurling them into the galactic outskirts or even ejecting them from the galaxy entirely. This will lead to a series of events:
- black holes will collect in the galactic center, eventually merging together,
- stars and brown dwarfs will collide with one another, creating heavier stars,
- the galaxy will develop a central concentration and a diffuse halo of stars,
- stellar systems will begin to lose their Oort clouds, Kuiper belts, and even planets due to close passes with other stars,
- even the lowest-mass stars will run out of fuel and die,
- and many of the lightest stars will be ejected entirely.
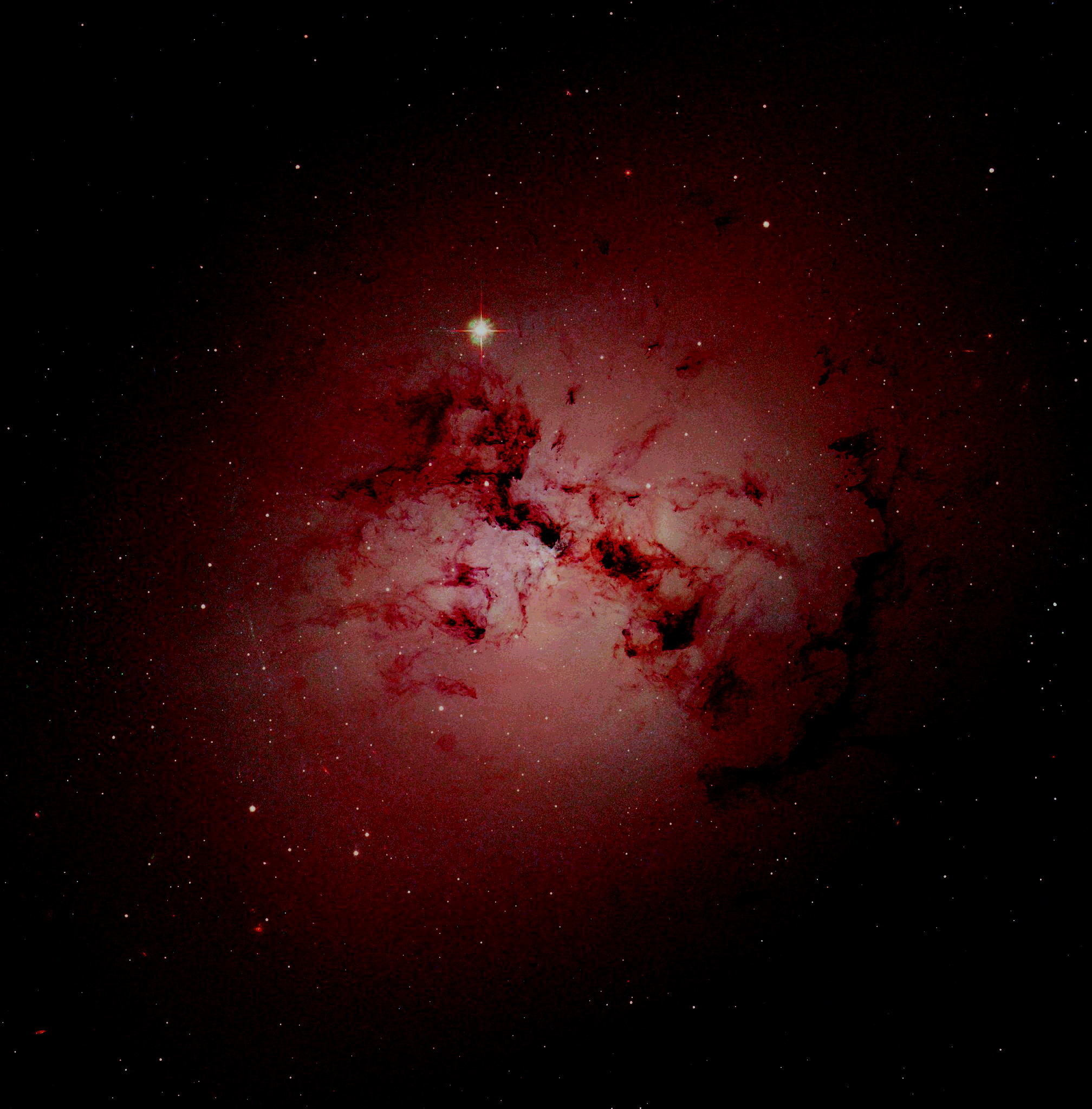
The big question, then, is which events are more common and which ones are rarer, and what timescales each process dominates on.
The lowest-mass stars that are still heavy enough to ignite nuclear fusion in their cores will be the longest-lived ones of all. Stars like Proxima Centauri will survive not for billions, but trillions of years, with the least massive stars of all shining for up to ~10 trillion years. When these stars run out of fuel in their cores, they’ll transition into white dwarfs, just as Sun-like stars do. These white dwarfs will radiate their heat away and will eventually cool to become black dwarfs: a process that takes anywhere from 100 trillion to a quadrillion years.
Although the merger of supermassive black holes should occur within only a few billion years, leading to a supermassive black hole that’s many hundreds of millions of solar masses at Milkdromeda’s center, other processes will take much longer. Gravitational interactions between individual objects in the galaxy will be the next important thing, with the outermost objects bound to a star becoming the first to be ejected. By the time most of the presently existing stars have faded away, most stellar systems will have lost their Oort clouds and Kuiper belt, as well as their outer planets. The Solar System, for instance, in a quadrillion years, might consist only of a black dwarf orbited by the remnants of Mars, Jupiter, and Saturn, plus whatever moons remain.
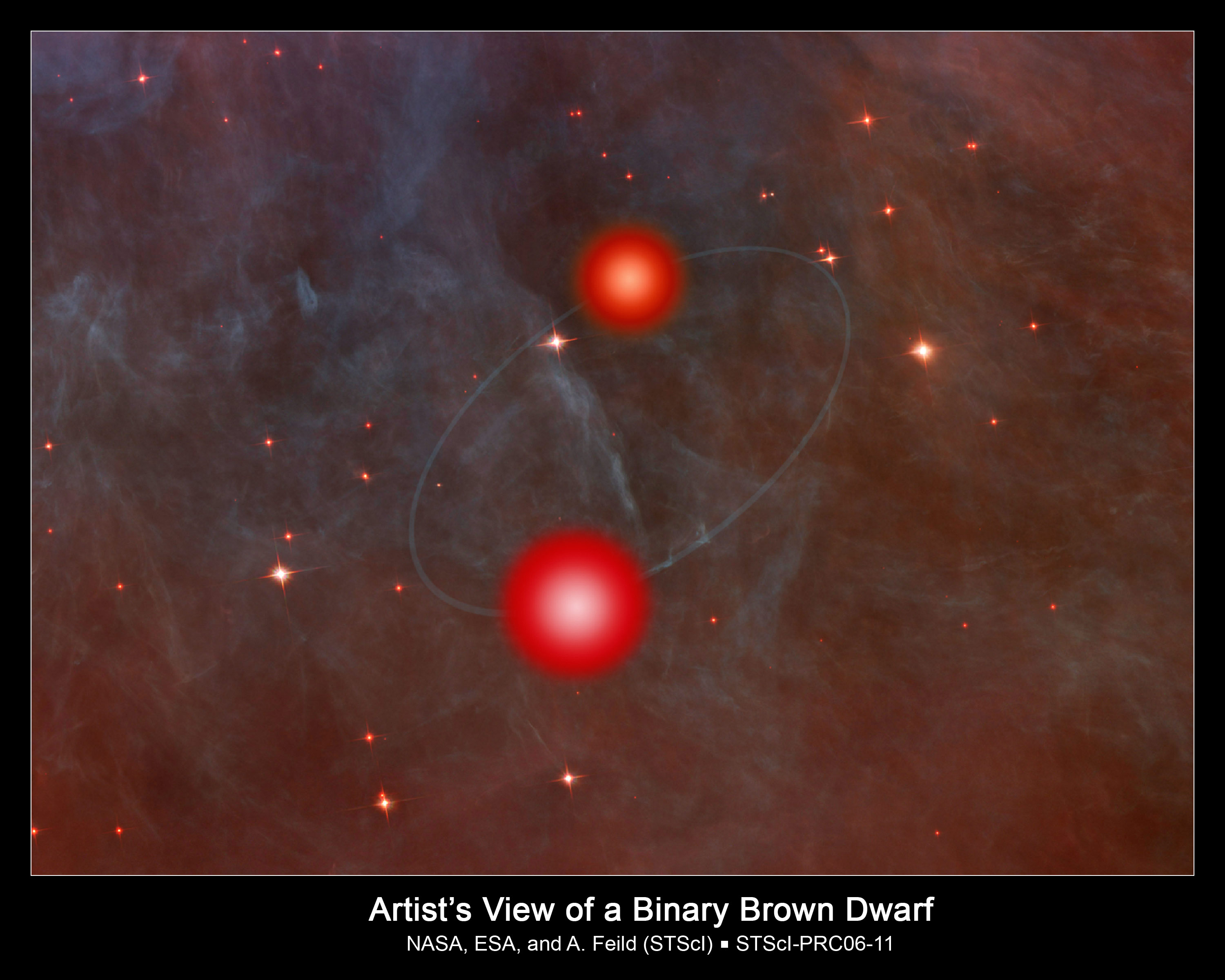
Although it’s tempting to think the Universe will now be “dark” once the last stellar remnants have faded away, there’s another source of light that will come to dominate: the mergers of brown dwarfs and other “failed stars” that are members of multi-star systems. We now have extraordinary confirmation that all masses in orbit around one another will eventually see those orbits decay, leading to inspirals and mergers. When two brown dwarfs whose total mass exceeds about 7.5% the (current) mass of the Sun merge together, they’ll form a full-fledged star, leading to stellar rebirth after all.
In fact, any planet orbiting one of those stars with the right ingredients for life might just get an opportunity to become a living world after all. Even in a mostly cold, dark, empty Universe, life might once again find a way. The timescale for gravitational waves to cause a merger depends entirely on the initial conditions a multi-star system formed with, but many of them will no doubt become stars over the first ~100 quadrillion years or so of the Universe’s history. Just like the prior low-mass stars, they’ll burn through their fuel for trillions of years, and then shine for hundreds of trillions to a quadrillion years more as a white dwarf, before fading away to a black dwarf.
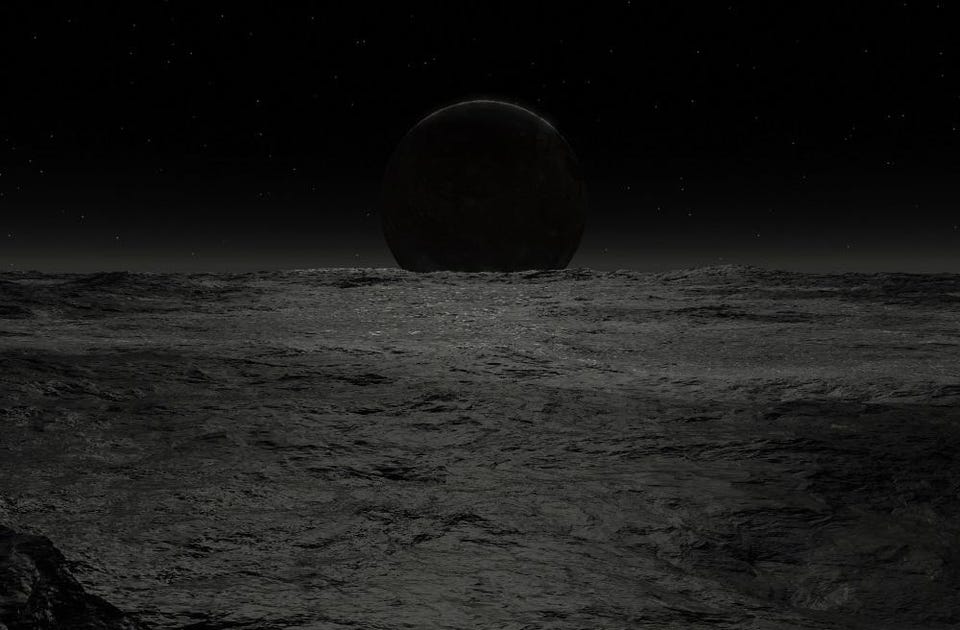
The systems that take longer than that to inspiral and merge, however, will be in grave danger of three other processes:
- gravitational interactions leading to their disruption and unbinding, or even a complete ejection,
- a random collision, leading to an immediate cataclysm,
- or being driven into the galactic center, where they’ll be devoured by the central supermassive black hole.
For any individual system, there’s about a 99% chance, if it doesn’t form a star during the first ~100 quadrillion years of our cosmic history, that it will experience a significant enough gravitational interaction to be ejected. There’s about a 1% chance that a collision between two stellar remnants or a brown dwarf and a stellar remnant will occur, leading to a nova, kilonova, or supernova. And about 1-in-10,000 of these systems will be driven into the galactic center, where a supermassive black hole will eventually devour them. The typical timescale for an ejection is on the order of ~100 quadrillion to 1 quintillion years; the timescale for a random collision is ~10 to 100 quintillion years; the timescale for being devoured by the central black hole is best measured in the sextillions of years or more.

Most of the objects made of normal matter within the galaxy, then, will be ejected, with only a few being devoured by the central, supermassive black hole. Eventually, there will only be a few remnants left in what remains of our galaxy, shrouded by an enormous halo of massive dark matter. Most of the planets and stars that ever formed will be ejected into the abyss of intergalactic space, where they’ll wander through the void for all eternity.
But there will be one additional way that flashes of light will wind up permeating the cosmos: through the evaporation of black holes. A black hole that’s the mass of the Sun will evaporate after 1067 years; the most massive ones, skyrocketing into the tens of billions of solar masses, will evaporate after around 10100 years. This process, although the slowest thing we’ve mentioned, is much faster than the timescales it would take for the non-ejected masses to radiate their orbital energy away through gravitational waves; these supermassive black holes will begin decaying much faster than they grow once a few sextillion years have gone by. Only these last “blips” of black hole evaporation will illuminate the Universe; everything else is cold, dead, and degenerate by now.

There are some open questions, however, that could change the story in subtle ways.
Dark energy may not be a cosmological constant, but could evolve with time, leading to either a Big Crunch or a Big Rip, or something else entirely. We may not be in the true vacuum state of the Universe, and if we experience vacuum decay, so could our fate. Atoms that we presently think are stable could wind up decaying on long enough timescales. And perhaps, although we don’t think it’s the case, it’s plausible that a gravitational interaction in a nearby galaxy group could lead to other members someday joining our Local Group. We can only extrapolate our future based on the limits of what’s known today.
But that said, we sure do know a lot about what to expect in our far, distant cosmic future! There will come an era where all the distant galaxies beyond our own have disappeared from view. After that, we’ll reach a time where the stars have all gone out, and where all the stellar remnants have faded away. Although the darkness will be punctuated by flashes of light, eventually, the stellar remnants in our galaxy will all be ejected, and the last remaining vestige of matter — our central, supermassive black hole — will decay away. The timescales are tremendous, but still finite; it’s a solemn reminder to enjoy the Universe in all its glory while it’s still here. In this Universe, it truly appears that nothing lasts forever.
Send in your Ask Ethan questions to startswithabang at gmail dot com!