How Do The Most Massive Stars Die: Supernova, Hypernova, Or Direct Collapse?
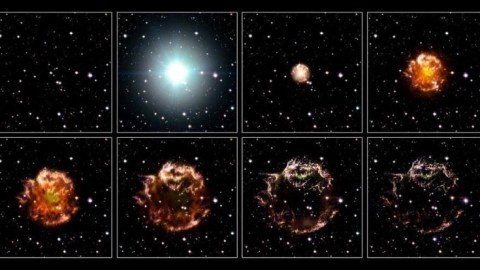
We’re taught that the most massive stars in the Universe all die in supernovae. We were taught wrong.
Create a star that’s massive enough, and it won’t go out with a whimper like our Sun will, burning smoothly for billions upon billions of year before contracting down into a white dwarf. Instead, its core will collapse, leading to a runaway fusion reaction that blows the outer portions of the star apart in a supernova explosion, all while the interior collapses down to either a neutron star or a black hole. At least, that’s the conventional wisdom. But if your star is massive enough, you might not get a supernova at all. Another possibility is direct collapse, where the entire star just goes away, and forms a black hole. Still another is known as a hypernova, which is far more energetic and luminous than a supernova, and leaves no core remnant behind at all. How will the most massive stars of all end their lives? Here’s what the science has to say so far.
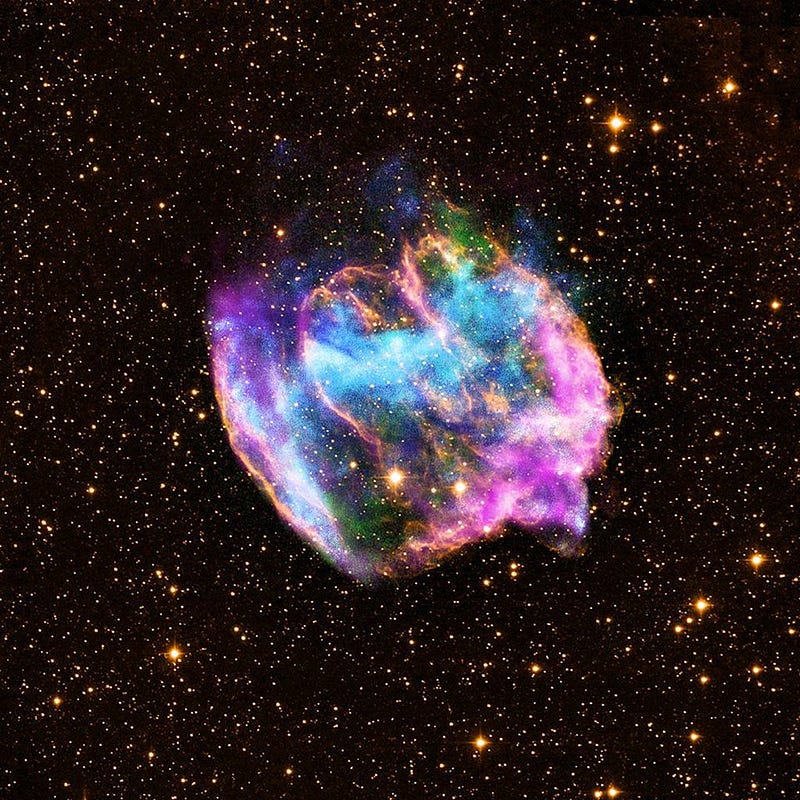
Every star, when it’s first born, fuses hydrogen into helium in its core. Sun-like stars, red dwarfs that are only a few times larger than Jupiter, and supermassive stars that are tens or hundreds of times as massive as ours all undergo this first-stage nuclear reaction. The more massive a star is, the hotter its core temperature reaches, and the faster it burns through its nuclear fuel. As a star’s core runs out of hydrogen to fuse, it contracts and heats up, where — if it gets hot and dense enough — it can begin fusing even heavier elements. Sun-like stars will get hot enough, once hydrogen burning completes, to fuse helium into carbon, but that’s the end-of-the-line in the Sun. You need a star about eight (or more) times as massive as our Sun is to move onto the next stage: carbon fusion.
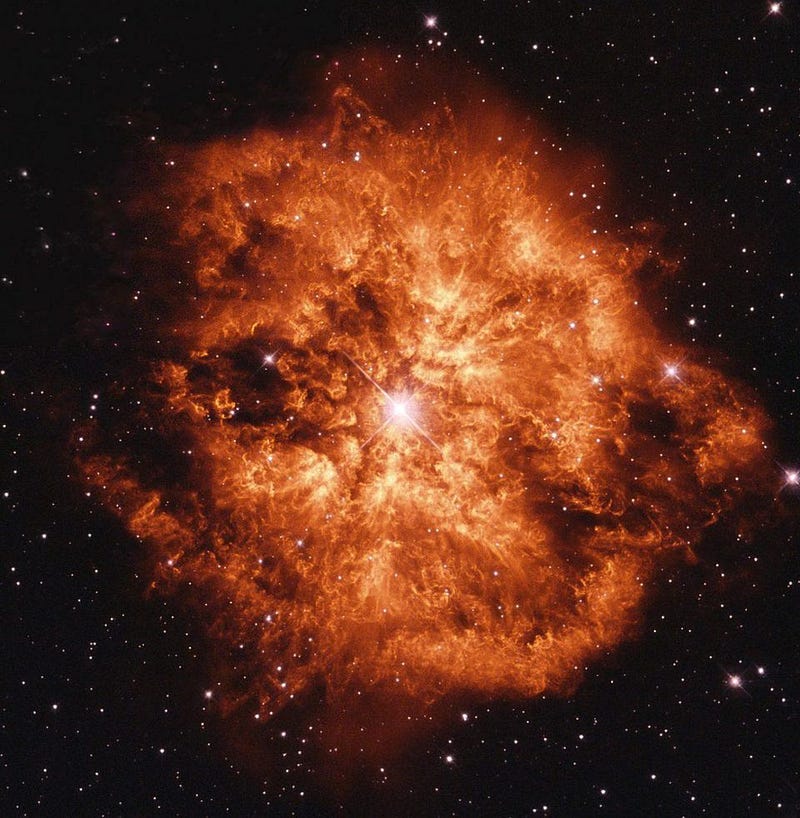
If your star is that massive, though, you’re destined for some real cosmic fireworks. Unlike the Sun-like stars that gently blow off their outer layers in a planetary nebula and contract down to a (carbon-and-oxygen-rich) white dwarf, or the red dwarfs that never reach helium-burning and simply contract down to a (helium-based) white dwarf, the most massive stars are destined for a cataclysmic event. Most often, especially towards the lower-mass end (~20 solar masses and under) of the spectrum, the core temperature continues to rise as fusion moves onto heavier elements: from carbon to oxygen and/or neon-burning, and then up the periodic table to magnesium, silicon, and sulfur burning, which culminates in a core of iron, cobalt and nickel. Since fusing these elements would cost more energy than you gain, this is where the core implodes, and where you get a core-collapse supernova from.
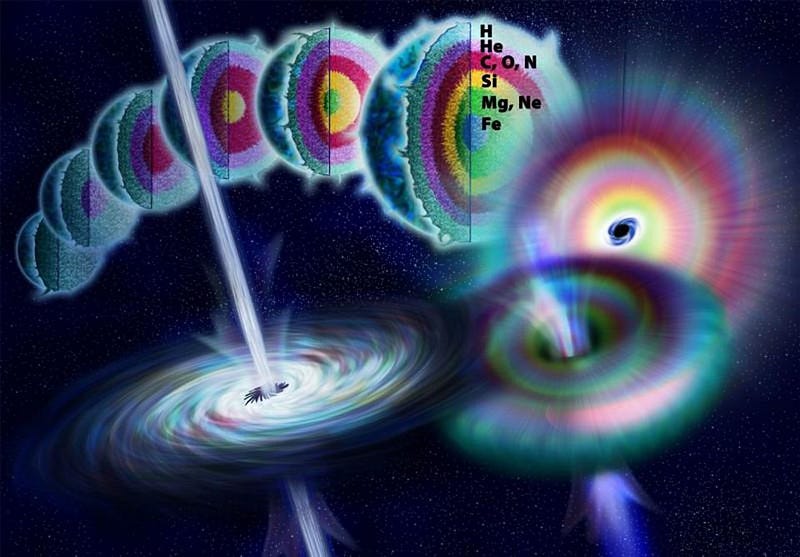
It’s a brilliant, spectacular end for many of the massive stars in our Universe. Of all the stars that are created in this Universe, less than 1% are massive enough to achieve this fate. As you go to higher and higher masses, it becomes rarer and rarer to have a star that big. Somewhere around 80% of the stars in the Universe are red dwarf stars: only 40% the Sun’s mass or less. The Sun itself is more massive than about 95% of stars in the Universe. The night sky is full of exceptionally bright stars: the easiest for the human eye to see. Beyond the lower limit for supernovae, though, there are stars that are many dozens or even hundreds of times the mass of our Sun. They’re rare, but cosmically, they’re extremely important. The reason is that supernovae aren’t the only way these massive stars can live-or-die.
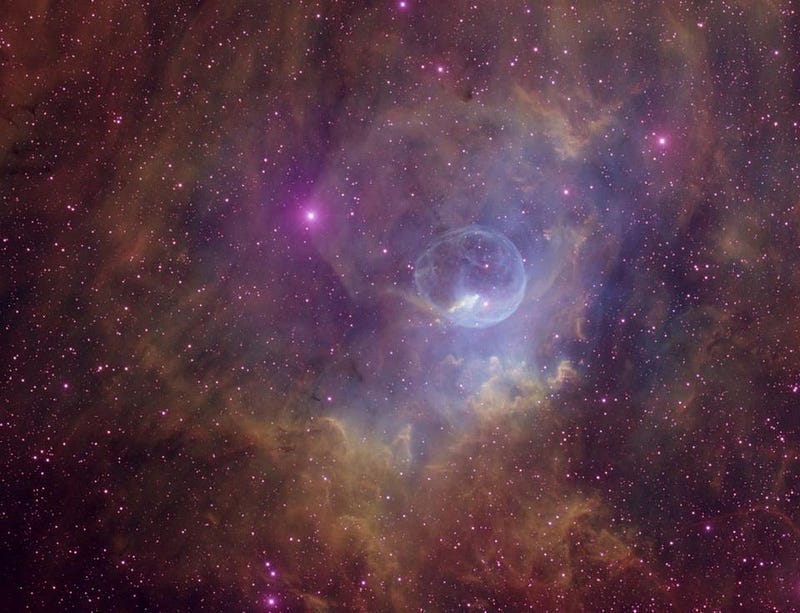
First off, many massive stars have outflows and ejecta. Over time, as they get close to either the end of their lives or the end of a particular stage of fusion, something causes the core to briefly contract, which in turn causes it to heat up. When the core becomes hotter, the rate of all types of nuclear fusion increase, which leads to a rapid increase in the energy created in a star’s core. This energy increase can blow off large amounts of mass, creating an event known as a supernova impostor: brighter than any normal star, causing up to tens of solar masses worth of material to be lost. The star Eta Carinae (below) became a supernova impostor in the 19th century, but within the nebula it created, it still burn away, awaiting its ultimate fate.
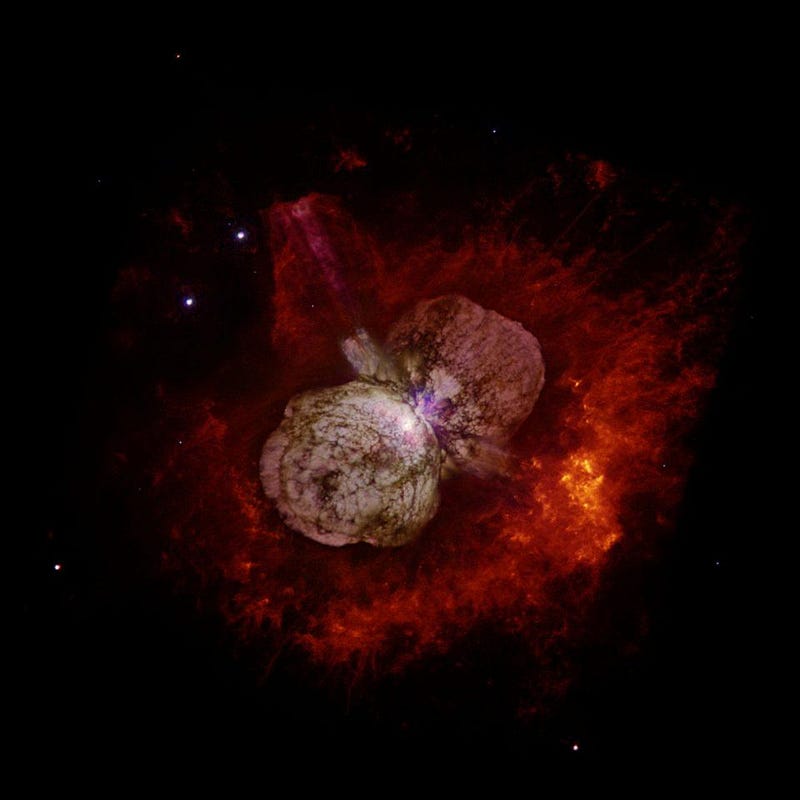
So what will the ultimate fate of a star more massive than 20 times our Sun be? Well, there are three possibilities, and we aren’t entirely sure what the conditions are that can drive each one. One is a supernova, which we’ve already discussed. Any ultra-massive star that loses enough of the “stuff” that makes it up can easily go supernova if the overall star structure suddenly falls into the right mass range. But there are two other mass ranges — and again, we’re uncertain what the exact numbers are — that allow for two other outcomes. Both of them must exist; they’ve already been observed.
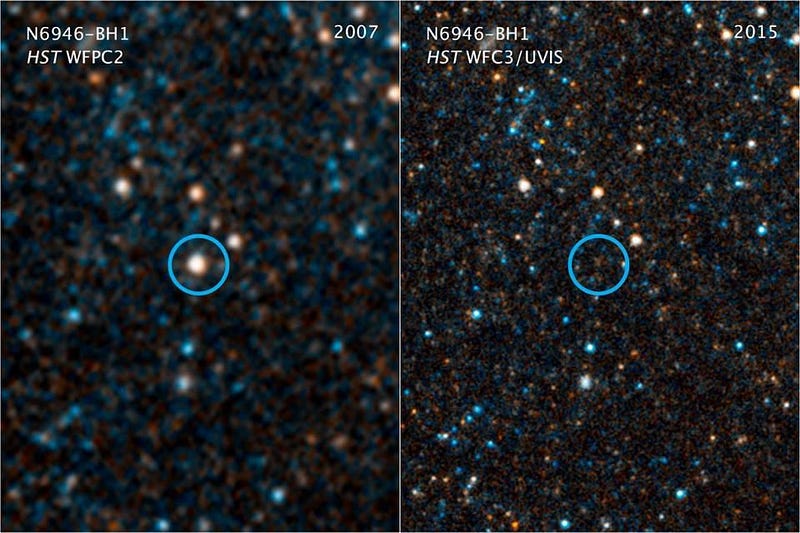
Direct collapse black holes. When a star goes supernova, its core implodes, and can either become a neutron star or a black hole, depending on mass. But just last year, for the first time, astronomers observed a 25 solar mass star just disappear. Stars don’t simply go away without a sign, but there’s a physical explanation for what could’ve happened: the core of the star stopped producing enough outward radiation pressure to balance the inward pull of gravity. If the central region gets dense enough, in other words, if enough mass gets compacted inside a small enough volume, you’ll form an event horizon and create a black hole. And if you make a black hole, everything else can get pulled in.
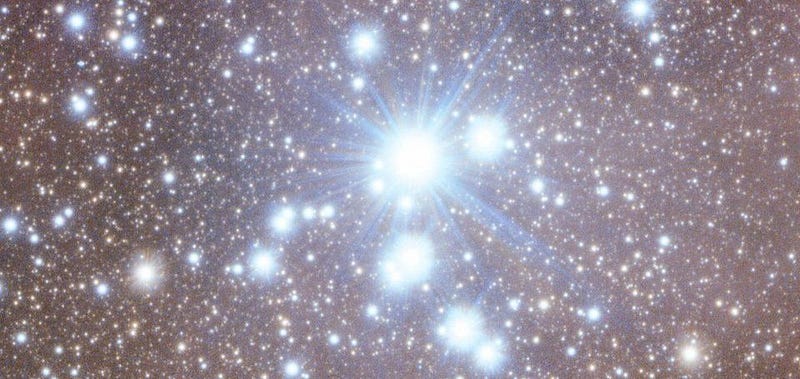
Direct collapse was theorized to happen for very massive stars, beyond perhaps 200–250 solar masses. But the recent disappearance of such a low-mass star has thrown all of that into question. Perhaps we don’t understand the interiors of stellar cores as well as we thing, and perhaps there are multiple ways for a star to simply implode entirely and wink out of existence, without throwing off any appreciable amount of matter. If this is the case, forming black holes via direct collapse may be far more common than we thing, and may be a very neat way for the Universe to build up its supermassive black holes from extremely early times. But there’s another outcome that goes in the entirely opposite direction: putting on a light show far more spectacular than a supernova can offer.
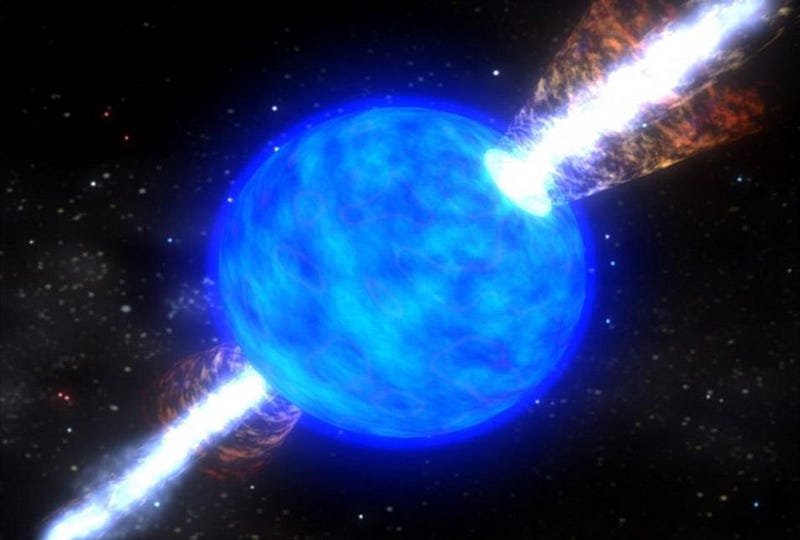
Hypernova explosions. Also known as a superluminous supernova, these events are far brighter and display very different light curves (the pattern of brightening and fading away) than any other supernova. The leading explanation behind them is known as the pair-instability mechanism. When you collapse a large mass — something hundreds of thousands to many millions of times the mass of our entire planet — into a small volume, it gives off a tremendous amount of energy. In theory, if we made a star massive enough, like over 100 times as massive as the Sun, the energy it gave off would be so great that the individual photons could split into pairs of electrons and positrons. Electrons you know, but positrons are the anti-matter counterparts of electrons, and they’re very special.
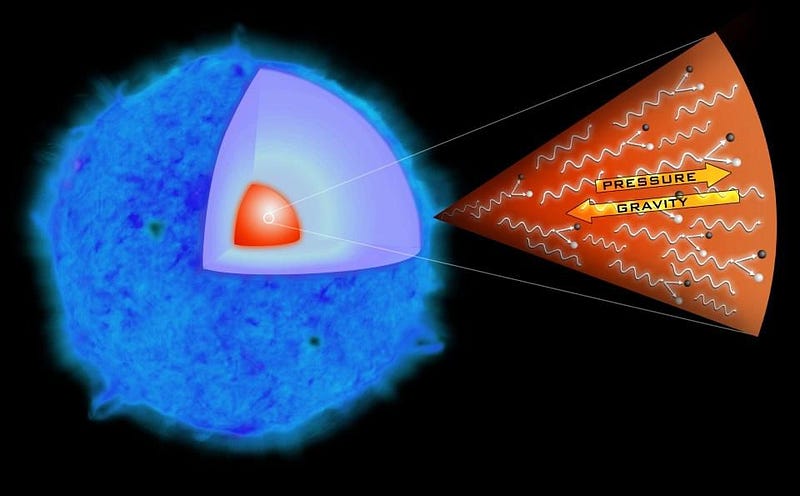
When positrons exist in great abundance, they’ll inevitably collide with any electrons present. This collision results in the annihilation of both, producing two gamma-ray photons of a very specific, high energy. If the rate of positron (and hence, gamma-ray) production is low enough, the core of the star remains stable. But if the rate of gamma-ray production is fast enough, all of these excess 511 keV photons will heat up the core. In other words, if you start producing these electron-positron pairs at a certain rate, but your core is collapsing, you’ll start producing them faster and faster… continuing to heat up the core! And you can’t do this indefinitely; it eventually causes the most spectacular supernova explosion of all: a pair instability supernova, where the entire, 100+ Solar Mass star is blown apart!
This means there are four possible outcomes that can come about from a supermassive star:
- a neutron star and the gas from a supernova remnant, from a low-mass supernova,
- a black hole and the gas from a supernova remnant, from a higher-mass supernova,
- a very massive black hole with no remnant, from the direct collapse of a massive star,
- or the gas from a remnant alone, from a hypernova explosion.
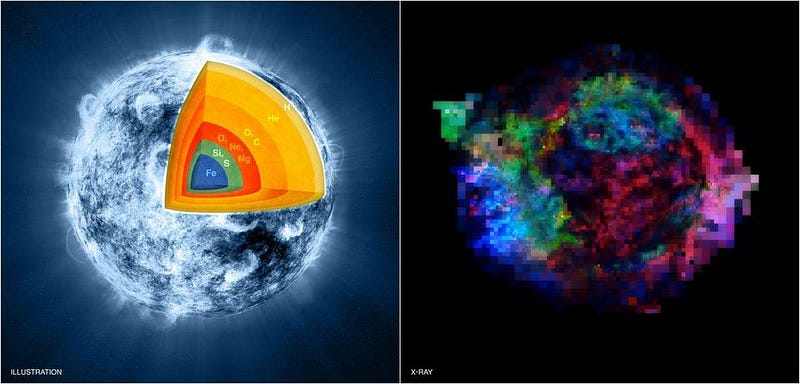
When we see a very massive star, it’s tempting to assume it will go supernova, and a black hole or neutron star will remain. But in reality, there are two other possible outcomes that have been observed, and happen quite often on a cosmic scale. Scientists are still working to understand when each of these events occurs and under what conditions, but they all happen. The next time you look at a star that’s many times the size and mass of our Sun, don’t think “supernova” as a foregone conclusion. There’s a lot of life left in these objects, and a lot of possibilities for their demise, too. We know our observable Universe started with a bang. For the most massive stars, we still aren’t certain whether they end with the ultimate bang, destroying themselves entirely, or the ultimate whimper, collapsing entirely into a gravitational abyss of nothingness.
Ethan Siegel is the author of Beyond the Galaxy and Treknology. You can pre-order his third book, currently in development: the Encyclopaedia Cosmologica.