Has LIGO Just Detected The ‘Trifecta’ Signal That All Astronomers Have Been Hoping For?
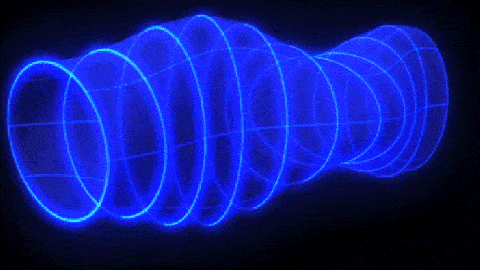
The dream of multi-messenger astronomy is to seen an event with gravitational waves, neutrinos, and light all together. The newest candidate just might get us there.
When it comes to cataclysmic events in the Universe — wherever large-magnitude astrophysical interactions cause an enormous release of energy — our understanding of the laws of physics tells us that there are three possible ways to detect and measure them. The first is the most familiar: through light, or electromagnetic waves. The second is through the arrival of particles: like cosmic rays or energetic neutrinos. And the third, which first came to fruition just under four years ago, is from the detection of gravitational waves.
Since gravitational wave detection first occurred, astronomers have been hoping for the ultimate event: a signal that would be identifiable and detectable via all three methods. It’s never been observed before, but ever since LIGO started its latest data-taking run in April, it’s been the not-so-secret hope of astronomers of all types. With a new candidate event observed on Sunday, July 28, 2019, we might have just hit the jackpot.
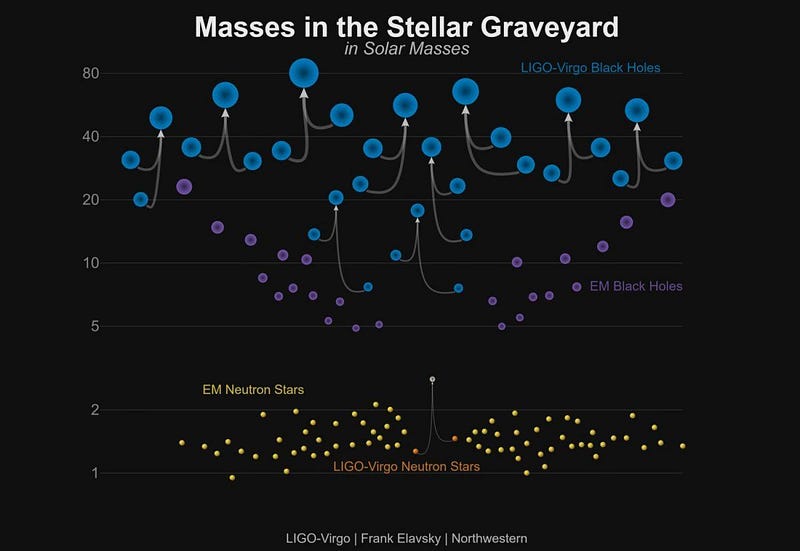
LIGO was operational and taking data over two different periods from 2015 through 2017, with runs of 4 and 9 months in duration, respecively. The latter included an overlap, during the summer of 2017, with the operation of the VIRGO detector. Over that timespan, those gravitational wave detectors saw a grand total of 11 events that have now been classified as robust gravitational wave detections.
10 of them were from black hole-black hole mergers, where the masses of those merging black holes ranged from a low of 8 solar masses to a high of 50 solar masses, albeit with large uncertainties. When black holes merge, they aren’t expected to have an electromagnetic counterpart. Only one of those events — the very first one — had any light-based signal detected that was possibly associated with it, and even that was only by one detector (NASA’s Fermi) and at a modest (2.9-sigma) significance.
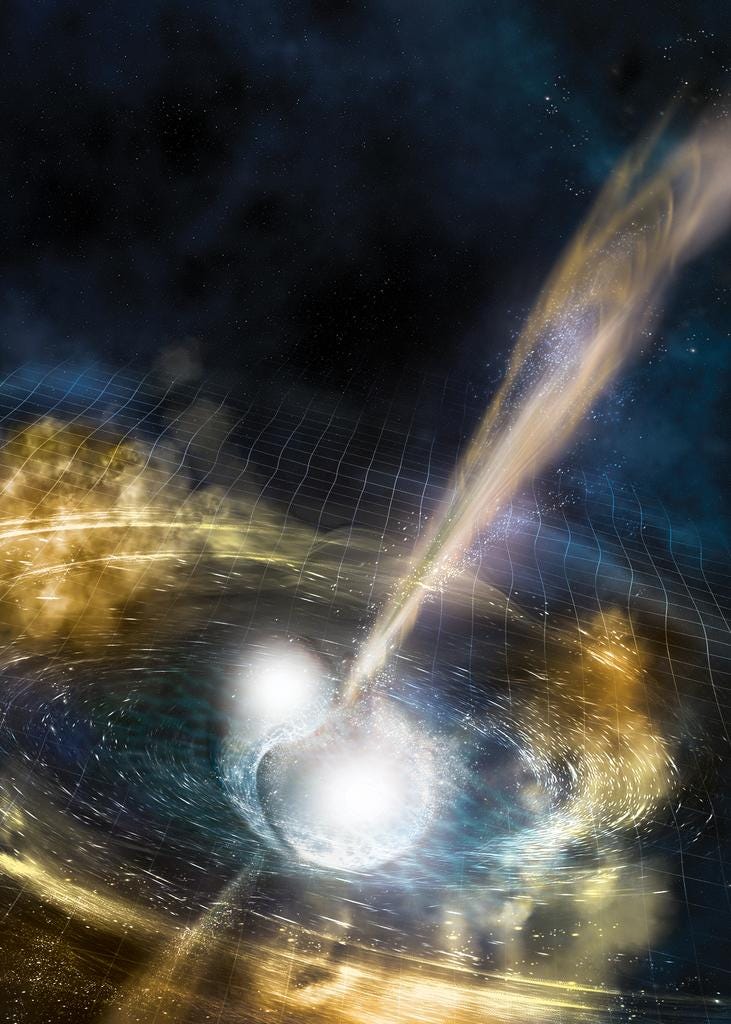
But one signal was fundamentally different. Instead of a black hole-black hole merger, it had the right frequency and amplitude properties to indicate a different type of event: a neutron star-neutron star merger. Whereas black holes have event horizons around the overwhelming majority of their masses, shielding the outside Universe from any particles or electromagnetic radiation that would be created from the cataclysmic event, neutron stars do not.
As a result, a gamma-ray signal arrived nearly at the same exact time as the gravitational waves, with less than a 2-second difference in arrival time. Across a journey of more than 100 million light-years, that one measurement both confirmed that gravitational waves and electromagnetic waves travel at the same speed to within 15 significant digits, and also heralded the first multi-messenger signal that involved gravitational waves.
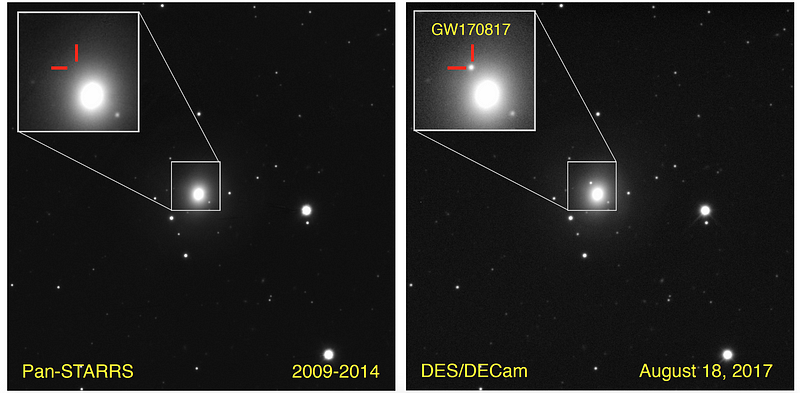
Over the coming weeks, dozens of other professional observatories got in on the action. X-rays, optical signals, infrared and radio observations allowed astronomers to better study this kilonova event, and helped astronomers across fields understand how their data and information would be complementary to one another in the case of such an event.
While we can learn a tremendous amount of astrophysical information about these objects and events from each electromagnetic wavelength, the information we learn from gravitational waves is different. Even with just this one multi-messenger event, gravitational waves alone taught us:
- the rough location of this event,
- the masses of the neutron stars prior to the merger,
- the final mass of the final-state object,
- and that the post-merger object was a rapidly-rotating neutron star for a substantial fraction of a second before finally collapsing into a black hole.
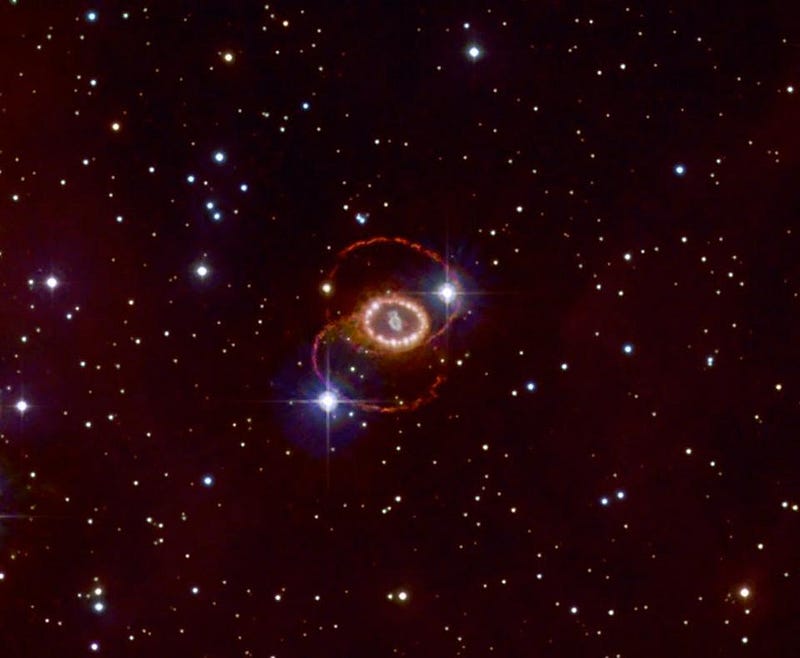
This marked the first time that gravitational waves were used as a component of multi-messenger astronomy, but it wasn’t the only multi-messenger event ever observed. Back in 1987, a supernova went off in the Large Magellanic Cloud, which is cosmically in our own backyard at just 165,000 light-years distant. It marked the closest supernova to occur, in proximity to Earth, in the modern era of physics and astronomy.
While the light arrived at our telescopes and detectors, it was a remarkable boon for astronomy, as this enabled us to study a supernova up close in a way that hadn’t been possible since the invention of the telescope. But supernovae are accompanied by runaway nuclear fusion reactions, and those generate tremendous numbers of neutrinos. With large, fluid-filled tanks lined with photomultiplier tubes, we were able to detect a slew of neutrinos at the same time.
This marked the true dawn of multi-messenger astronomy, and with it, we learned a tremendous amount of information about the phenomenon we were observing. The neutrinos all carried specific amounts of energy and arrived over a timespan of multiple seconds. This allowed us to understand the internal mechanisms of nuclear reactions occurring in a core-collapse supernova: information we never could have received from electromagnetic signals alone.
Many scientists are hopeful that were a similar supernova to go off today, our scientific instruments would enable us to detect tens of thousands of neutrinos — and, if nature is kind, gravitational waves as well — in addition to the light signals. That would realize the ultimate dream of the relatively new field of multi-messenger astronomy: to measure three fundamentally different types of signals associated with the same event.
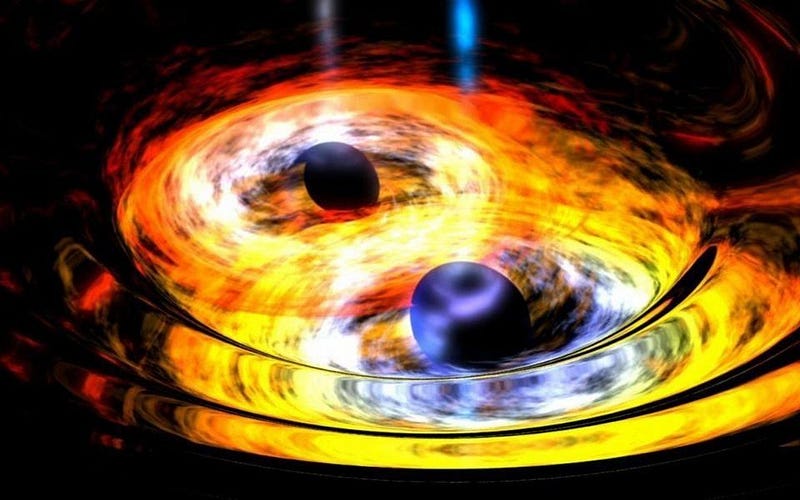
Well, it’s still extremely early, but this dream may be realized with an event that occurred on July 28, 2019. You might be surprised to learn that LIGO turned on again, after a substantial upgrade that increased its sensitivity and detection range, back in April of 2019. It’s been operating for nearly four full months, taking data for practically all of it.
And even though you haven’t heard anything from the collaboration in that time, they have a publicly available database of everything that they consider to be candidate events. At the time that this piece is being written, 24 have been recorded: more than twice the total number of events seen during the prior two runs combined. The latest, presently labeled S190728q, may turn out to be the first triple multi-messenger astronomy event ever.
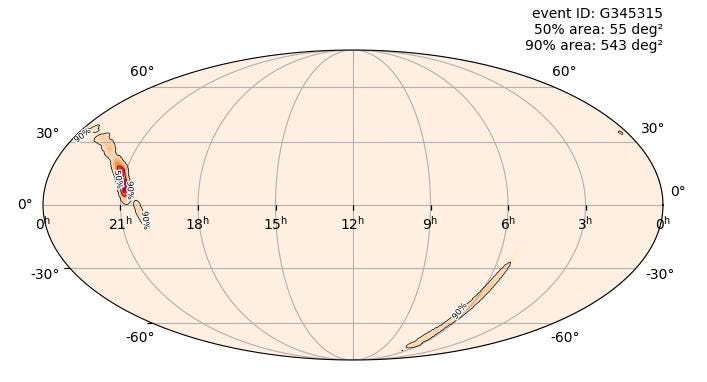
From the gravitational waves alone, scientists were able to perform a fast analysis and restrict the location where the originating event may have occurred to just 55 square degrees (out of ~40,000 on the entire sky) as the best place to look for other types of messenger signals.
Completely independently, the IceCube neutrino detector at the South Pole detected a track-like neutrino event that corresponds to almost the exact same time of origin. Because of how rare neutrinos are, every event at IceCube is of potential interest as a signal from the distant Universe. This one, in particular, has astronomers across the globe holding their breath.
We can reconstruct its location on the sky, and find that, remarkably, the neutrino overlaps in both space and time with the preliminary gravitational wave signal seen by LIGO and Virgo!
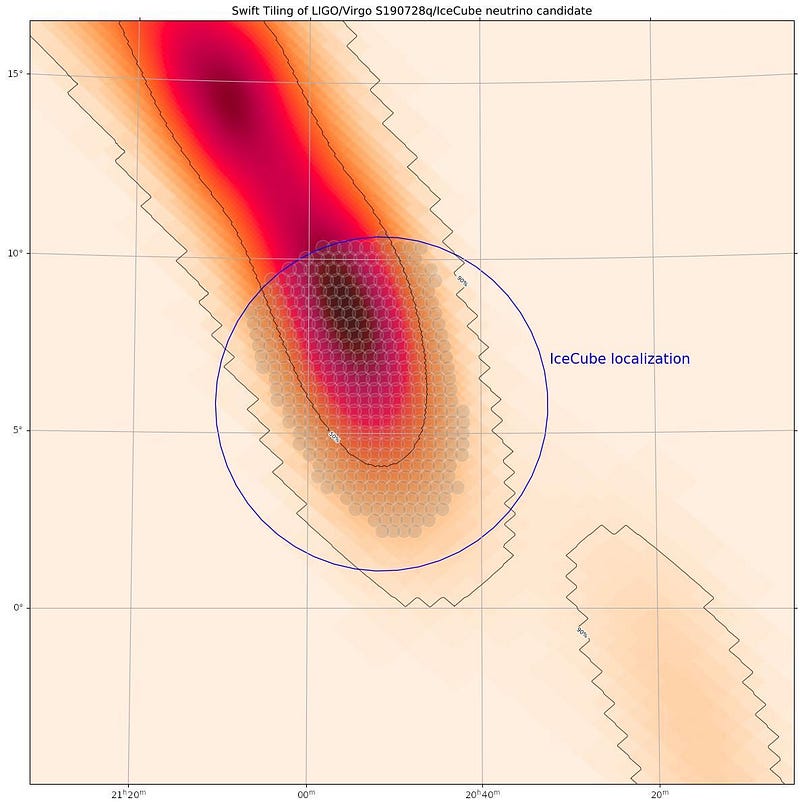
Right now, LIGO is stating, with 95% confidence, that this was most probably a binary black hole merger occurring an estimated 2.87 billion light-years away. If there does turn out to be an electromagnetic counterpart, it would be revolutionary. All at once, we would:
- have our first three-messenger astronomy event,
- learn that either this object wasn’t a binary black hole or that binary black holes could produce electromagnetic counterparts, and
- have a clue as to what types of events could produce detectable gravitational waves, light signals, and neutrinos from such a large distance away.
Even if no electromagnetic signal is seen, but the IceCube and LIGO/Virgo signals do turn out to be real, robust, and aligned, it would be a tremendous achievement. This would mark the first multi-messenger event to involve both gravitational waves and particles.
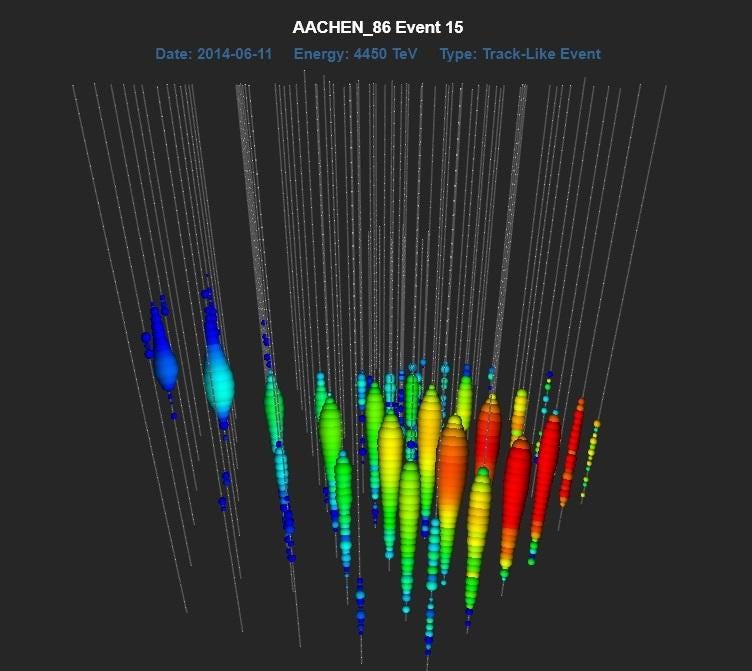
Of course, all of this is just preliminary at this point. The LIGO collaboration has yet to announce a definitive detection of any type, and the IceCube event may turn out to be either a foreground, unrelated neutrino or a spurious event entirely. No electromagnetic signal has been announced, and there might not be one at all. Science moves slowly and carefully, as it should, and all of what’s been written here is a best-case scenario for the optimistic hopefuls out there, not a slam-dunk by any means.
But if we keep watching the sky in these three fundamentally different ways, and keep increasing and improving the precision at which we do so, it’s only a matter of time before the right natural event gives us the signal every astronomer has been waiting for. Just a generation ago, multi-messenger astronomy was nothing but a dream. Today, it’s not just the future of astronomy, but the present as well. There’s no moment in science quite as exciting as being on the cusp of an unprecedented breakthrough.
Ethan Siegel is the author of Beyond the Galaxy and Treknology. You can pre-order his third book, currently in development: the Encyclopaedia Cosmologica.