Yes, removing groundwater is changing Earth’s tilt
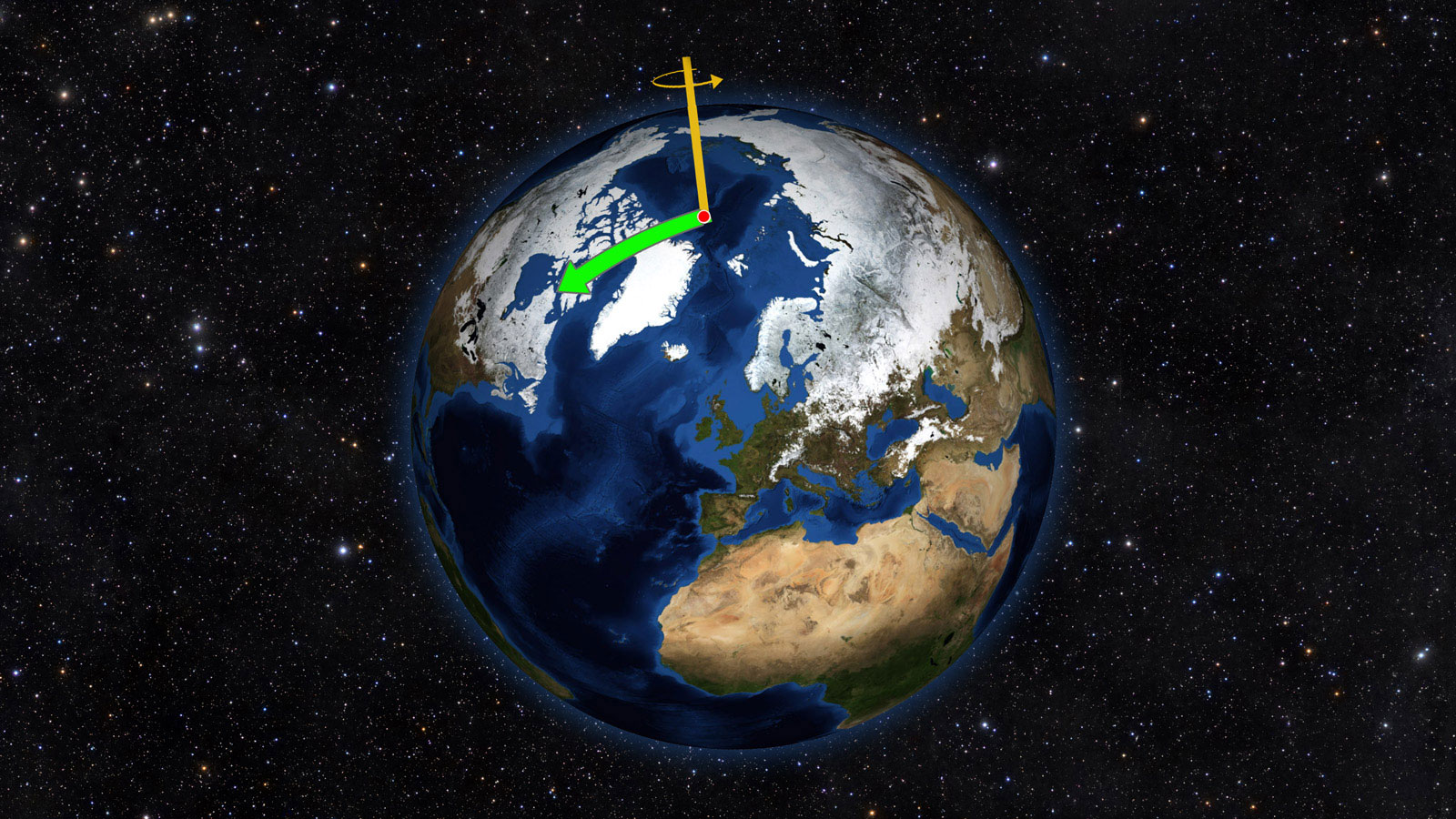
- Under normal circumstances, with no human intervention, Earth's water cycles between the oceans, atmosphere, and land masses, leading to a natural "wobble" for our planet's axis.
- However, human pumping of groundwater, which has been particularly depleted across western North America and northwest India through the 1990s and 2000s, has gradually caused our axial tilt to shift.
- The interplay between Earth's internal mass distribution and the effects we experience on the surface are playing a crucial role for life on planet Earth. Here's how to make sense of it.
Many of us wonder how, as tiny, individual human beings, our actions — even with all 8+ billion of us, put together — could possibly affect something as enormous as the Earth. When we water our lawns and gardens, we don’t generally think about that having a global impact. Even when we consider the copious amounts of water used for crop (and, in many places, golf course) irrigation, it’s difficult to imagine that even the cumulative human uses of water, which covers around 70% of Earth’s surface, could possibly affect the planet as a whole.
And yet, a new study has just linked human-caused groundwater depletion, particularly driven by water-intensive human activities (such as irrigation) in western North America and northwestern India, with a recently observed migration of Earth’s axial tilt that’s happened over the same time period. The study itself goes further, and argues that by linking groundwater depletion to the steady and substantial migration of Earth’s tilt, observed to be moving at 4.36 centimeters-per-year, that same amount of groundwater depletion over the years 1993-2010 has caused 0.62 centimeters of global sea level rise, or the equivalent of 0.37 millimeters-per-year. It’s yet another way that human activity is changing the entire planet, for better and for worse, on alarmingly fast timescales.

When it comes to the planet Earth, we normally think of it as incredibly stable. Sure, we spin on our axis as we revolve around the Sun, and have to deal with the gravitational influence of the Moon and the other planets on top of the primary effects of the Sun’s gravity and Earth’s intrinsic angular momentum: of both the spin and orbital varieties.
Over time, the Moon’s tidal effects on the Earth — meaning that the Moon gravitationally pulls with a greater amount of force on the “near side” of the Earth than the “far side” of the Earth — lead to a few long-term changes.
- The Earth’s rotation steadily slows, lengthening the duration of the day over time.
- The Moon slowly spirals outward, getting more and more distant from Earth, causing more solar eclipses to be annular and fewer to be total as time goes on.
- And the Earth, whose axial tilt would otherwise vary wildly due to the influence of the other planets (just as Mars’s axis varies from about a 10° to a 50° tilt), remains stabilized, varying only between about 22° and 25° over time.
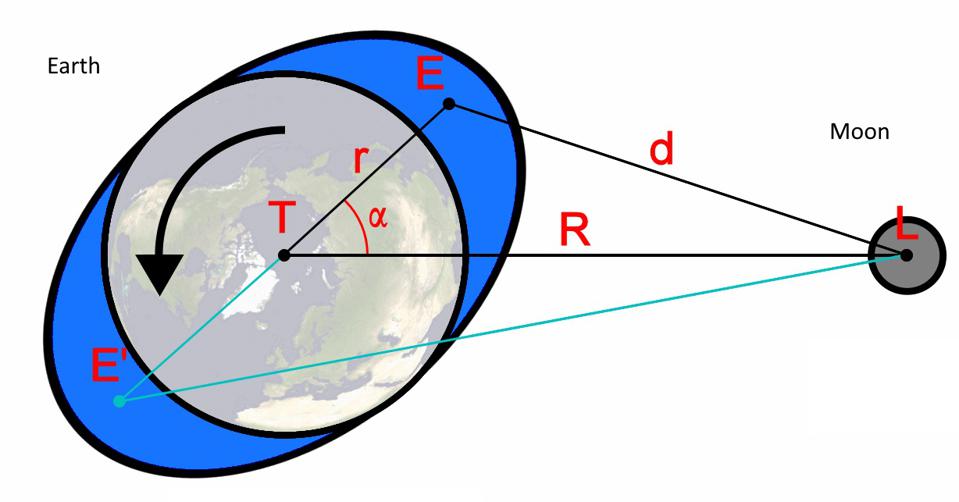
But those changes typically aren’t noticeable on short-term timescales; they require millions of years to add up to something substantial. Even though our axis precesses, or changes the direction in which it points, on timescales of ~26,000 years, the axial tilt normally remains very stable over timescales. The reason is simple and straightforward: angular momentum — or the combination of how an object spins on its axis and revolves around whatever object it orbits — is one of those quantities that’s always conserved under the laws of physics.
But just because something is conserved, or can neither be created nor destroyed, doesn’t mean that we can’t change how it’s distributed among its component parts.
One classic example is to consider a figure skater as she performs the common maneuver of spinning on one skate. With her arms and/or leg extended, she rotates slowly about her axis. However, when she brings her arms and legs closer to the axis of rotation, she speeds up in her rotation. The reason is that angular momentum is a combination of your angular velocity, or how quickly you make a full rotation, and your moment of inertia, or how your mass is distributed: close to or far from the axis of rotation.

In other words, even though angular momentum is always conserved, simply by changing how your internal mass is distributed, you can change your angular velocity, or how quickly you rotate about your axis.
This simple physical law — the conservation of angular momentum — leads to a lot of physical consequences: some of which are small but significant, while others can be severe and even catastrophic, as anyone who’s ever lost their balance while riding a bicycle can attest.
It’s why, when you get your car’s wheels aligned and balanced, they sometimes hammer little metal weights onto the edges where the rims meet the tires: so that the moment of inertia of the wheels-and-tires perfectly aligns with the axis of the rotating axle that supports them. Without that sort of perfect alignment, an off-axis force would get created, which in turn would make a torque, which would put unnecessary pressure on the wheel-and-tire-and-axle system, leading to a faster wearing-out of your equipment. In the most extreme case, driving too fast or for too long with an unbalanced, unaligned wheel like this will simply result in the wheel-and-axle system breaking, leading to the wheel separating from the car.

On Earth, however, the situation is a little bit different. There’s no axle holding the Earth to its axis of rotation, so when we do something that rearranges the mass of the Earth — i.e., that changes the Earth’s moment of inertia — there are two possible responses from the Earth itself.
- The overall moment of inertia could increase (where mass moves farther away from the axis of rotation) or decrease (where mass moves closer to the axis of rotation), which changes the Earth’s angular velocity: decreasing it and causing it to spin slower if the moment of inertia increases, or increasing it and causing it to spin faster if the moment of inertia decreases.
- Alternately, and this is an “and/or” situation, the moment of inertia could simply shift so that the balance of Earth’s mass relative to the axis of its rotation gets rearranged: i.e., masses that were balanced around the Earth’s axis of rotation are now balanced around a slightly different axis of rotation. When this occurs, it causes Earth’s axial tilt to change slightly, shifting toward a more balanced state.
In reality, both of these changes routinely occur. The first typically occurs whenever there’s an Earthquake, as the rearrangement of Earth’s surface-and-interior typically results in more mass being brought closer to the center of the Earth, decreasing the planet’s moment of inertia while causing its angular velocity (or spin rate) to slightly increase.
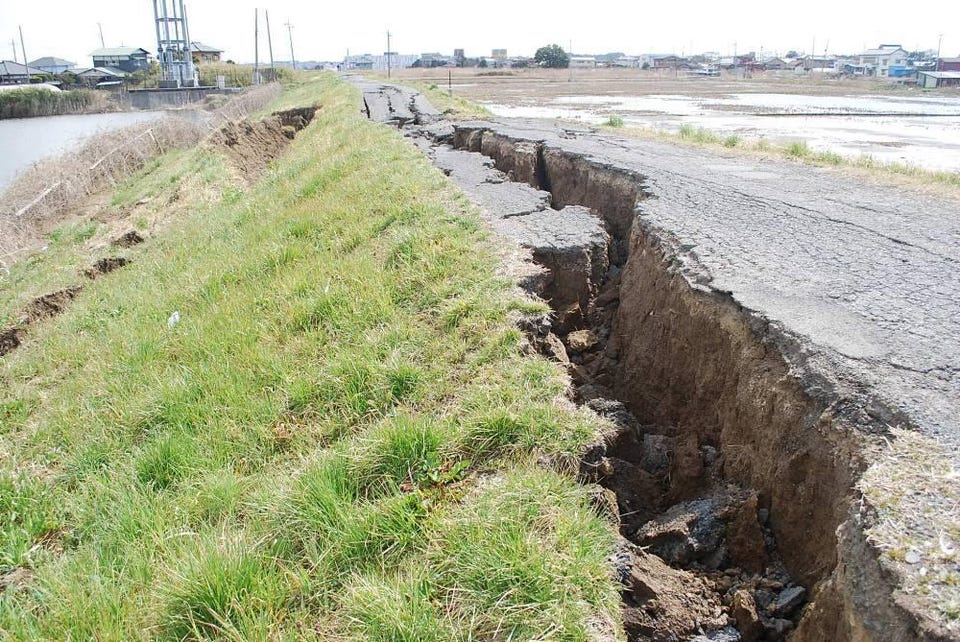
But the water cycle of Earth is the most common cause of the Earth’s rotation axis shifting. When you think about how much mass there is present in the form of water on the Earth, it’s actually a tremendous amount: both in absolute terms and also relative to the entire mass of the Earth. There are approximately 1.35 quintillion (1018) tonnes of water on Earth — primarily in Earth’s oceans but also in seas, lakes, rivers, glaciers, and polar icecaps — adding up to approximately 0.02% of the total mass of our planet.
As icecaps grow and retreat with the seasons, and as water cycles from the oceans to the atmosphere to stores of frozen fresh water on the surface and back again, the orientation of our axial tilt can routinely change by several meters, even over the course of a single year. We’ve known for a long time that the motion of Earth’s water can have this effect, and that’s part of why measuring the gravitational field of Earth over every single point on the surface, to very high precision, is an important scientific endeavor.
Tracking how Earth’s water moves — and understanding how rich vs. depleted groundwater stores are, over time, on a local, regional, and global scale — is a vital endeavor for managing an important but limited natural resource on our planet.
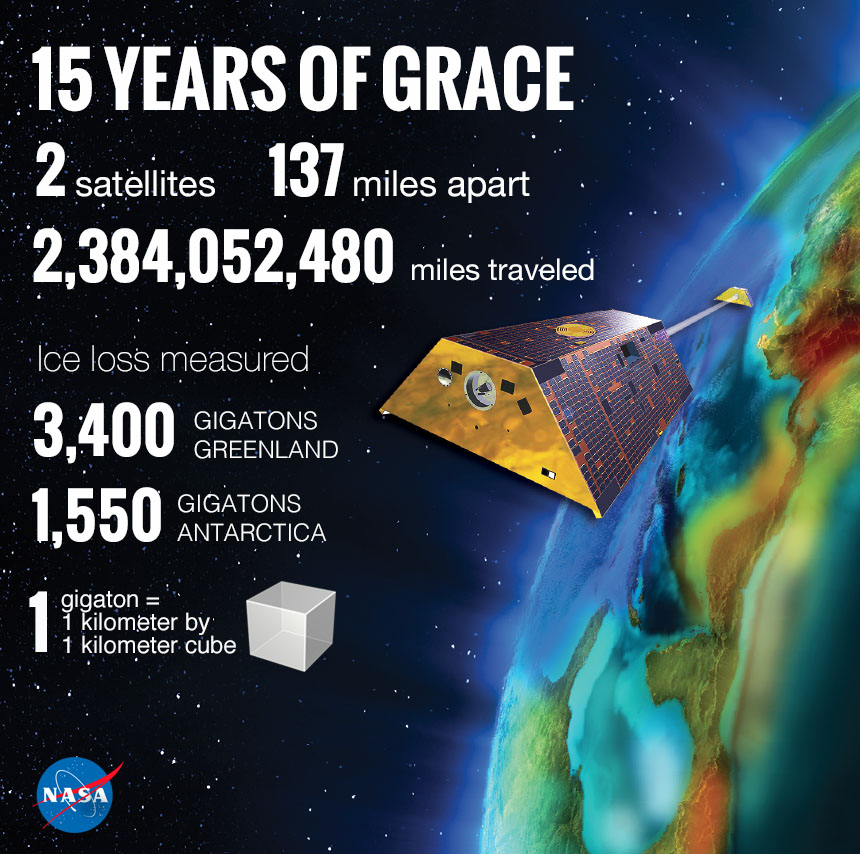
This was one of the main science goals of NASA’s Gravity Recovery and Climate Experiment (GRACE) mission, which operated from 2002 until 2017, before a follow-on mission was launched in 2018. It was able to show, quite surprisingly to many, that the water table in many drought-sensitive regions of the world — including throughout much of southern California — was rapidly decreasing, in some cases by as much as a few centimeters per year, in a sustained, year-after-year fashion.
But understanding precisely how groundwater depletion is working on a global scale, and how it’s rearranging the mass distribution of Earth as a result, is a task that requires even more than precise Earth-monitoring from space. There’s a complex interplay, involving sustained changes-over-time beyond the annual year-to-year variations, between:
- the stores of groundwater throughout the Earth,
- the motion and/or migration of Earth’s rotational axis,
- and the net amount of sea level rise due to water that was previously stored beneath the continents being deposited into Earth’s oceans.
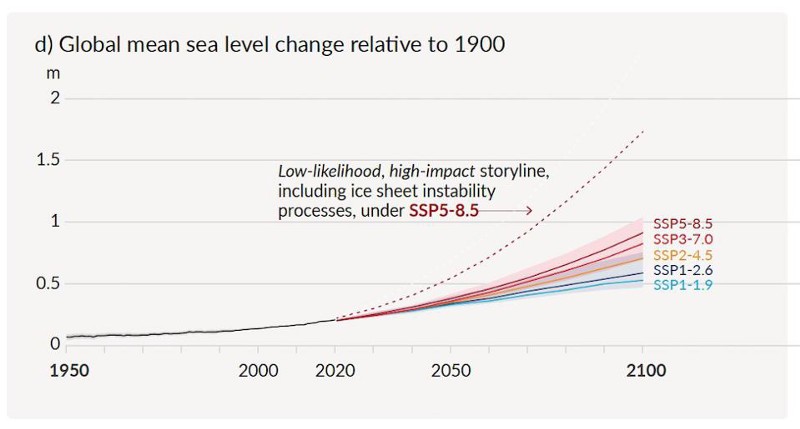
That’s why it should come as a shocking find to everyone that this latest study has determined that, if you neglect the effect of groundwater depletion, the behavior of Earth’s axial tilt and how it’s moved over the time period from 1993-2010 cannot be explained. It can’t be glaciers or glacial melting; it can’t be icecap physics, including seasonal melting and growth; it can’t be due to changes in surface reservoirs of water. All of those factors, when accounted for, still failed to explain what was observed.
Instead, if you take groundwater depletion into account, the scientists found that the location of Earth’s pole — which serves as an excellent proxy for its axial tilt — has been shifting, gradually but in a sustained fashion over that well-studied 17 year time period, at a rate of 4.36 cm/yr, for a cumulative shift of about 74 centimeters (or 29 inches) over that entire duration.
How much groundwater depletion does that correspond to?
A shockingly large number: the equivalent of 2.15 trillion tons of water, or about 1600 parts-per-billion of all the water present on planet Earth.
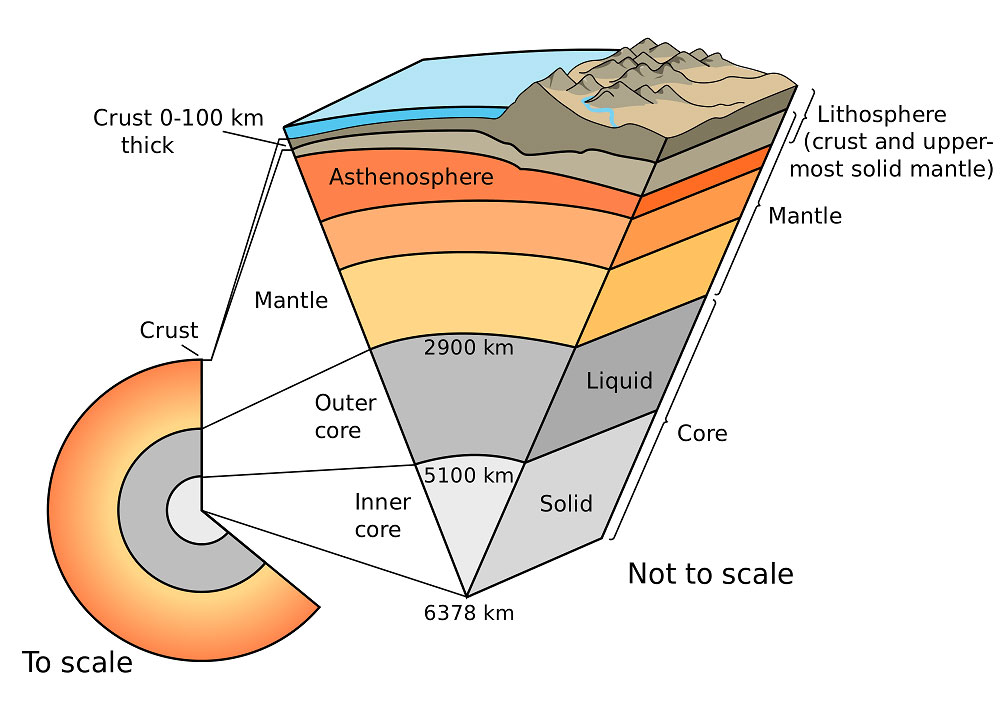
Where does all of that water, that once was in the form of groundwater but was depleted by irrigation, go?
The answer, as you might expect, is “into Earth’s normal water cycle.” The simplest assumption one can make is that the majority of it would go into Earth’s oceans, and that what we can do is then calculate two interesting numbers.
- How much would adding this amount of groundwater into the oceans cause sea levels to rise?
- If we were to redistribute 2.15 trillion tons of groundwater from beneath Earth’s surface, primarily from western North America and northwestern India, evenly into all of the oceans, what would the predicted migration of Earth’s axial tilt have been?
The answer to the first question is a surprisingly significant number: 6.24 millimeters over that 17 year timespan, or about 0.37 millimeters-per-year. This accounts for about 10% of the total current annual sea level rise, with the remaining coming from icecap and glacial melting and the thermal expansion of the oceans as they warm along with the rest of the Earth.
The answer to the second question, however, is what makes this study so compelling: the predicted migration from this simple model would be a change in Earth’s axial tilt of 78.5 cm, in the same direction as was observed, from 1993-2010. That’s an impressive agreement to within just 6% of the observed value, showcasing just how much the distribution of water on Earth’s surface can change the tilt of the Earth itself.
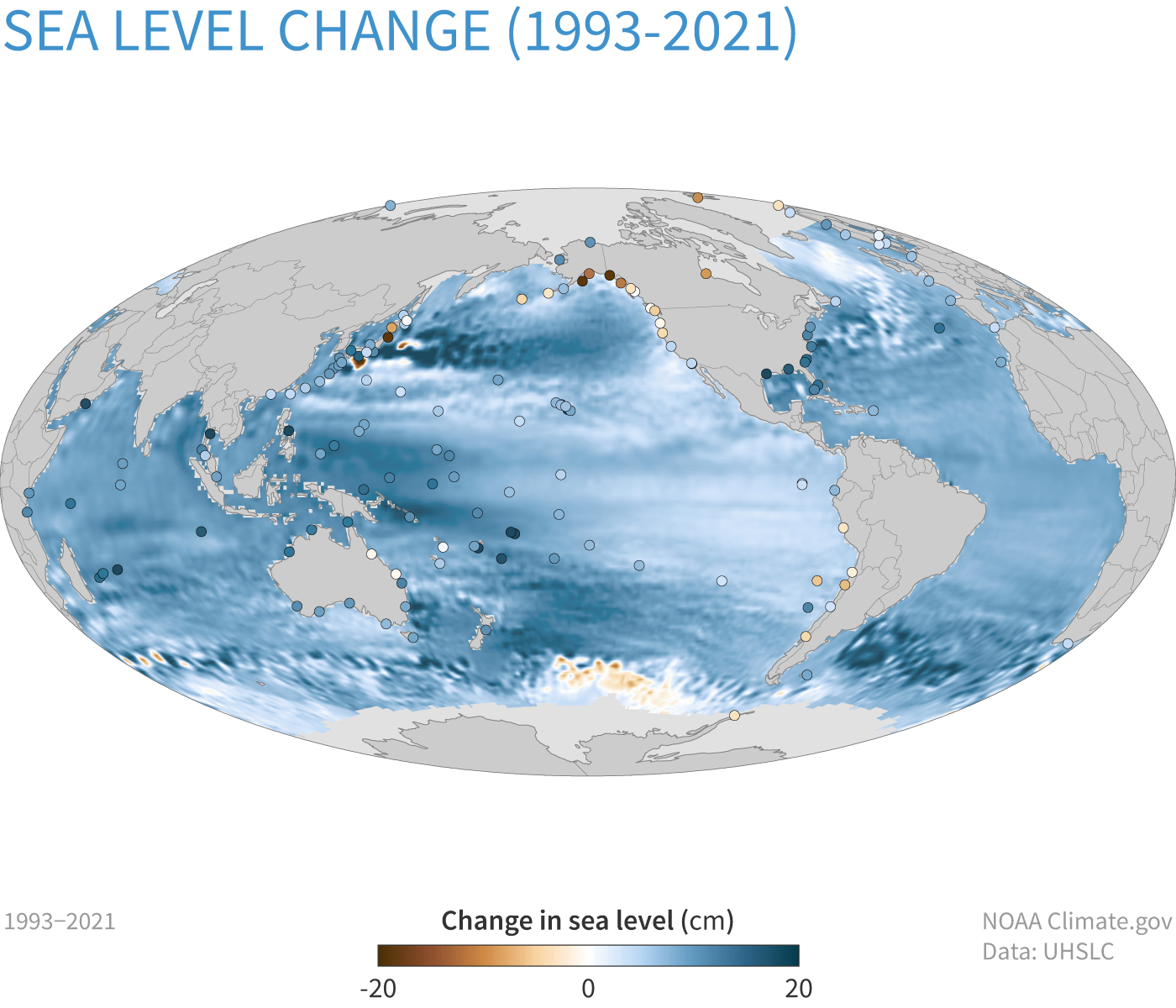
There are a number of lessons one can glean from this study, but the overall, larger lesson is simple. Over even relatively short time periods, the physical changes that we’re making to the Earth are large enough in magnitude that they are having observable, long-term consequences with many downstream effects. As simple and widespread a practice as irrigation, which depletes groundwater unevenly across the Earth, is affecting Earth’s axial tilt and the rate at which our sea level rises substantially. If we continue these practices as we’ve been doing them in the recent past, with no changes, these effects are going to continue, to worsen, and to add up cumulatively over time.
It also underscores the importance of continuing to monitor these changes, as knowing how the Earth is changing and how human activities are driving that change is of paramount importance as the 21st century progresses, and will become even more important as novel water security issues emerge. But over and above these issues, it highlights just how powerful swift action can be, as simply changing where we get our water from and how we distribute it to provide food (and, if I may be so bold to suggest, maybe not so many golf courses) to the 8+ billion inhabitants of our planet can change the rate and direction at which Earth’s axis migrates as well as the rate of sea level rise. After all, as the latest studies clearly show, local changes that we make in one place on Earth really do affect the entire world.