Schumann resonances: Amazing physics, sham medicine
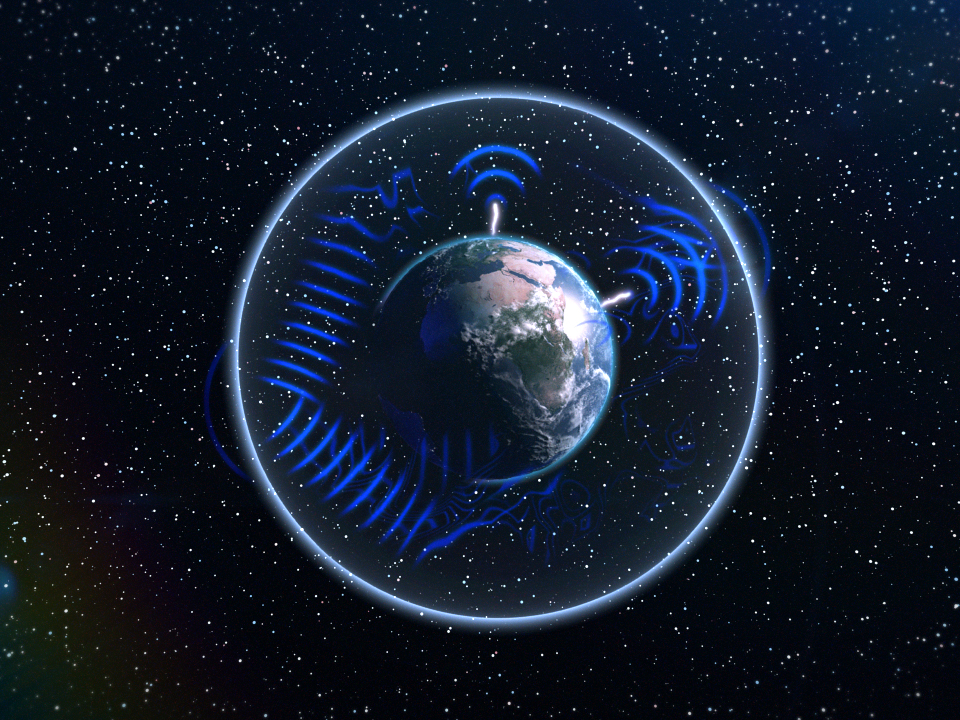
- Earth, in many ways, is a layer cake, with an atmospheric layer known as the ionosphere interacting with Earth's surface to behave like a closed, conducting waveguide.
- Driven primarily by lightning activity, standing electromagnetic waves encircle the globe in an omnipresent fashion, causing Earth to resonate in a series of Schumann resonances.
- Other worlds and planets may possess these Schumann resonances too, and this resonant phenomenon may help us understand many properties of planetary atmospheres in general.
From a physical perspective, all of planet Earth is simply one enormous layer cake. Deep in our planet’s interior, the densest material is found in our innermost core: a 400 mile (650 kilometer) wide ball of metal. Atop that is the inner core, made of those same metallic elements but configured into a different structural phase, surrounded by our liquid outer core. Atop that layer lies the Earth’s mantle — itself often subdivided into the four zones of upper mantle, the transition zone, the lower mantle, and the D” layer, which meets the outer core — and atop that floats our planet’s crust. Above the crust lies the oceans, followed by the atmosphere, which itself has several layers where various phenomena occur.
The lowest atmospheric layer, the troposphere, contains 80% of our atmosphere’s mass, and only goes up 9 to 11 miles (14-18 km) in altitude. Above that lies the stratosphere, containing the ozone layer, and then the mesosphere, which has a thin sodium layer. Above that, you can find the Kármán line — the artificial line between Earth and space — where the density of atmospheric particles plummet but their temperatures rise, leading to a swarm of ionized particles: the ionosphere. Between the ionosphere and Earth’s surface, a series of resonating electromagnetic waves known as Schumann resonances can be found. Although these resonances are real and reveal a tremendous amount of information about our world, there’s also an enormous amount of misinformation about them as well. Here’s how to separate fact from fiction.
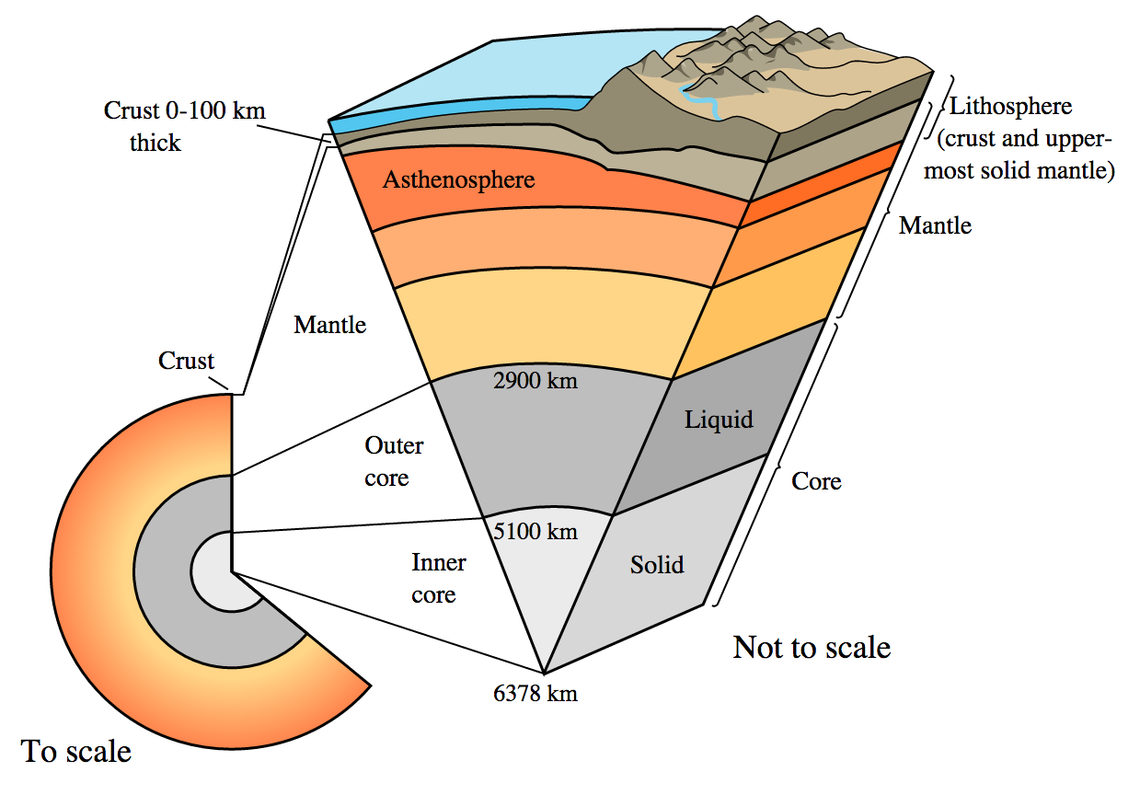
What’s important to recognize about the Earth is that two of its layers, the solid surface of the Earth and the tenuous ionosphere, are both relatively good electrical conductors relative to the rest of the planet. Because the materials that both the solid Earth and the ionosphere are made from are good conductors, not only can electrons be readily transported through them, enabling the flow of electricity, but electromagnetic waves — i.e., light — can be reflected between them.
The history of this goes way, way back to the late 19th century, and the work of Irish physicist George Francis FitzGerald. FitzGerald’s greatest claim to fame was in the aftermath of the famed 1887/1888 Michelson-Morely experiment: the experiment that attempted to detect the motion of Earth through the then-hypothesized aether. Michelson and Morely built the world’s most sensitive interferometer, a device that:
- splits light into two perpendicular waves,
- allows those waves to propagate equal distances until they’re reflected by mirrors,
- then brings them back together at the same point they had previously been split,
- and then measures how those waves interfere with one another: constructively, destructively, or otherwise.
By orienting their interferometer at various angles with respect to Earth’s motion, Michelson and Morely attempted to measure the speed at which Earth, rotating on its axis and revolving around the Sun, moves relative to the aether that light propagates through. Their null results suggested no evidence for this aether, and would eventually lead Einstein, in the early 1900s, to develop his theory of relativity.
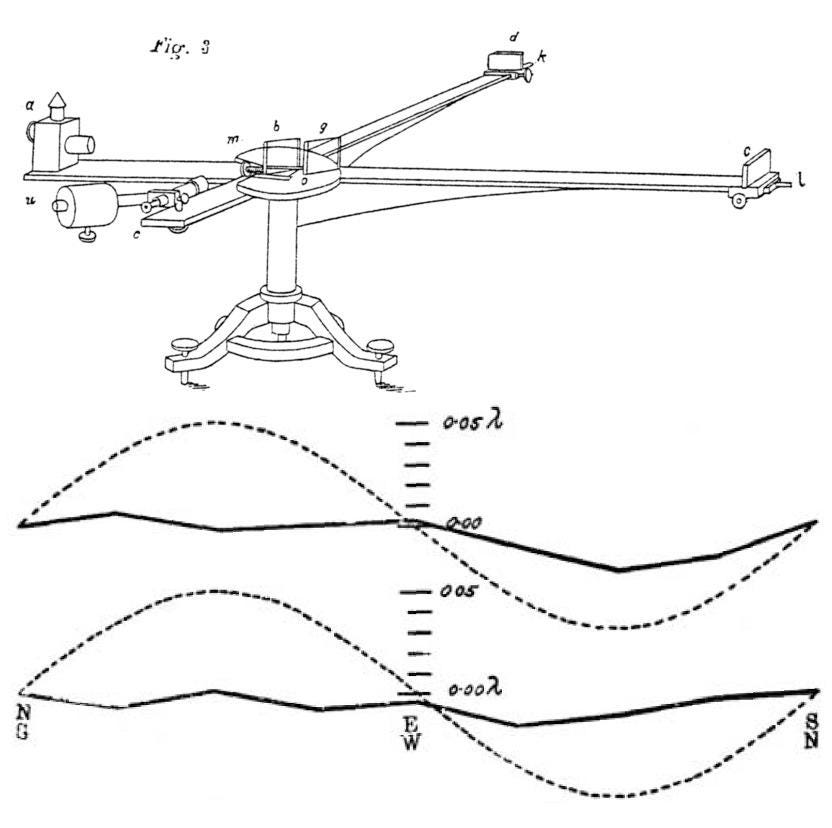
But before that, in 1889, FitzGerald wrote a letter to the editor of Science magazine, noting that if all moving objects were foreshortened (i.e., contracted) along their direction-of-motion, this could explain why Michelson and Morely achieved a null result for their experiments. Three years later, in 1892, Hendrik Lorentz would derive the full set of transformations governing both length contraction and time dilation, and in 1905, Einstein would fully piece together special relativity. Even today, the phenomenon of length contraction bears FitzGerald’s name, as it’s also known as the Lorentz-FitzGerald contraction.
But in 1893, FitzGerald turned his attention toward Earth and its electromagnetic phenomena, noting that the upper layers of the atmosphere must conduct and therefore they themselves should vibrate when stimulated, similar to how a bell vibrates and resonates when struck. He estimated the period of vibration for this conducting layer to be on the order of one oscillation per second, or what we’d call 1 Hz (Hertz) today. This was the first inkling we had, as a species, that the Earth itself may be exhibiting the phenomenon of electromagnetic resonance on a planet-wide scale.
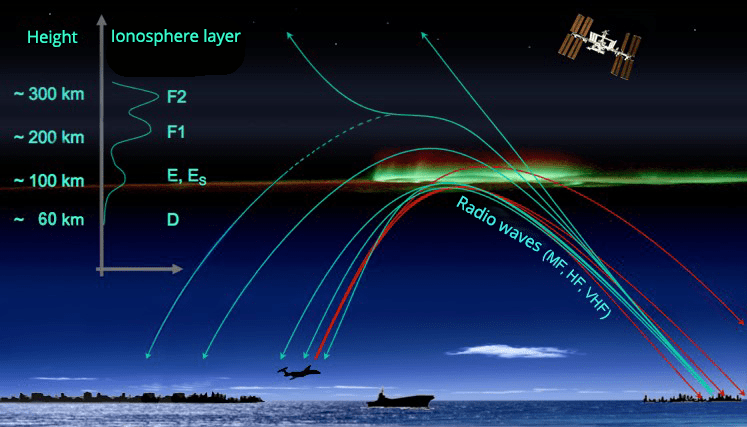
The subsequent decades would see many developments in both theoretical physics and observational astronomy. Working with Maxwell’s equations, physicists and engineers began to understand the science of waveguides, and how both electromagnetic waves and fields behave within resonant, conducting cavities. The mechanism for how Earth’s atmosphere would behave as a conductor was suggested by theoretical physicist Oliver Heaviside and electrical engineer Arthur Kennelly in 1902, and the existence of the ionospheric layer was finally experimentally demonstrated in 1925 by Edward Appleton and Miles Barnett.
Finally, in 1952, physicist Winfried Schumann put the whole picture together. He recognized that the space between the solid (conducting) Earth’s surface and the hot, rarefied (conducting) ionosphere some ~100 kilometers up would behave like a cavity capable of reflecting electromagnetic waves: a waveguide. Simultaneously, he realized that the electric currents created by lightning strikes would be able to naturally excite this cavity and create resonances. Just as a musical instrument has frequencies at which the waves within it vibrate, creating sounds, the electromagnetic waves traveling within the space between the Earth’s surface and the ionosphere would lead to the resonant vibration — at specific sets of frequencies — for the cavity itself.
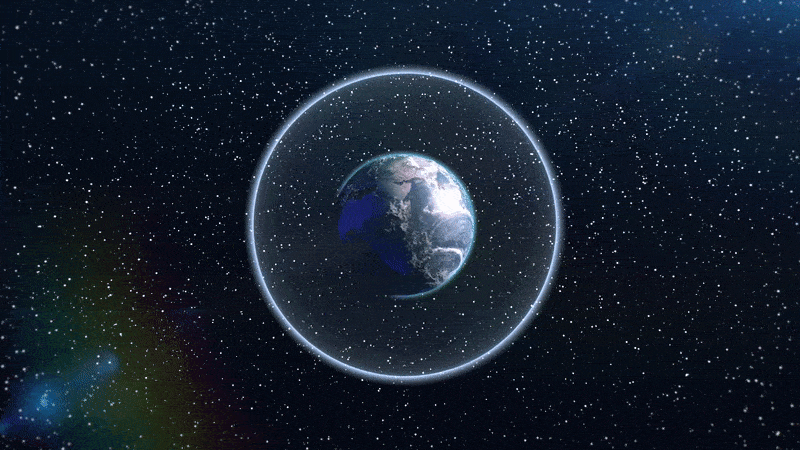
Based on the circumference of Earth, the speed of light, and the way that resonant frequencies work inside cavities, Schumann was able to calculate a basic formula for the frequencies that the ionosphere should resonate at. Because the Earth is large (~40,000 km in circumference), but light travels between 7 and 8 times that distance in a second (the speed of light is ~300,000 km/s), Schumann correctly predicted that the fundamental frequency that the ionosphere would vibrate at would fall within this range.
Using modern equipment and with a more advanced understanding of the electromagnetic phenomena at play interfacing between the Earth’s surface and the ionosphere, including:
- the finite conductivity of the ionosphere,
- day-night differences in the height of the ionosphere from Earth’s surface,
- the asymmetrical nature of Earth’s magnetic field, particularly variations in latitude,
- the absorptive behavior of Earth’s polar ice caps,
- and small variations in the Earth’s actual radius from place-to-place,
the actual Schumann resonances have been found to occur at 7.83 Hz for the fundamental frequency, and then increase by somewhere between 6.2-6.5 Hz for each “overtone” above that fundamental frequency, with the peaks broadening more substantially at higher frequencies.
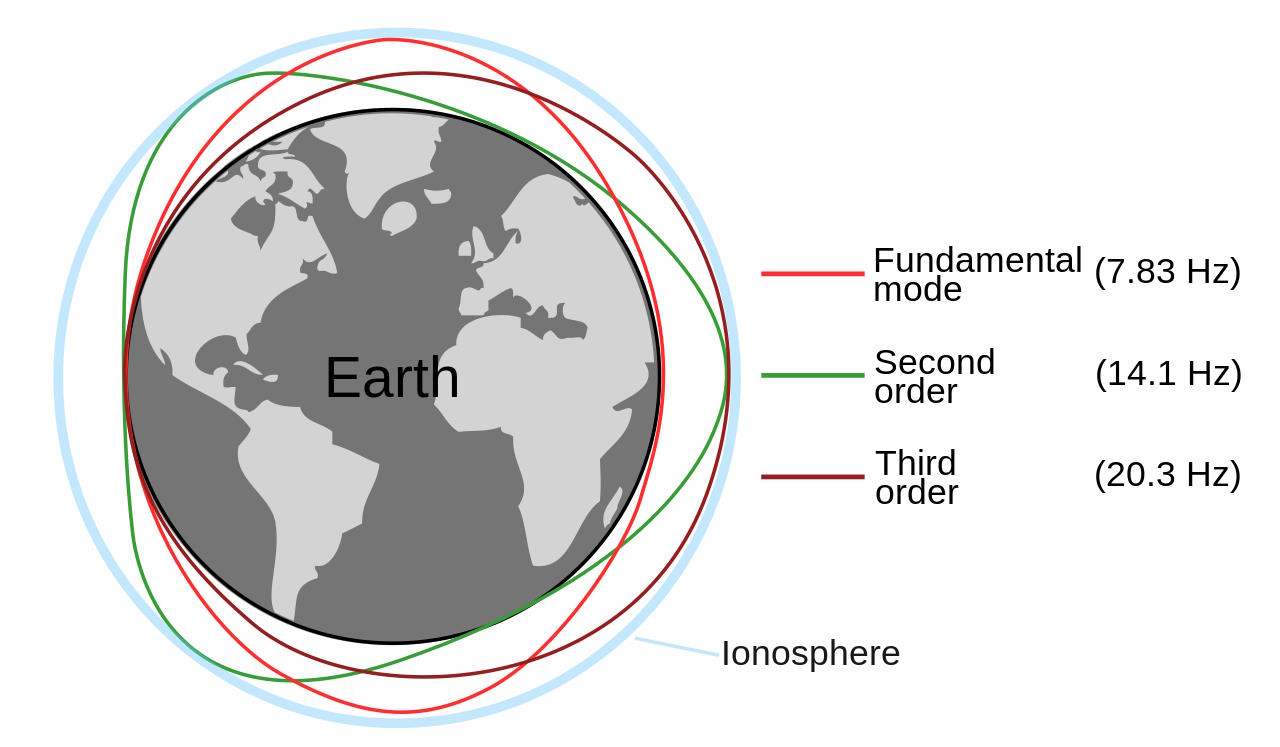
However, even though lightning strikes and electrical discharges only occur in sporadic bursts across the Earth, our planet is large enough that, cumulatively, these Schumann resonances are practically always being excited. At any given moment in time, there are approximately two thousand independent thunderstorms occurring on Earth, and approximately 50-100 individual lightning strikes occurring each second on our planet all together.
Although these lightning strikes generate electromagnetic waves of a wide variety of frequencies, it’s only the electromagnetic waves that have the right sets of frequencies — frequencies that fall into the broad peaks of the various Schumann resonances — that will combine, interfere, and resonate within the electromagnetic cavity created by the boundary conditions set by the Earth’s surface (from below) and the Earth’s ionosphere (from above).
The “sweet spot” for the resonance requires it to be a distance that’s in integer multiples of the distance the waves can travel (between the Earth’s surface and ionosphere) to complete a full revolution around the Earth, which is why it’s only these resonant frequencies that get amplified. The result is a “ringing” that permeates Earth’s atmosphere at these specific Schumann resonances.
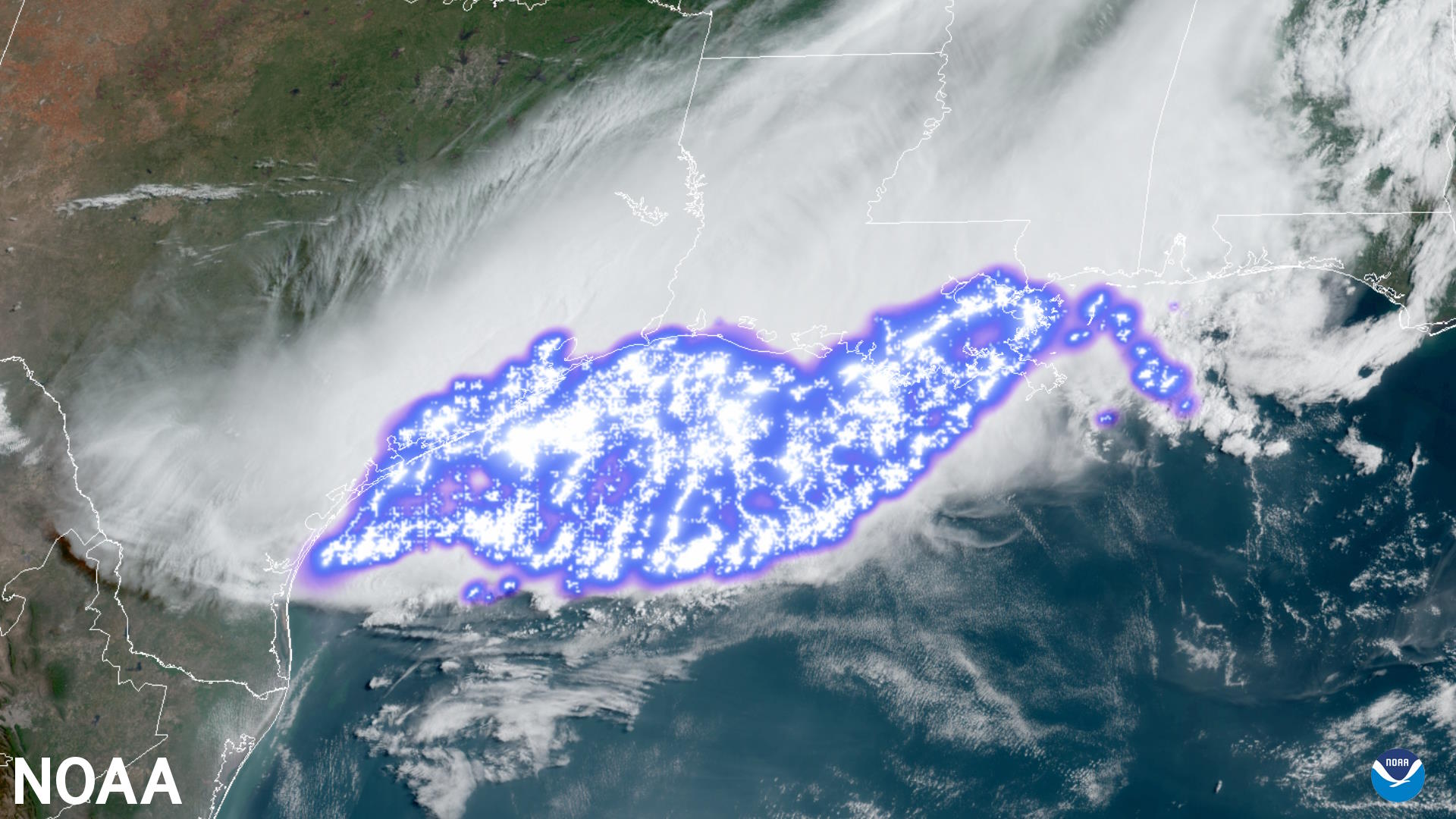
Poetically, these resonant frequencies, with 7.83 Hz as the lowest (fundamental) frequency, are sometimes referred to as Earth’s “hum” or even as the atmosphere’s “heartbeat,” and there’s both very good science and also very dubious, unproven science that these resonances have been linked to.
On the good side, Schumann resonances are studied by physicists, engineers, climate scientists, and meteorologists to track global lightning activity. When there are ionospheric disturbances, such as due to space weather, auroral activity, or other phenomena that can affect Earth’s geomagnetic configuration, effects can be seen by monitoring these Schumann resonances.
Here on Earth, a number of upper-atmospheric phenomena have long been observed, but have only recently been characterized, including:
- sprites,
- ELVES,
- jets,
- and upper-atmosphere lightning.
These phenomena, when observed, have been linked to time-variations seen in the behavior of Schumann resonances, sparking further interest in them and how Earth’s ionosphere interacts with them.
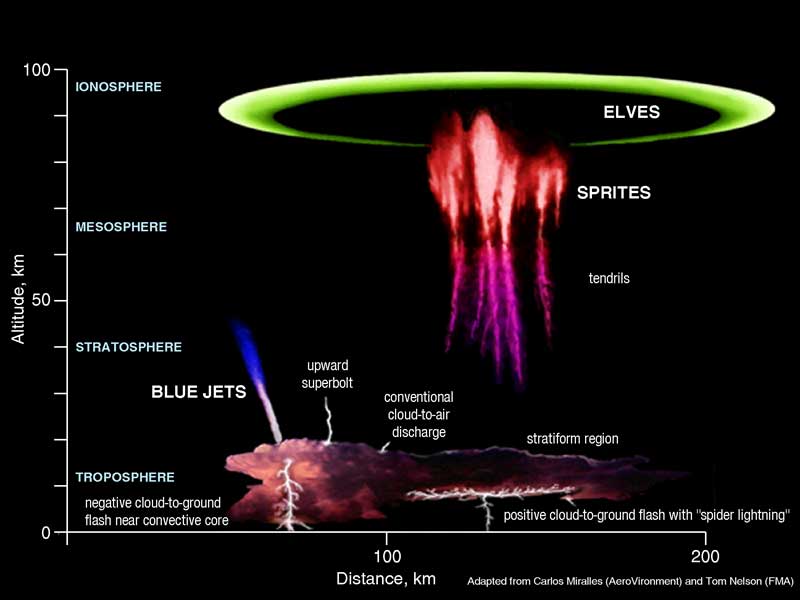
One of the most interesting fields of study to recently emerge around Schumann resonances is in the field of global warming. As the temperature of Earth rises, so does what’s known as the lightning flash rate, or the global rate of lightning strikes across our planet. By monitoring these Schumann resonances across time, they’ve been shown to make an interesting long-term global tropical thermometer, providing an unintuitive but important metric which can be used to quantify climate change.
Other planets, so long as they have conductive layers separated by a non-conductive layer along with significant electrical discharges (i.e., lightning strikes), are potential candidates for possessing Schumann resonances of their own. These include:
- Venus,
- Mars,
- Jupiter,
- Saturn,
- and even Saturn’s moon Titan,
the last of which has the most massive atmosphere of any known moon in the Solar System. While evidence for lightning is substantial on Venus, Jupiter, and Saturn, the evidence for lightning on Mars is only indirect, and the evidence for lightning on Titan is dubious, although Saturn’s magnetosphere may induce ionospheric currents on Titan. However, whether Jupiter or Saturn have a conducting “lower layer” to enable the Schumann resonance is presently unknown.
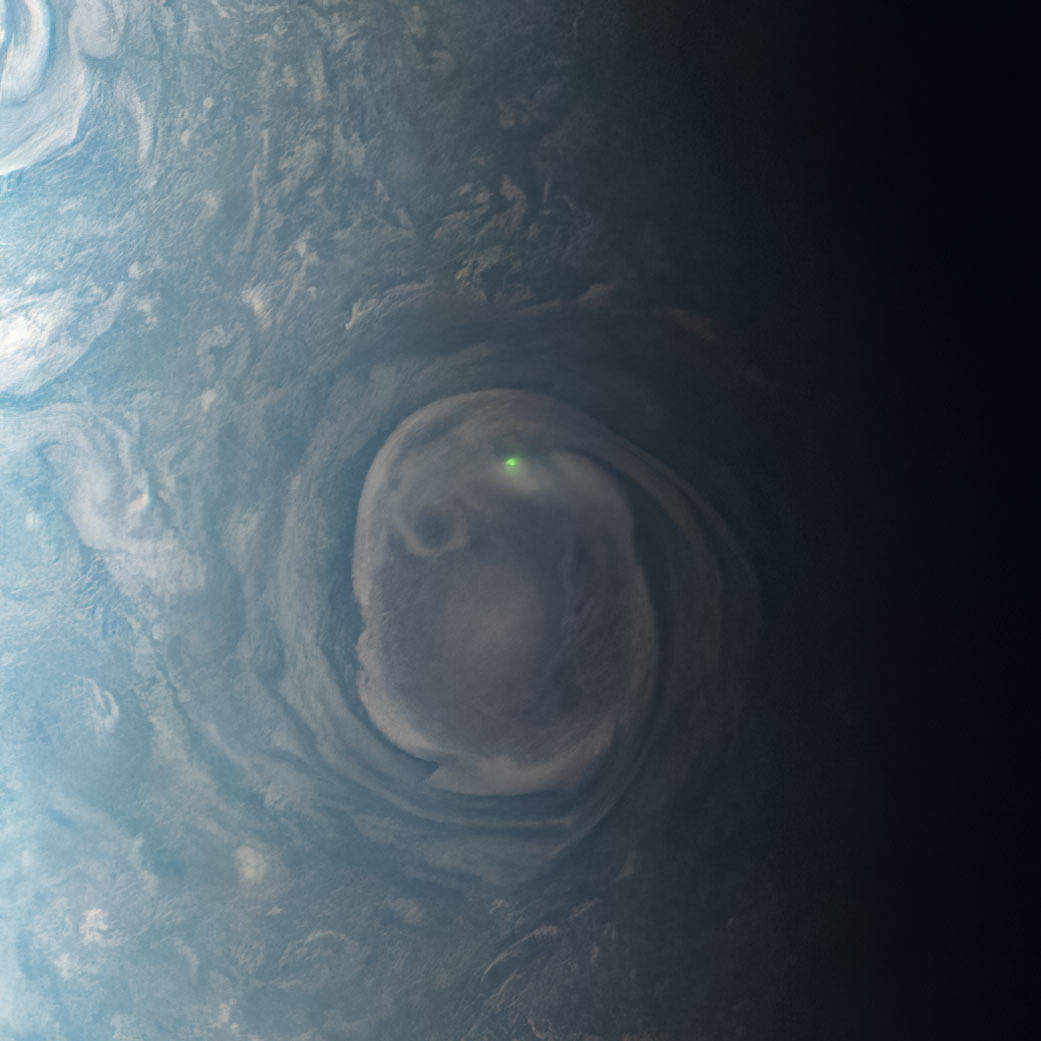
However, there are many very speculative studies — many of which are peer reviewed but all of which are unproven — claiming that Schumann resonances can be biologically or geologically useful in wholly unproven ways. Some want to use biophysical devices that “vibrate” with Schumann resonances to treat all sorts of conditions, from tinnitus to neurodegenerative conditions and far beyond. Claims have been made that the Schumann resonance can be used to locate offshore hydrocarbon deposits: a dubious assertion that has only been made by one documented author.
There are no known mechanisms for the interplay of low-frequency electromagnetic waves — waves which would have wavelengths of several thousands of kilometers, even for excitations beyond the fundamental (lowest) Schumann resonance — with any biological systems; these are ideas that scientists are free to explore, but that lack any supporting evidence behind them. Just as there are many supernatural/paranormal speculations about phenomena like “the hum,” there is real science behind Schumann resonances, but also a lot of bad science, nonsense, and conspiracies related to them.
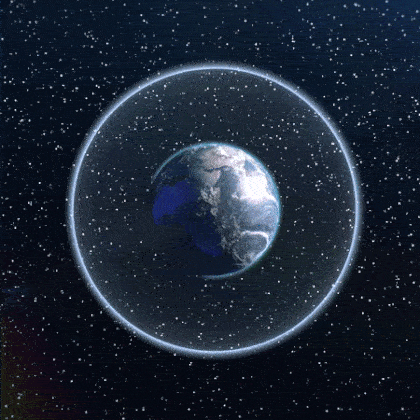
However, the real, genuine science is that the combination of:
- electromagnetic activity and large electric currents here on Earth, primarily driven by lightning strikes occurring continuously across our planet,
- along with the conductive nature of our planet’s surface and ionosphere separated by the insulating atmosphere between them,
provides the right conditions for electromagnetic resonance. These resonances have supporting science for them going all the way back to the 19th century but were first explicitly predicted and quantified by Winfried Schumann back in 1952.
Since that time, we’ve determined that other planets and worlds may possess these Schumann resonances as well, and that as lightning activity and other ionospheric or geomagnetic activity occurs, these Schumann resonances will be affected as well. However, beware of claims purporting to use these Schumann resonances to affect your body or any biological system; these often evidence-free claims are wild, unsubstantiated, and fail to align with our modern understanding of how the physical world actually works. The entire Earth is resonating with electromagnetic waves, and Schumann resonances explain how.