Ejection Failure! Scientists Decode Why Our Sun’s Events Sometimes Fizzle

Watch the plasma slide down the prominence like a roller coaster!
Our Sun, despite it’s outward appearance as a perfectly hot sphere, is anything but uniform. When take a closer look at the photosphere, we start to see how intricate its imperfections are. In addition to sunspots — areas of the Sun that are so much cooler than average that they appear as dark regions to the human eye — the Sun is also divided into a series of roiling cells on its surface, with hot plasma “spots” between them. But perhaps the most outstanding feature of our Sun are these loops and filaments of plasma extended high off the Sun’s outer surface, tracing out the Sun’s strong but chaotic magnetic field.
These plasma loops, and the magnetic fields that underpin them, store a tremendous amount of energy. When the right conditions arise, these loops can break apart at a critical moment, reconnecting with other elements of the magnetic field found throughout the Sun or even extending into the solar corona. A solar prominence can give rise to a coronal mass ejection: a violent space weather event capable of causing aurorae and electrical grid disruption all over the globe. But recently, a particularly interesting “failed” coronal mass ejection was discovered, and its properties might just help us decode why some solar events sizzle while other’s fizzle out entirely.
The nightmare scenario, of course, is something akin to the great Carrington Event of 1859. Back in the mid-19th century, solar astronomy was in its infancy as a science, when astronomer Richard Carrington — who happened to be watching a particularly large set of sunspots — saw something spectacular. Dancing along those sunspots for only a few minutes was a “white light flare” that could be seen even against the overwhelming brightness of the Sun, followed by a sudden stop. Although we didn’t know it at the time, a coronal mass ejection had just occurred.
Just about 17 hours later, the effects of that coronal mass ejection began to appear on Earth. The aurorae went wild, appearing all over the globe, even at equatorial latitude. It caused workers on the Earth’s night side to awaken, as the light was bright enough to confuse humans with the impending dawn. And, perhaps most frighteningly, our early devices powered by electricity, such as telegraphs, began activating automatically, even when they were disconnected entirely from a power source. In some places, the telegraph devices tapped so powerfully that the paper that recorded their signals caught fire.
What was happening wasn’t greatly appreciated at the time, but we now widely recognize what occurred as an example of the enormous effects that space weather can have on Earth. Two of Earth’s defining characteristics are:
- its relatively thick atmosphere, which prevents even energetic charged particles originating from our Sun from reaching the surface of Earth,
- and its magnetic field, which functions like a large magnetic dipole, causing charged particles that enter the influence of our magnetic field to mostly be diverted away, with only a small fraction of them getting redirected by Earth’s magnetism to produce a “ring” of particle collisions around both the north and south magnetic poles.
When the Sun is quiet, which is to say it isn’t undergoing any major ejection events, the stream of particles from the Sun is relatively constant: the solar wind. However, these flare-like events, when they occur, they can not only intensify the solar wind, they can create particles that are faster-moving, more energetic, and that can disrupt and even penetrate Earth’s own magnetic field.
While we normally think of the Sun as being somewhat localized in space, the greater truth is that the solar corona — and the Sun’s magnetic field — actually extends very far into space, even encompassing the entire Earth. When the Sun sends off an energetic event such as a coronal mass ejection, the solar magnetic field and the Earth’s can interact, and if they connect in just the right (or wrong, depending on your perspective) fashion, it can create a funnel-like effect for bringing these particles down around Earth’s magnetic poles in great numbers.
These fast-moving charged particles still won’t reach the surface, but they can change the magnetic field at Earth’s surface significantly in short periods of time. Changing magnetic fields, wherever you have a loop or coil of wires (especially large-area ones), will induce currents in those wires, and that can cause:
- power surges,
- electrical discharges,
- massive voltage changes,
- fires,
and many other ill effects for our infrastructure. While the direct danger to humans from such a space weather event is low, the secondary danger, as a result of fires, power loss, and damage to our vital infrastructure, could rise to a price tag of multi-trillion dollars. If a Carrington-like event were to occur today, we are not sufficiently prepared; the worst of those consequences would not be mitigated in any meaningful way.
But not every solar eruption results in a coronal mass ejection. In fact, there are three main type of solar eruptions, and coronal mass ejections are just one of them: the largest and most powerful, but not the only option by any means. In fact, coronal mass ejections may be the rarest of these solar eruptions.
More commonly are smaller, less energetic events known as jets. These wind up being small, thin columns of plasma that get injected into the solar wind; they have only a negligible effect on Earth’s space weather. They seem to originate from smaller, weaker plasma loops, and don’t consist of large numbers of energetic, fast-moving particles. As far as the “normal” solar wind goes, a jet event adds only a slight enhancement.
But there is a third type of event: failed prominence eruptions. These are where large, beautiful plasma loops — commonly seen as solar prominences — extend far away from the Sun’s photosphere and can even enter the Sun’s corona. Instead of small jets or large coronal mass ejections, however, we basically see an unsuccessful eruption: the plasma just peters out, and winds up falling back onto the Sun.
(Seriously, it’s a very impressive video.)
The question, of course, is why?
To understand that, you have to understand what’s occurring when you have a successful coronal mass ejection. There are a few ways to make this happen, but there are commonalities between them.
- They always involve magnetic fields from different parts of the Sun creating large loops that are followed by the hot solar plasma.
- These fields from different parts will interact and, at a critical moment, reconnect with one another.
- Depending on the exact geometry of the magnetic fields and the precise way that the field lines from various parts reconnect, you can get a few different mechanisms: kink-instability eruptions (if the prominence has a significant enough twist to it), torus-instability eruptions (a different type of magnetic reconnection), or solar breakouts (an alternative to either instability mechanism), where the fields reconnect inside the Sun and causes a flare-like eruption.
At this point in time, we cannot say for certain which of the three mechanisms are responsible for the majority of major eruptions, but we can say, for absolute certain, that not all of the massive prominence loops we see will end in an eruption.
Previous work had focused on observing how prominences that looked like they might erupt would instead fail, which noticed a number of fascinating clues. First off, when they examined the filament spines — the proverbial “backbones” of these prominences — they found no significant rotation or twisting in the prominences that failed to erupt. In addition, the way the filaments, once they failed to erupt, fell back onto the Sun indicated that gravitation, and not any sort of electromagnetic force, was the driving factor at play.
But in 2016, a team of researchers saw a new failed prominence, and the clues just didn’t add up. Based on all the features that were there, including the size and magnitude of the prominence, the fact that magnetic reconnection occurred, and the fact that it had a hot plasma “cap” (or dome) atop a cooler ring of prominence plasma, they fully expected a coronal mass ejection to result. But what happened instead was a whimper: the hot plasma cap simply lifted off gently, creating a wide version of a weak jet, while the cooler prominence utterly failed to erupt at all, simply draining back along the filament to the solar surface.
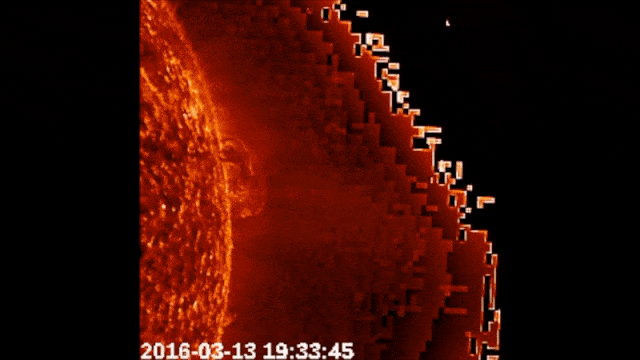
According to Dr. Emily Mason, lead author of the recent paper that analyzed this failed eruption along with Spiro Antiochos and Angelos Vourlidas,
“The three of us that wrote the paper spent 18 months staring at this event, arguing over mechanisms, dropping it, then circling back a few months later. It just wouldn’t leave us alone; it confronts us with glaring gaps in our knowledge of the Sun, but also teases us with the hope that if we can just explain this event, we’ll have made real progress.”
The big unknown, unfortunately, is to figure out what exactly happens to the spine of these filaments magnetically, since the particulars of the magnetic reconnection events are likely what powers (or fails to power) the potential eruption that would ensue. The odd thing about this particular failed prominence is that it looks like the spine blows outwards initially early in the eruption. Is the magnetic field moving? Or is it simply transporting the hot plasma while the field itself remains stationary? Both options have problems and both remain viable; it’s still an undecided question.
Nevertheless, this observation offers a fantastic potential for understanding three phenomena all within a unified framework for the first time. Remember, when this prominence failed to erupt, the upper, hot cap of did fly off away from the Sun, but only gently, slowly, and in a broad, rather than collimated, fashion. Meanwhile, the lower, cooler portion didn’t simply fall down as though gravitation was the dominant force, but slid backwards along the same filament — and likely the same magnetic field — that traced out the prominence earlier. In the words of the authors, the cooler plasma slid back, like cars along a roller coaster track.
This allows us to create a unified model of jets, failed eruptions, and coronal mass ejections all at the same type. Jets are the smallest structures, where there’s only the cool plasma tracing a minuscule prominence; when magnetic reconnection occurs, there’s only a slight eruption. Coronal mass ejections are the largest, connecting the photosphere to the corona, where reconnection can cause an enormous release of energy. And now, we have these failed eruptions, which seem to be in between, exhibiting some features of jets and coronal mass ejections, but where the fallback of the cooler plasma is the dominant effect.
The next steps to this research will be to scale up computer models, attempting to understand which underlying magnetic structures and reconnection processes can successfully reproduce these peculiar dynamics of such a failed eruption. On the small end of things, the events that lead to jets are relatively isolated in terms of their magnetic properties. Coronal mass ejections, however, are complicated, with three distinct mechanisms currently in contention for powering the majority of them. But the failed eruptions are somewhere in between, and now the puzzle is to figure out precisely how.
As Mason explained, “If we can essentially scale up what we already know about jet eruptions, we may gain important insights on how CMEs erupt as well.” The mystery remains unsolved for now, but humanity will get a new science tool in its solar arsenal in just 5 months: when the Daniel K. Inouye Solar Telescope begins its full science operations. With its Cryo-NIRSP instrument for observing the corona, and the ability to extrapolate the magnetic field configurations in the low corona, all three sets of eruptions might soon be fully explained. If we can sufficiently measure and understand the interplay of magnetic fields with the Sun’s plasma, flares, and coronal events, perhaps the next Carrington-like event won’t come as such a surprise to humanity, giving us the key ingredient we need to prepare: time.
Starts With A Bang is written by Ethan Siegel, Ph.D., author of Beyond The Galaxy, and Treknology: The Science of Star Trek from Tricorders to Warp Drive.