Earth is the Solar System’s densest planet. It shouldn’t be.
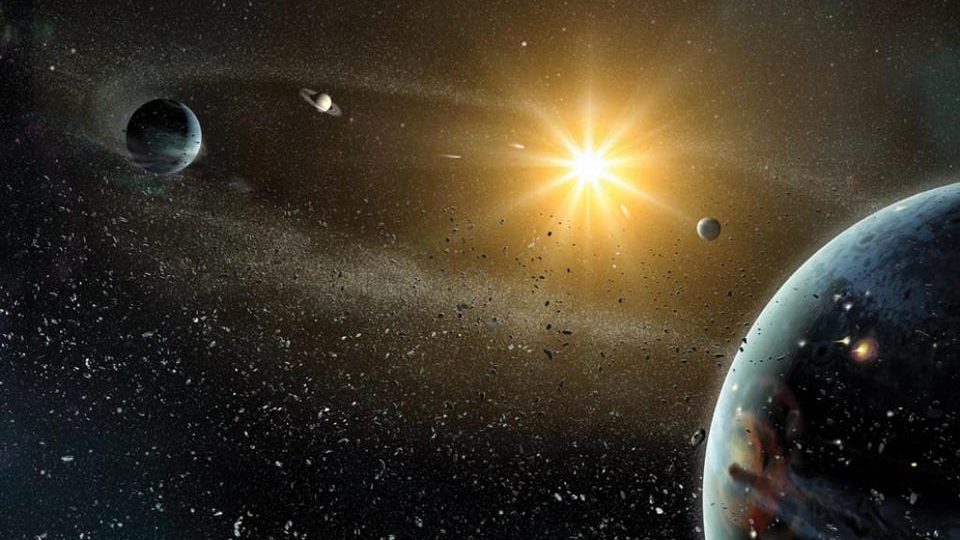
- When solar systems first form, the heaviest elements sink preferentially towards the central protostar, while lighter elements are easily blown away.
- Based on their atomic composition and ratios alone, Mercury ought to be the densest planet.
- But Earth is even denser, owing to a combination of its composition and gravitational compression. Here’s why the full story matters.
When it comes to the Solar System, the elements that make up each planet are determined by how they all formed. Closest to the Sun, the high temperatures, large amounts of solar radiation, and intense solar winds can easily kick the lightest elements off of any protoplanets that are forming. But farther away from the Sun, these factors have more difficulty expelling light elements. As a result, we wind up with planets that are disproportionately made of heavier elements the closer you get to the Sun, and that have lower density compositions — and large amounts of lighter atoms — the farther out we venture.
In the innermost part of the Solar System is the planet Mercury, which has only a negligible atmosphere and is made largely of rocky and metallic material. As we travel farther away from the Sun, atmospheres become common, as do greater proportions of lighter elements. If we were to look at each planet’s composition in terms of the atoms that make it up, Mercury would have the highest percentage of heavier elements, trailed by Venus and Earth, with Mars even farther behind.
You might think that would make Mercury the densest planet, but that’s not true at all. If you measure each planet’s mass and divide it by its volume, it turns out that Earth, not Mercury, is the densest world in our Solar System. Here’s the surprising science behind why.
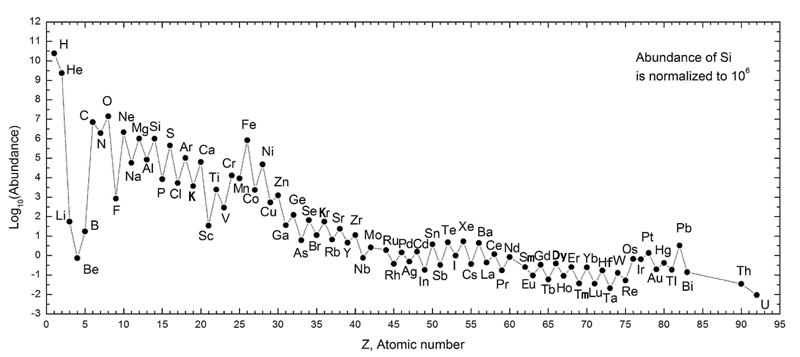
Credit: 28bytes/English Wikipedia
What is it, precisely, that makes up the various planets? On a superficial level, the answer is straightforward: atoms. If we rewind the clock some 4.6 billion years, back to when our Solar System was first forming, we would have watched multiple competing processes all work against one another when it came to creating not just the major planets, but every body in the Solar System. From an initial cloud of collapsing gas, a large number of massive clumps — gravitational imperfections — form and begin growing. The clumps that get large enough fast enough will preferentially attract their surrounding matter, and the cosmic “winners” in this gas clouds are the ones that will emerge with at least one star within them.
Our central protostar represents one such clump, and when it first formed, it formed along with a protoplanetary disk, like practically all protostars we know of. This disk, even in the early stages, is going to be made of a wide variety of materials: all of the atoms that were present in the molecular cloud that collapsed to form us. However, the central location of the protostar is key: the location where the most mass gathers is also the infant Solar System’s source of:
- heat
- electromagnetic radiation
- high-energy particles
- gravitation
Put simply, it’s the primary external factor driving the evolution of all the surrounding matter.
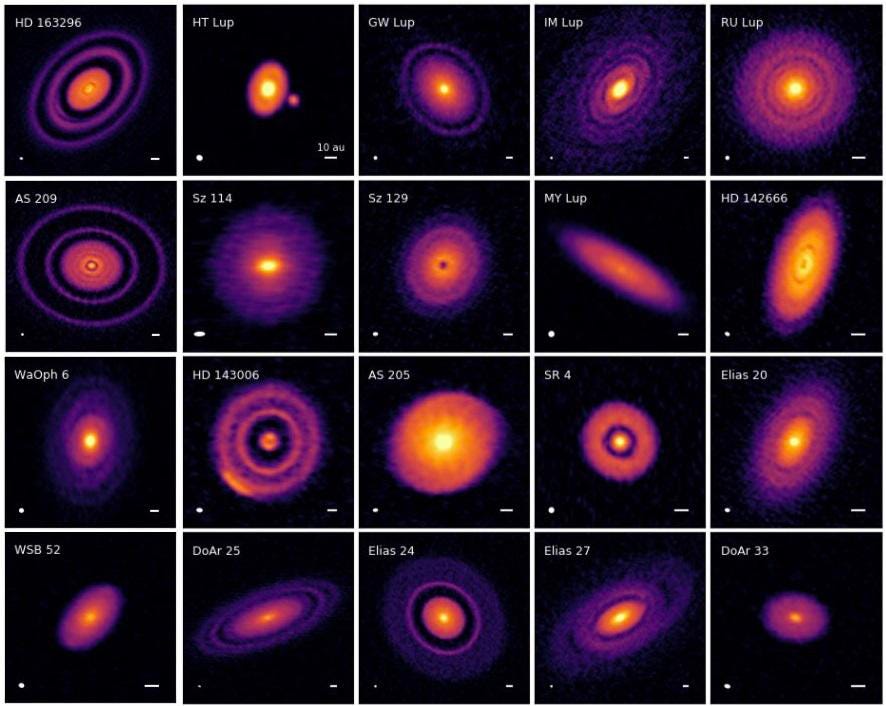
Credit: S.M. Andrews et al., ApJL, 2018
The most common configuration for Solar Systems — which we’ve only learned of through directly measuring the properties of protoplanetary disks that are in the process of forming planets around other newborn stars — is that they form planets in a single plane. As our Sun was in the process of forming, gas was being drawn into it, while a large, diffuse, and dusty disk of matter formed around it.
The reason you get a disk is the same reason that spiral galaxies form a disk: Whenever you have a clump of matter, it’s going to be asymmetrically distributed, and one axis will inevitably be shorter than the other two. That axis will collapse before the other two, and since the material is made of normal matter, it will go “splat” in that direction.
You might bristle at how unscientific the word “splat” sounds, but the scientific term isn’t much better: we say that it “pancakes” instead. Because normal matter is made up of atoms and their constituents, we shouldn’t be surprised that they go “splat” when they collide, and that splat means that they heat up through collisions and friction. After a very short amount of time, we wind up with a disk of matter that rotates around the central, growing, and heating mass.
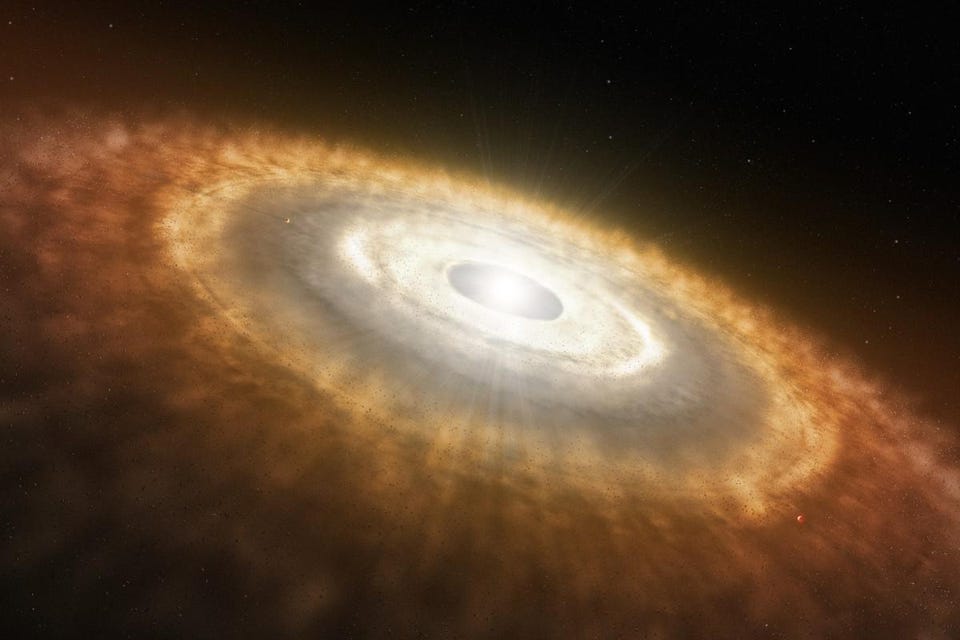
(Credit: ESO/L. Calçada)
This is pretty typical for a protoplanetary disk. But what comes next is a cosmic race between these important competing factors. Here’s what’s at play.
- Gravitational imperfections exist in this disk, and will lead to clumps of matter forming, growing, and working to attract the surrounding material onto them.
- When clumps collide, they can either stick together and accelerate their growth, or they can smash one another to smithereens, leading to fragmentation and a repopulation of the disk.
- Meanwhile, the radiation and particles emanating from the central star will effectively push out the particles it encounters, kicking them to higher, less tightly-bound orbits.
This sounds like a very simple race at first pass. The imperfections will race to form planets and gobble up as much mass as they can, while the central, protostellar engine will race to blow that planet-forming material away. As time goes on and the protostar evolves, it heats up, which means it preferentially pushes away volatile, low-density material more effectively, particularly the material that’s closest to the central star itself.

Credit: NASA/JPL-Caltech/Invader Xan
To no one’s surprise, the biggest, earliest clumps that form will be the most successful, sweeping up all of the matter in their orbits and from surrounding orbits. They won’t just form a single, massive planet, but will form their own miniature protosystem as well; the giant planets all formed circumplanetary disks, the analogue of the protoplanetary disks that form around the newborn stars.
Additionally, the clumps that form the earliest will draw in a mix of the three different types of matter that are present in these early protoplanetary disks:
- heavy metals, which will quickly sink to the cores of these massive clumps
- mantle-like material, primarily made of silicates and other rocky particles
- volatiles, or light elements and compounds, that are easily boiled away when subject to heat
This, right here, is the recipe for forming everything that we see in our modern Solar System.
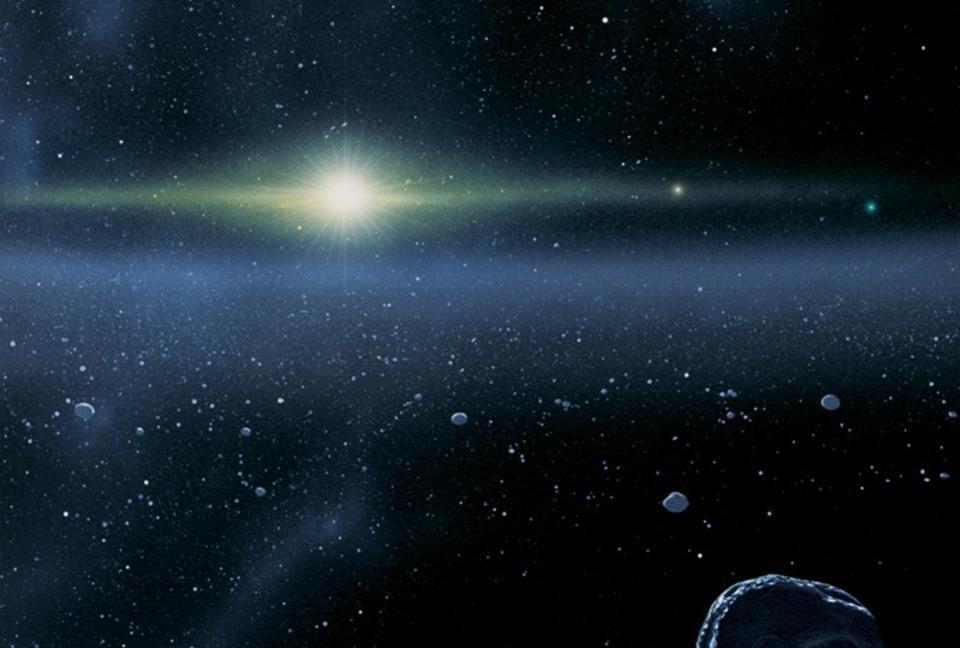
Credit: JHUAPL/SwRI
The volatiles are most easily blown away from the inner Solar System, while the heavier elements are the only ones that remain. The innermost planet, Mercury, should therefore be made out of the heaviest elements, and hence you’d expect Mercury to be the densest planet. Venus and Earth are farther out, but they’re both substantially more massive than Mercury, indicating that they probably formed a little bit earlier on. When a planet forms early, it can draw larger amounts of mass into itself, since there’s more mass available, and that means you’re likely to get slightly different ratios of materials: probably less metals, more mantle-like material, and some of the volatiles that the innermost planet lacks.
Farther out, we expect Mars to be made of less dense material than any of the other three terrestrial planets. The asteroids should be somewhat less dense than Mars, on average, though it’s possible that some asteroids had their volatiles completely boiled away, leaving a denser core behind. Jupiter was probably the earliest clump to form in our protoplanetary disk, and likely has a massive, dense core that’s many times the mass and size of Earth. The hydrogen and helium it possesses are this pristine, volatile material, but Jupiter can hold onto it only because of its enormous mass. However, out at Jupiter and beyond, all moons, planets, and other bodies possess lower overall densities than the inner, terrestrial planets do.
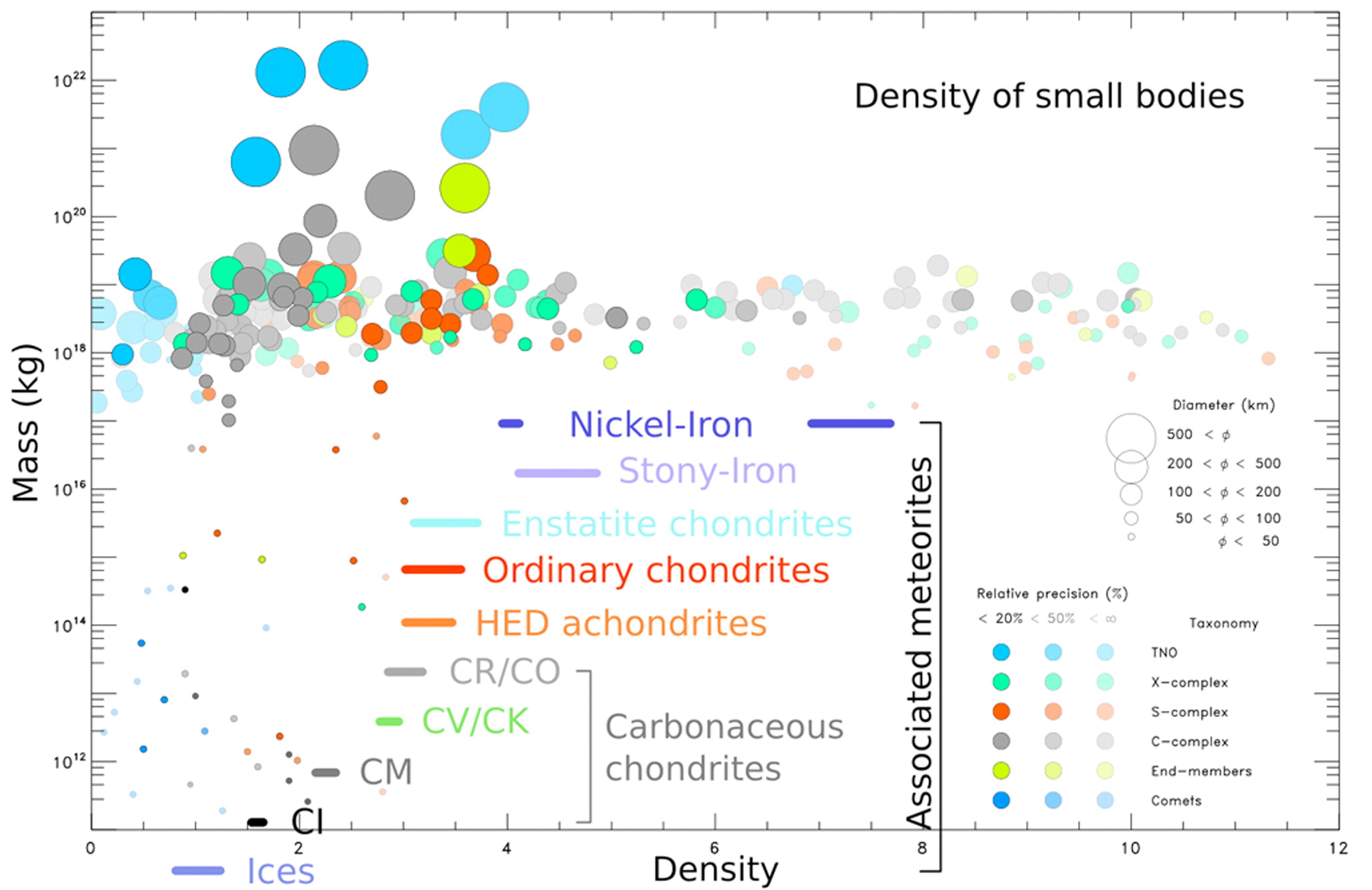
So, perhaps naively, you’d anticipate that Mercury would be the densest planet, followed by Venus, then Earth, then Mars, and then the giant planets in some order. If you included the moons of those giant planets and the asteroids as well, some of them might be competitive with Mars — the least dense of the rocky planets — in terms of density. But they’d all be much smaller and lower in mass. Certainly you’d expect that none of these other worlds would be able to hold a candle to the innermost three planets of the Solar System.
In fact, that turns out to be the case, except for one giant surprise: Mercury, Venus, and the Earth are the three densest worlds in the Solar System. Mars is the next most-dense object, followed by Jupiter’s innermost large moon, Io, then by Earth’s moon, and then by Jupiter’s second large moon, Europa. Every other large solar system world is less dense than granite and basalt, typical rocks on Earth’s surface, and only a few other worlds are even suspected of having a metallic core.
And yes, Mercury is just a little bit denser than Venus, just like you’d expect. But Earth? Surprisingly, Earth has them all beat. In terms of density, Earth is the record-holder in our Solar System; no other world exceeds our own planet’s density.
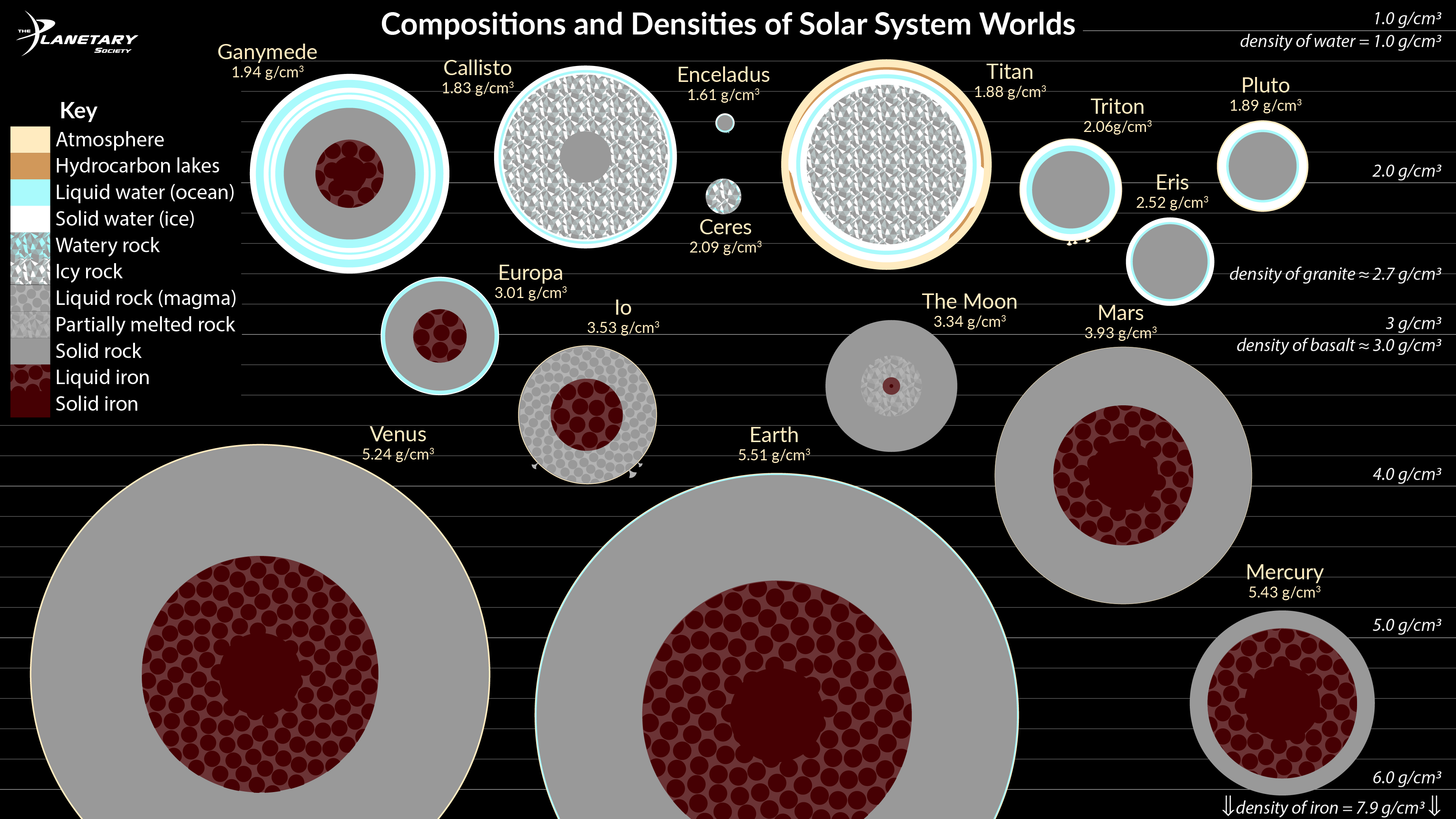
Credit: Bruce Murray/The Planetary Society
This should be a surprise. After all, if we were to list the densities of the eight major planets, simply by measuring their masses and volumes, here’s what we’d find. In units of grams per cubic centimeter (g/cm3), where water has a density of 1 g/cm3, the densities of the planets are:
- Mercury: 5.43 g/cm3
- Venus: 5.24 g/cm3
- Earth: 5.51 g/cm3
- Mars: 3.93 g/cm3
- Jupiter: 1.33 g/cm3
- Saturn: 0.69 g/cm3
- Uranus: 1.27 g/cm3
- Neptune: 1.64 g/cm3
They all go in descending order until the density slightly rises again beyond Saturn, but Earth sticks out like a sore thumb. For some reason, Earth is the densest planet, even beating the innermore Mercury and Venus.
This should be even more surprising when we think about the composition of Mercury. Mercury not only has no atmosphere, but it has a very, very thin mantle: one that makes up only about 15% of Mercury’s radius. Interior to that, Mercury is almost all metallic core, which makes up some 85% of its interior, by radius, and also explains why Mercury has an observed magnetic field. It’s as if not only was Mercury’s atmosphere stripped away, but also most of what would become its outer layers. And yet for Earth, where the core only makes up about 55% of our radius, and where we’re almost three times farther out from the Sun than Mercury, we’re the densest planet of all.
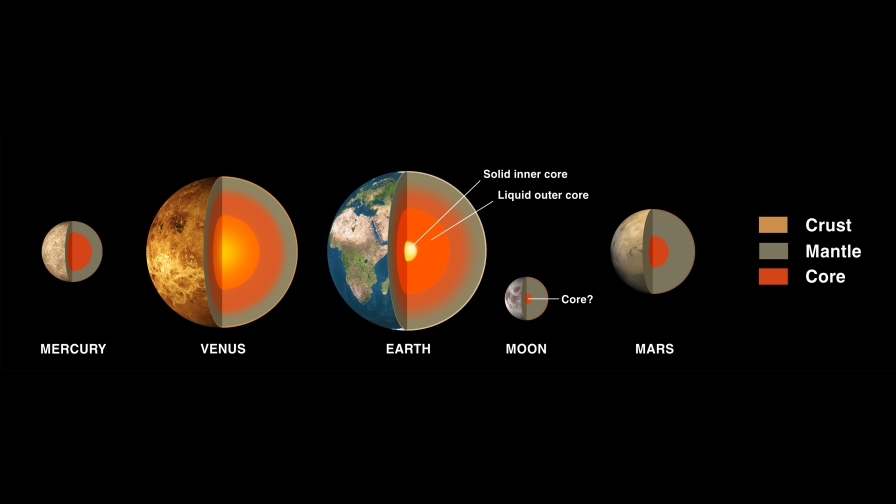
Credit: NASA/JPL
So, what’s the culprit?
Believe it or not, it’s simply due to gravity. Inside the Earth’s core, the cumulative gravitational force of everything surrounding it exerts a crushing pressure on the planet’s interior: about 3,600,000 times the pressure we experience at sea level and significantly less than any pressure experienced in Mercury’s interior. At these extreme pressures, atoms themselves start to change, as they become compressed to only a fraction of their normal, zero-pressure size. This factor, known as gravitational compression, is the key piece of the puzzle to understanding Earth’s remarkable density.
As it turns out — and this was worked out all the way back in the 1950s — a planet can’t be much bigger than Earth and still remain a rocky planet. Beyond a radius of 10,000 kilometers (and Earth is already pushing up against that with a radius exceeding 6,000 kilometers), a planet will actually start to contract as you add more and more mass. As the mass of your planet grows, the sizes of the central atoms shrinks faster than the additionally added atoms grow the overall size of the planet.
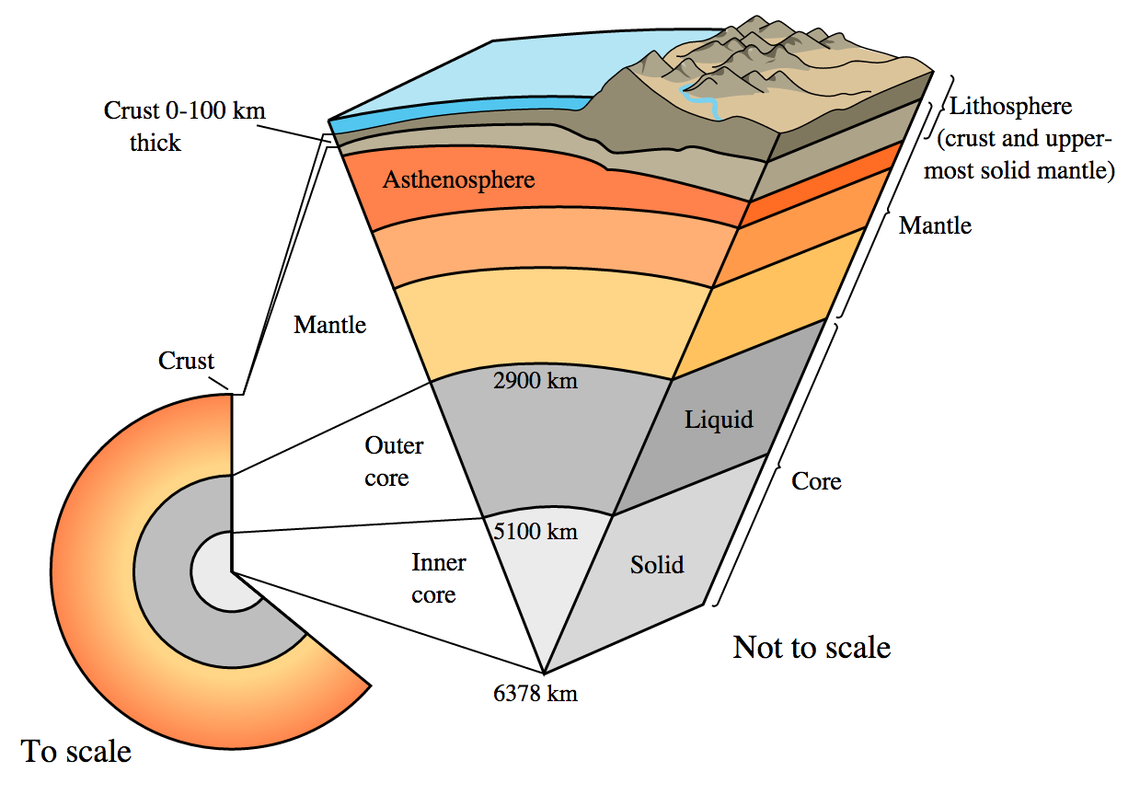
Credit: USGS
The effects of gravitational compression are vital in considering a planet’s density. Without them, Mercury would return to its position as the densest planet in the Solar System, defeating Earth and beating Venus by an even larger margin than it does now. Based on atomic composition alone, Mercury is made of denser, heavier atoms than any other planet in our Solar System. If we only looked at the atoms that they’re made of, the densities of Earth and Venus would be extremely close to one another, and our lack of understanding of Venus’s interior means that it remains possible that Venus — based solely on what it’s composed of — might even be made of heavier “stuff” than Earth is.
But Earth is massive: far more massive than Mercury and even more massive than Mercury, Venus, and Mars combined. That large amount of mass in one place is sufficient to start compressing the atoms in Earth’s interior, and particularly in Earth’s core, substantially, which is the decisive reason why Earth is the Solar System’s densest planet. If atoms had been truly and completely incompressible, Mercury would be the Solar System’s densest planet, and Earth’s density would only be comparable to that of Venus. Remarkably, even on planetary scales, the physics governing the humble atom is ultimately responsible for answering the question, “why is Earth, not Mercury, the densest planet of all?”