Black Holes Are Real And Spectacular, And So Are Their Event Horizons
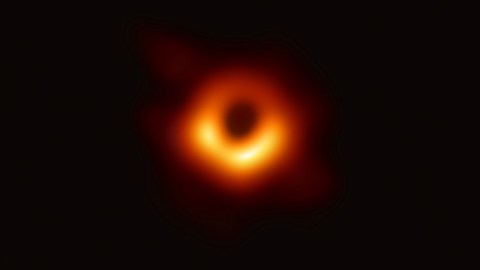
The first image of an Event Horizon is here. Here’s what it means.
Variety isn’t just the spice of life, but a natural consequence of living in our Universe. Gravitation, obeying the same universal laws on all scales, creates clumps and clusters of matter in an enormous suite of combinations, from tenuous clouds of gas to massive stars, all assembled into galaxies, clusters, and a great cosmic web.
From our perspective on Earth, there’s an enormous amount to observe. However, we can’t see it all. When the most massive of stars die, their corpses become black holes. With so much mass in such a small volume of space, nothing — no signals of any type — can get out. We can detect the matter and light emitted around these black holes, but within the event horizon, nothing escapes. In an incredible success story for science, we’ve just successfully imaged an event horizon for the first time. Here’s what we saw, how we did it, and what we’ve learned.
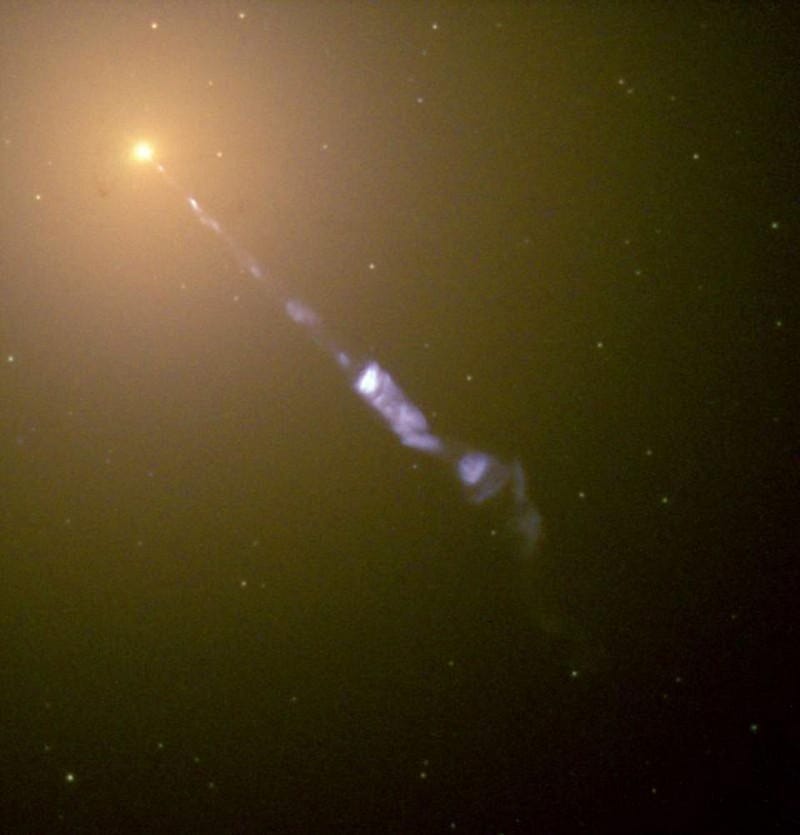
What did we see? What you see is dependent on where you look and how you do your observing. If we want to see an event horizon, our best bet was to look at the black hole that would appear largest from our perspective on Earth. That means it needs to have the greatest ratio of actual, physical size to its distance from us. While there may be as many as a billion black holes present in our own galaxy, the most massive one that we know of — by far — is located approximately 25,000 light-years away: in the center of the Milky Way galaxy.
This is the largest black hole, in terms of the angular size of its event horizon, visible from Earth, with an estimated mass of 4 million Suns. The second-largest is much more distant but much, much bigger: the black hole at the center of M87. This black hole is an estimated 60 million light-years distant, but weighs in at at estimated 6.6 billion Suns.
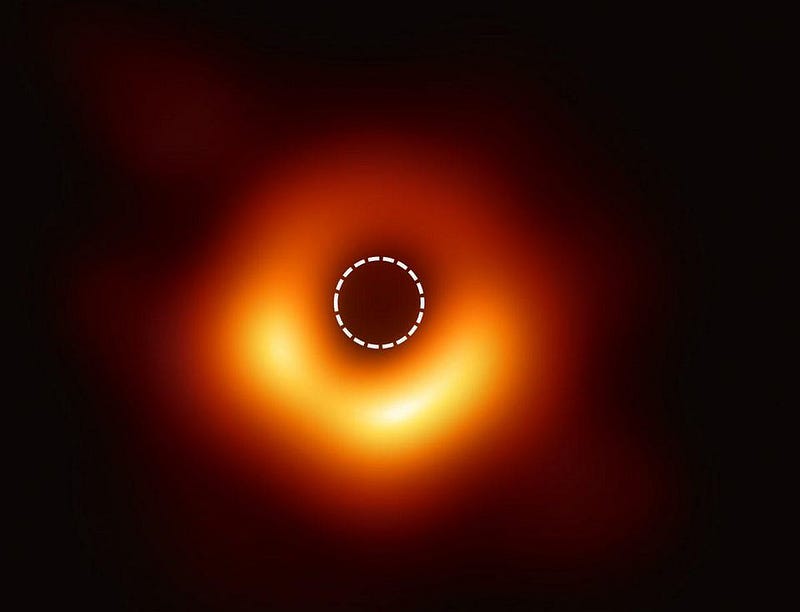
The Event Horizon Telescope attempted to image the event horizons of both of these, with mixed results. Originally estimated to be slightly larger than its M87 counterpart, the black hole at the center of the Milky Way — known as Sagittarius A* — has not yet had its event horizon imaged. When you observe the Universe, you don’t always get what you expect; sometimes, you get what it gives you. Instead, it was M87’s black hole that came through first, which was a much brighter and a much cleaner signal.
What we’ve found is spectacular. Those dark pixels at the center of the image are actually the silhouette of the event horizon itself. The light that we observe comes from the accelerated, heated matter around it, which must emit electromagnetic radiation. Where the matter exists, it emits radio waves, and the dark circle we see is where the background radio waves are blocked by the event horizon itself.
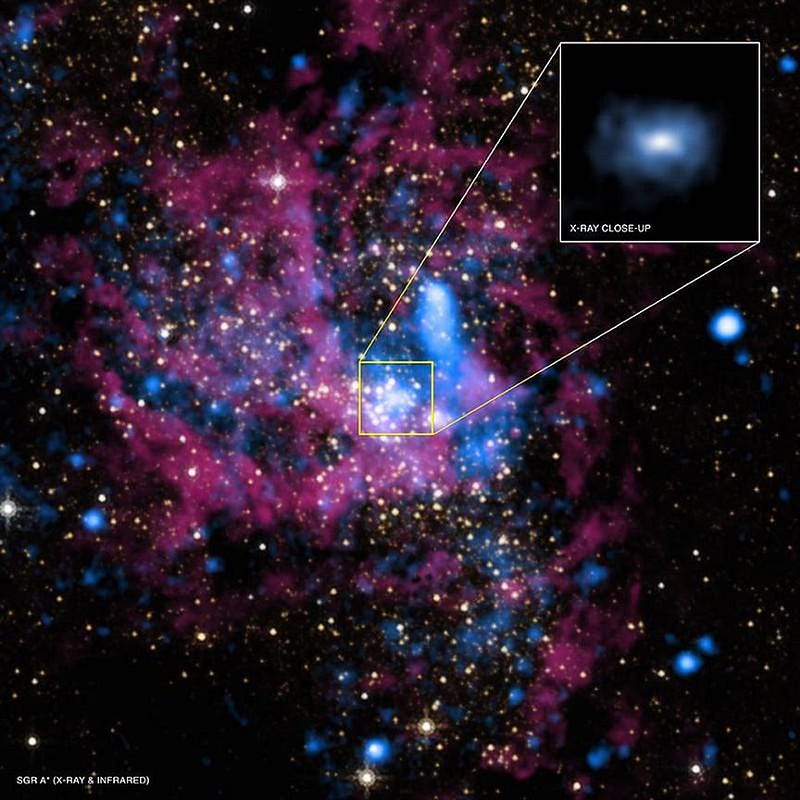
For M87, we saw everything we could’ve hoped for. But for Sagittarius A*, we didn’t quite get as lucky.
When you view a black hole, what you’re attempting to see is the background radio light surrounding the enormous mass at a galaxy’s center, where the event horizon of the black hole itself sits in the foreground of part of the light, revealing a silhouette. This requires three things to all line up in your favor:
- You have to have the right resolution, meaning that your telescope (or telescope array) needs to see the object you’re viewing as more than a single pixel.
- You need a galaxy that’s radio-loud, meaning that it emits a radio background that’s sufficiently strong to actually stand out against the silhouette of the event horizon.
- And you need a galaxy that’s radio-transparent, meaning that you can actually see all the way to the black hole without being confounded by foreground radio signals.
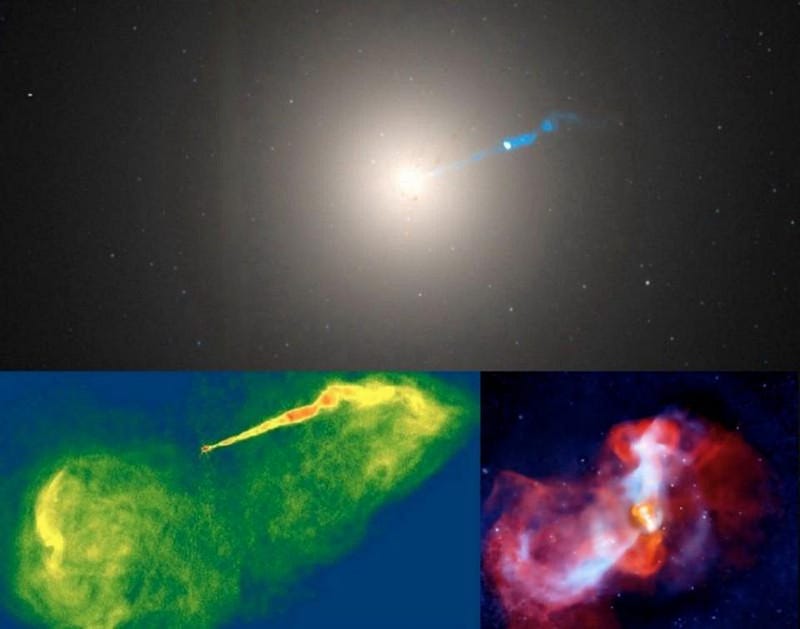
We’ve viewed extended emissions from around black holes many times in many wavelengths of light, including in the radio part of the spectrum. While M87 may meet all three of the necessary criteria, the black hole at the center of our own galaxy didn’t have a sufficient signal-to-noise ratio to create an image, possibly on account of the much lower levels of radiation intensity. Too bad, as we would’ve loved a better image of a second black hole, and the largest one, by angular size, in the Earth’s sky. We get the Universe we have, however, not the one we hope for.
The third-largest black hole as seen from Earth is at the center of the distant galaxy NGC 1277. While the Event Horizon Telescope has the right resolution to view it, it’s a radio-quiet galaxy, and so there isn’t enough radio background to see the sihouette. The fourth-largest black hole is close by, in the center of Andromeda, but our resolution, even with the Event Horizon Telescope, is too low to view it.
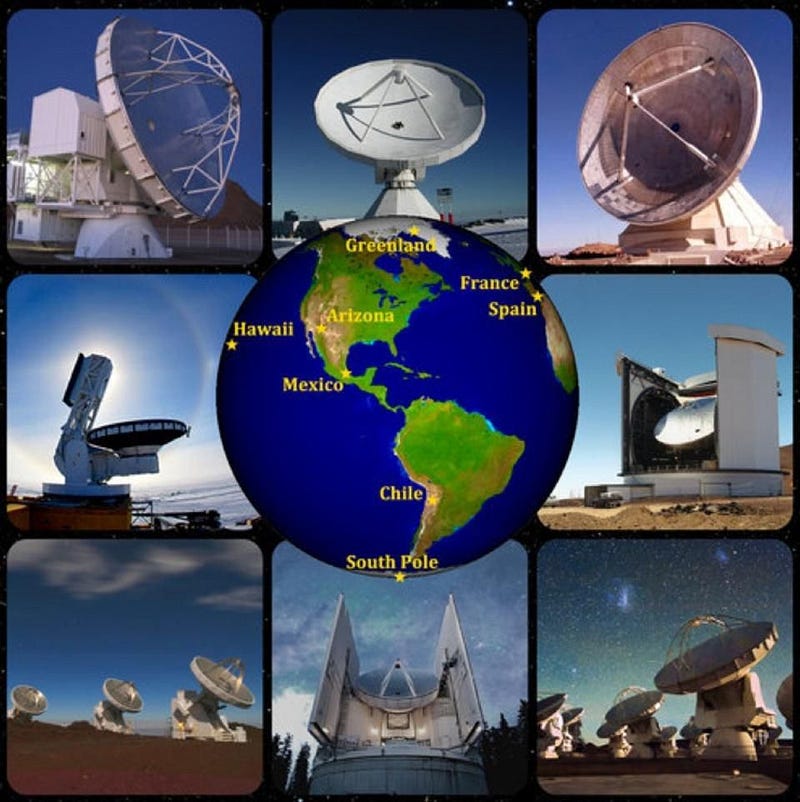
How did we see it? This is kind of the most remarkable part. The Event Horizon Telescope, like any telescope, needs two different aspects of the data it collects to cross a critical threshold.
- It needs to gather enough light to distinguish signal from noise, radio-loud regions from radio-quiet regions, and the region surrounding the black hole from the rest of the environment around the galactic center.
- It needs to achieve a sufficiently high resolution so that the precise details can be located at their correct angular position in space.
We need both of those in order to reconstruct any the details of any astronomical object, including a black hole. The Event Horizon Telescope had an enormous challenge to overcome in order to get an image of any black hole at all, owing to the tininess of its angular size.
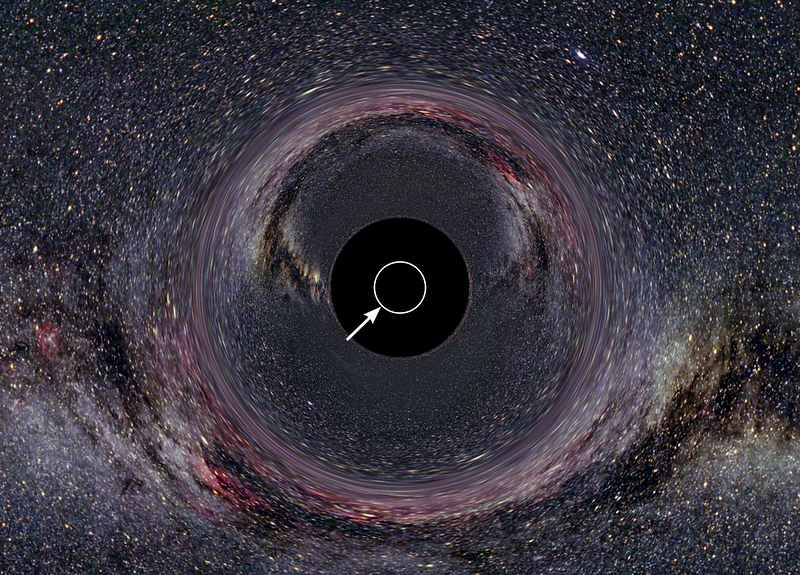
Because the regions around black holes are accelerated to such great speeds, the matter within them — made up of charged particles — generates strong magnetic fields. When a charged particle moves in a magnetic field, it emits radiation, and that’s where the radio signals come from. Even a modest-sized radio telescope, of only a few meters in diameter, is sufficient to pick the signal up. In terms of light-gathering power, observing the signal over the noise is pretty easy.
But the resolution is extremely challenging. It’s dependent on the number of wavelengths of light that can fit across your telescope’s diameter. To view the tiny black hole in our galaxy’s center, we’d need an optical telescope with a diameter of 5,000 meters; in the radio, where waves are much longer, we’d need a diameter of around 12,000,000 meters!
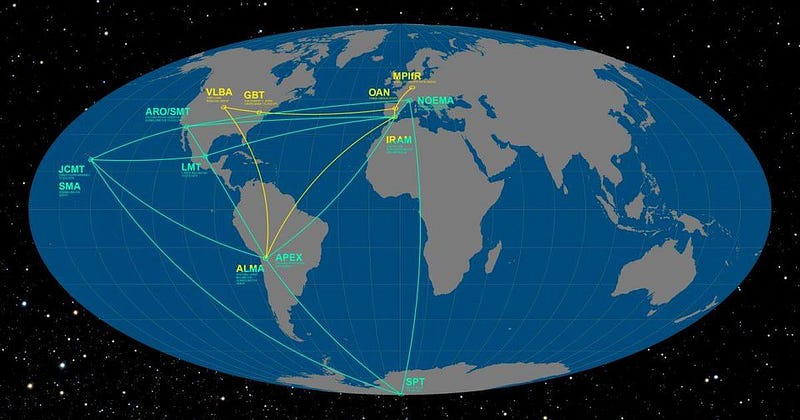
That’s why the Event Horizon Telescope is so powerful and clever. The technique it uses is known as Very Long Baseline Interferometry (VLBI), which basically takes two or more telescopes that can make the same types of observations from two distinct locations, and locks them together.
By taking simultaneous observations, you only get the light-gathering power of the individual dishes added together, but you get the resolution of the distance between the dishes. By spanning the diameter of Earth with many different telescopes (or telescope arrays) simultaneously, we were able to obtain the data necessary to resolve the event horizon.
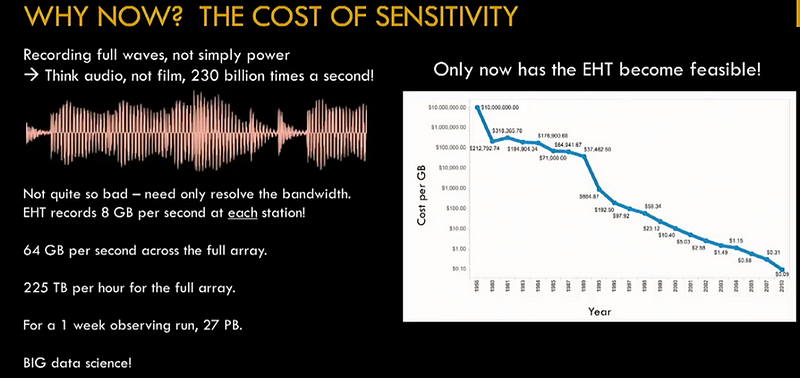
The data rates were incredible:
- It records a wave at a frequency that corresponds to 230 billion observations per second.
- That corresponds to 8 GB per second at each station.
- With 8 stations of telescopes/telescope arrays, an hour of continuous observations gets you 225 TB of data.
- For a 1 week observing run, that works out to 27 PB (petabytes) of data!
All for one single image of a black hole. After the data modules for M87 were brought together, it had 5 PB of raw data to work with!
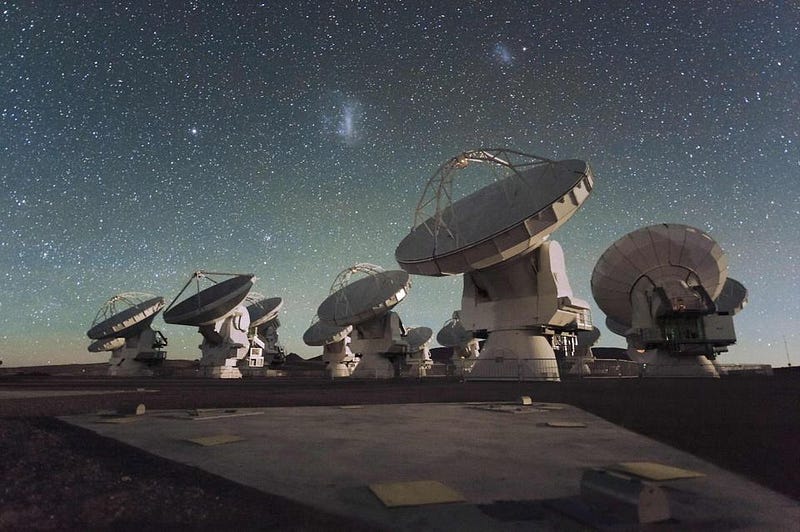
So, what did we learn? Well, there are a bunch of things we’ve learned, and there will be many stories about the different details and nuances that come out over the coming days and weeks. But there are four big takeaways that anyone should be able to appreciate.
First and most importantly, black holes really do exist! People have concocted all sorts of bizarre schemes and scenarios to avoid them, but the first direct image of an event horizon should put all of those doubts to rest. Not only do we have all the indirect evidence from LIGO, gravitational measurements of orbits around the galactic center, and the data from X-ray binaries, but we now have an image of the event horizon directly.
Second and almost as mind-blowing, General Relativity wins again! Einstein’s theory predicted that the event horizon would be spherical, rather than oblate or prolate, and that the region devoid of radiation would be of a particular size based on the measured mass of the black hole. The innermost stable circular orbit, predicted by General Relativity, shows the bright photons that are the last to escape from the black hole’s gravitational pull.
Once again, General Relativity, even when subject to a new test, emerged undefeated!
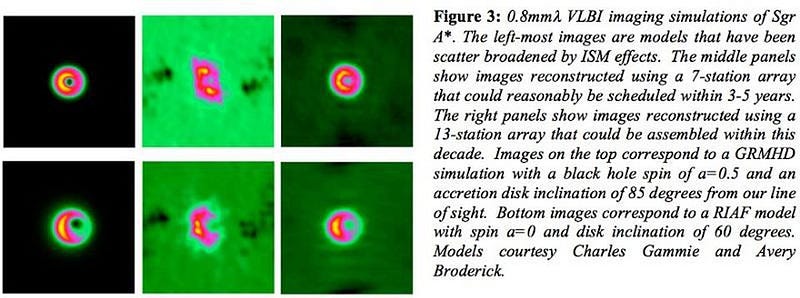
Third, we learned that our simulations for predicting what the radio emissions around the black hole should look like were really, really good! This tells us that we not only understand the environments around black holes very well, but that we understand the dynamics of matter and gas that orbit it. That’s a pretty spectacular achievement!
And fourth, we learned that the mass of the black hole that we inferred from gravitational observations are correct, and the mass of the black hole that we inferred from X-ray observations are systematically too low. For M87, these estimates differed by a factor of 2; for Sagittarius A*, they differed by a factor of 1.5.
We now know that gravity is the way to go, as the 6.6 billion solar mass estimates from M87’s gravity agree spectacularly with the Event Horizon Telescope’s 6.5 billion solar mass conclusion. Our X-ray observations, indeed, are biased towards values that are too low.
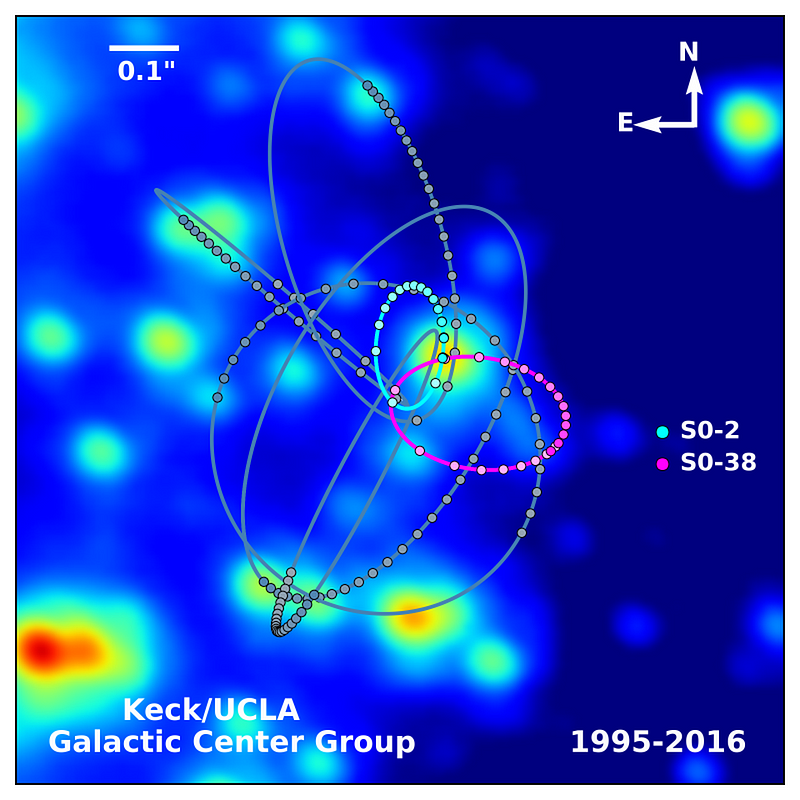
There will be more things to learn as we continue to do science with the Event Horizon Telescope. We can learn why black holes flare, and whether there are transient features that show up in the accretion disk, like hot blobs. We can learn whether the location of a central black hole moves over time, allowing us to infer the existence of smaller, hitherto invisible black holes near the supermassive, central ones. We can learn, as we gather more black holes, whether the masses we infer for black holes from either their gravitational effects or their X-ray emissions, are universally biased or not. And we can learn whether accretion disks have a universal alignment with their host galaxies or not.
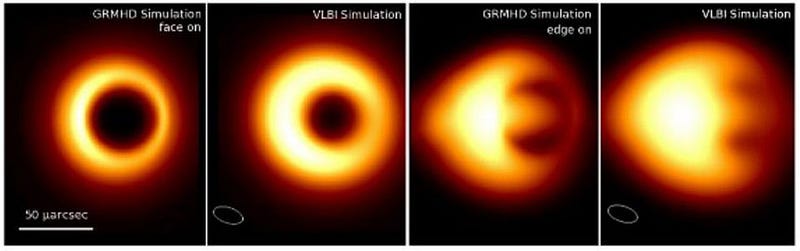
We cannot know these answers with just our first results, but this is just the start. We now live in a world where we can image the event horizons of black holes directly. We know that black holes exist; we know that event horizons are real; we know that Einstein’s theory of gravity has now been confirmed in an entirely unprecedented way. And any last lingering doubts that the supermassive behemoths at the centers of galaxies really are black holes has now evaporated.
Black holes are real, and they’re spectacular. At least in the radio part of the spectrum, thanks to the incredible achievement of the Event Horizon Telescope, we’re seeing them as never before.
Ethan Siegel is the author of Beyond the Galaxy and Treknology. You can pre-order his third book, currently in development: the Encyclopaedia Cosmologica.