Ask Ethan: How fast do gravitational waves travel?
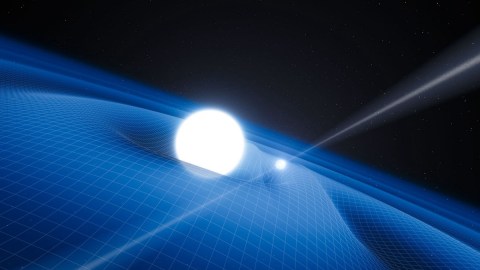
And if the Universe is expanding, does that mean these ripples can break the speed of light?
“Einstein’s gravitational theory, which is said to be the greatest single achievement of theoretical physics, resulted in beautiful relations connecting gravitational phenomena with the geometry of space; this was an exciting idea.” –Richard P. Feynman
One of the most surprising predictions of Einstein’s General Relativity is the existence of not just matter, radiation and other particle-based forms of energy, but the existence of gravitational radiation itself, or fundamental “ripples” in the very fabric of spacetime. This is one of the toughest things to understand, and Patreon supporter Robert J. Hansen wants to know more:
[Gravitational] waves are perturbations of spacetime which travel at c. However, spacetime is allowed to expand and contract faster than c. An expansion followed by a compression is pretty much the definition of a compression wave. This appears to produce a paradox: gravity waves travel at c, but there appears to be a way for them to be superluminal. What’s the resolution of this apparent paradox?
First off, let’s start with the concept of this radiation (and how it’s produced) itself.
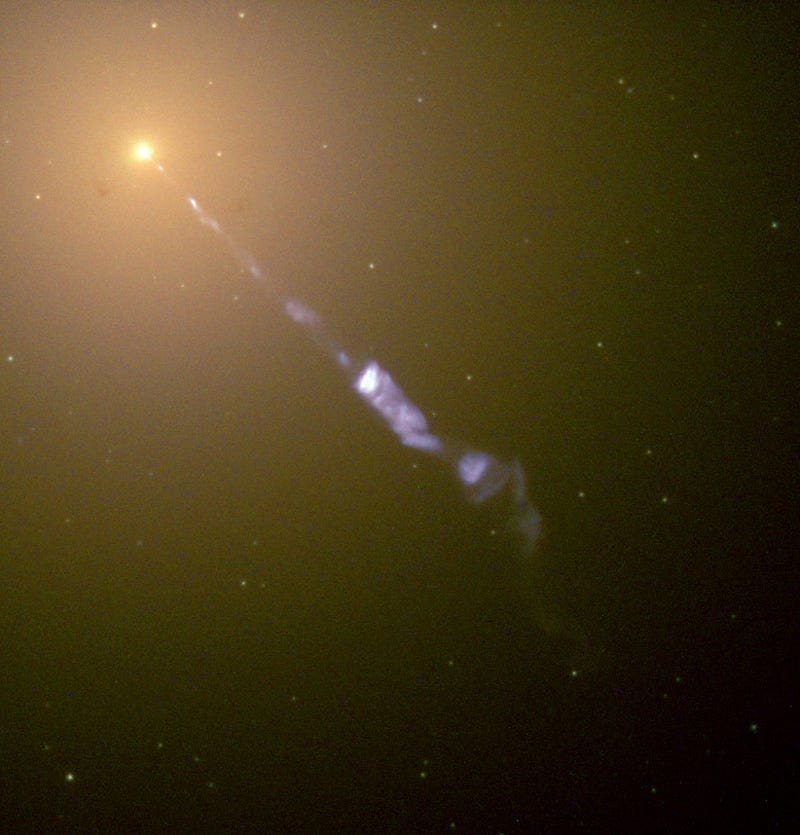
In electromagnetism — even in classical electromagnetism — there are only two things you need in order to produce electromagnetic radiation: a chargeand a field for it to move through. An electric charge can be positive (like a proton) or negative (like an electron), and if it passes through a magnetic field, that field is going to accelerate that charge, causing it to move in a circular or helical path from hereon out.
The bigger the field, the bigger the speed and the bigger the charge-to-mass ratio of the particle, the greater the acceleration (or change in motion) will be.
But interactions like this need to conserve both energy and momentum, and the way that plays out in electromagnetism is that any time a charge accelerates due to an external field, it has to emit radiation in order to do so. This radiation (in electromagnetism) comes in the form of photons, and is called Bremsstrahlung, Cyclotron or Synchrotron radiation, dependent on how it’s created.
In Newtonian physics, there’d be no such thing as gravitational radiation, but Einstein’s General Relativity changed all of that. Massive sources — things like particles — have the analogue of a gravitational charge, while the curved fabric of space itself is the analogue of a gravitational field. Any time a massive particle moves through curved space, which can be severely curved in the presence of a star, white dwarf, neutron star or black hole, it will emit the analogue of electromagnetic radiation: gravitational radiation.
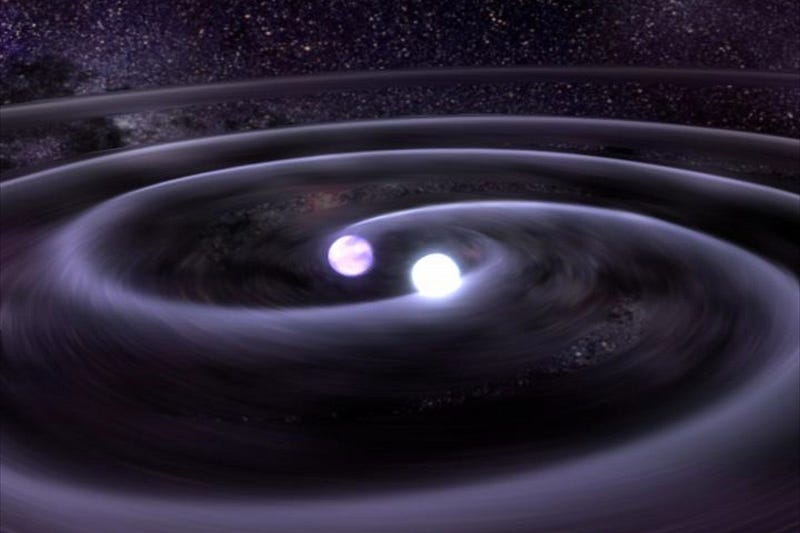
This new form of radiation is neither a photon nor any other form of particulate radiation, but is a ripple through the fabric of space itself: a gravitational wave. For a mass like the Earth orbiting the Sun, gravitational radiation is so small it would take some 10¹⁴⁰ ages of the Universe for the orbit to change in a noticeable fashion; we’ll never see it. But for systems where the masses are larger, the distances are closer and the fields are stronger, the consequences are more severe: systems like binary pulsars, something orbiting the supermassive black hole at our galaxy’s center or even merging black holes. In these cases, we can observe orbital decay, and in order to conserve energy, we know that something must be carrying it away.
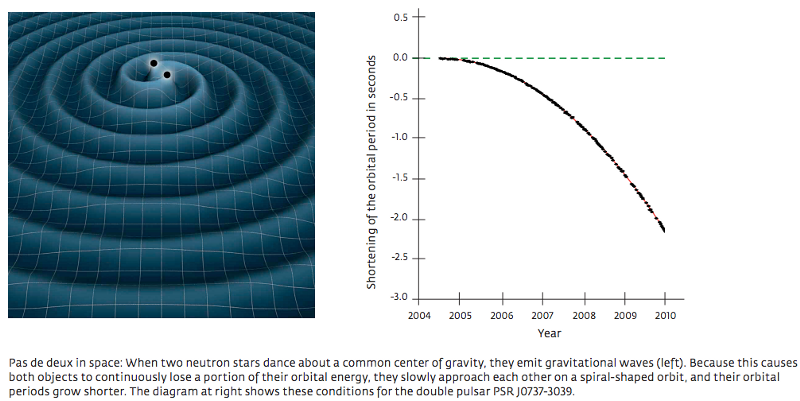
That “thing” must be gravitational radiation (a.k.a. gravitational waves), and thanks to observations of binary pulsar systems, we know that the speed of this gravitational radiation must be equal to the speed of light to a precision of just 0.2%! In other words, the ripples do, in fact, move through space at the same speed that photons do. The major difference is that, in the case of gravitational radiation, these are ripples inherent to the fabric of space itself.
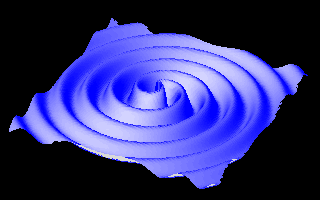
So what happens, then, to get back to Robert’s original question, when these ripples get created not in (approximately) static space, but in the expanding Universe? The answer is that they get stretched and subjected to the Universe’s expansion the exact same way photons do.
When photons propagate through the expanding Universe, their wavelength stretches as the fabric of space expands. Their number (and energy) density dilutes, and although they always propagate at the speed of light, the distances between the emitted source and the observing receiver changes. For example, at the very start of the hot Big Bang, some 13.8 billion years ago and just 10^-33 seconds after the end of inflation:
- A photon that’s reaching us today would have been just 100 meters away from us 13.8 billion years ago.
- That photon would have traveled for 13.8 billion years, traveling 13.8 billion light years through the expanding Universe, and would have had its wavelength stretched by approximately 28 orders of magnitude.
- And upon reaching us today, the location where that photon was emitted from would today be 46.1 billion light years distant from us.
Sounds crazy? Well, the exact same craziness happens to gravitational waves! A gravitational ripple must also travel through the expanding Universe, will also travel at the speed of light through space (whether that space is expanding, contracting or static), and will have its wavelength stretched the exact same way photons have theirs stretched. Gravitational waves “ride” the fabric of space the same way water waves “ride” the surface of the water; if you have a rock fall into a river, the ripples don’t just move radially outward; they move outward and get carried by the current downstream.
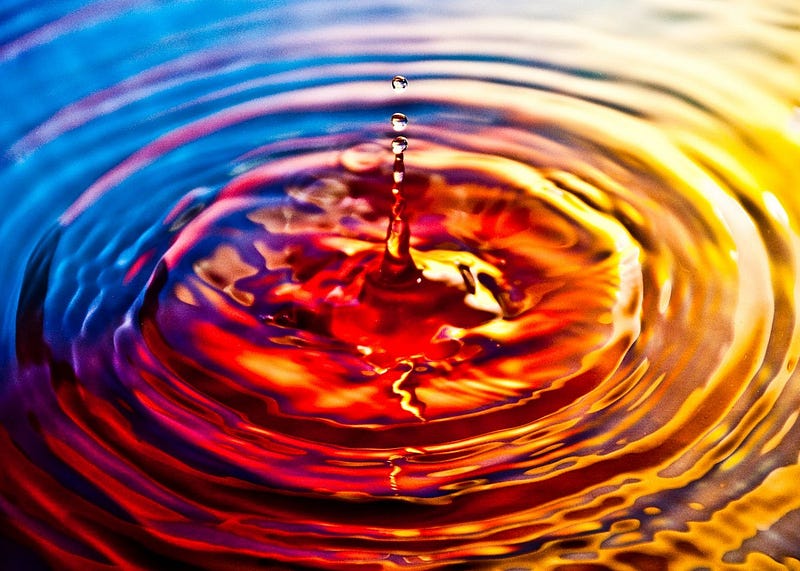
The gravitational ripples in the fabric of space are a little bit like that: the ripples move at the speed they always move at through the medium — at the speed of light, c — but sometimes the medium itself moves. That doesn’t mean it’s breaking the speed of light any more than photons do when they wind up 46 billion light years from where they started after just 13.8 billion years; gravitational waves are doing exactly what they’re supposed to do. The analogy of a compression followed by a rarefaction is actually a very, very good one, mind you; a passing wave will distort the fabric of space (and all the items/particles in it) by stretching and compressing them in a very particular fashion.
But the way that propagates through the Universe is at the speed of light on top of whatever the fabric of space itself is doing: expanding, contracting or remaining static. And that’s the resolution of the paradox: they travel at c, no matter what you do to the fabric that they travel through while they’re en route!
Leave your comments on our forum, and check out our first book: Beyond The Galaxy, available now, as well as our reward-rich Patreon campaign!