Ask Ethan: How does dark matter interact with black holes?
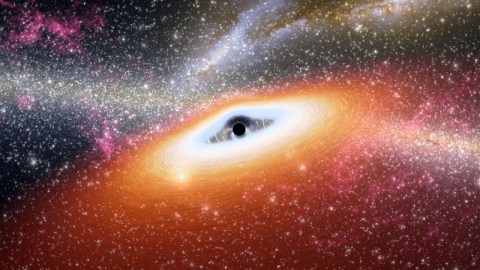
If black holes suck everything in and nothing can get out, what does that mean for dark matter?
“A single day is enough to make us a little larger or, another time, a little smaller.” –Paul Klee
Black holes are some of the most extreme objects in the Universe: a concentration of mass so great that it collapses, under General Relativity, into a singularity at its center. Atoms, nuclei, and even fundamental particles themselves are crushed down to an arbitrarily small thickness in our three-dimensional space. At the same time, everything that falls into it is doomed to never escape, but simply to add to its gravitational pull. What does that mean for dark matter? Our Patreon supporter kilobug wants to know:
How does dark matter interact with black holes? Does it get sucked into the singularity like normal matter, contributing to the mass of the black hole? If so, when the black hole evaporates through Hawking radiation, what happens to [it]?
This is a great question, and it all starts with what black holes actually are.
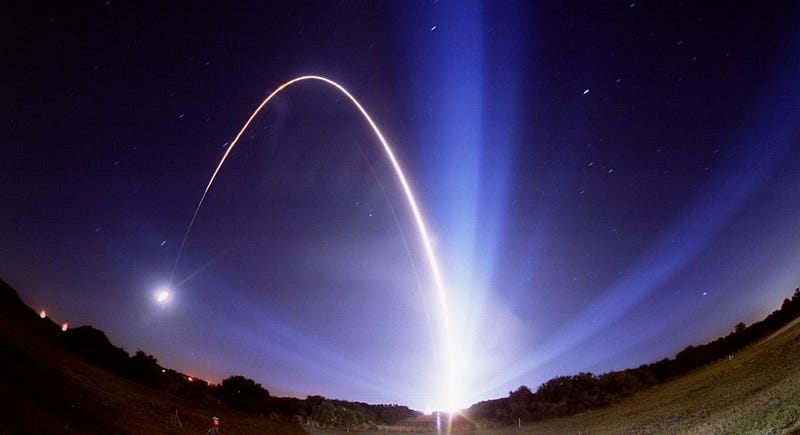
Here on Earth, if you want to send something into space, you need to overcome the Earth’s gravitational pull. For our planet, what we call “escape velocity” is somewhere around 25,000 mph (or 11.2 km/s), which we can achieve with powerful rocket launches. If we were instead on the surface of the Sun, the escape velocity would be much greater: about 55 times as great, or 617.5 km/s. When our Sun dies, it will contract down to a white dwarf, of about 50% the Sun’s current mass but only the physical size of Earth. In this case, its escape velocity will be about 4570 km/s, or about 1.5% the speed of light.
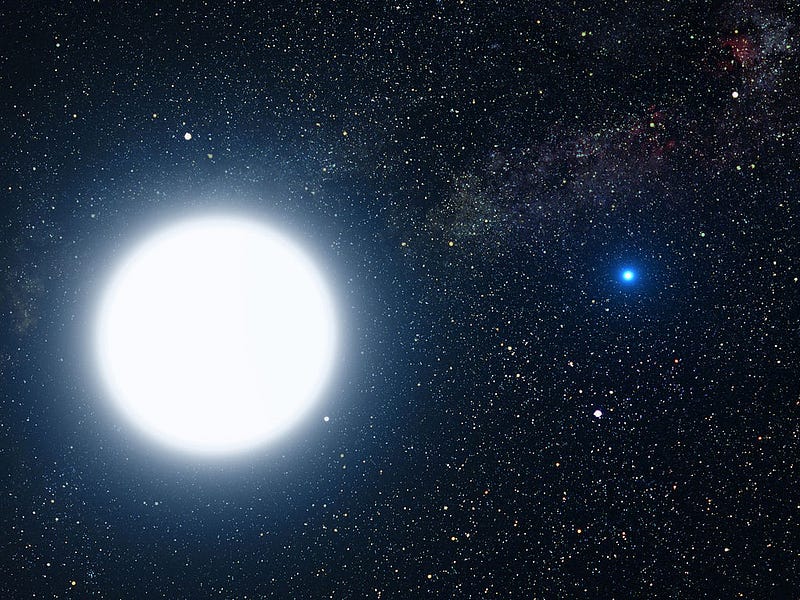
This is important, because as you concentrate more and more mass into a particular region of space, the speed required to escape this object gets closer and closer to the speed of light. And once your escape velocity at the object’s surface reaches or exceeds the speed of light, it isn’t just that light can’t get out, it’s required that — at least as we understand matter, energy, space and time today — everything within that object collapse down to a singularity. The reason is simple: all the fundamental forces, including the forces that hold atoms, protons, or even quarks together, can move no faster than the speed of light. So if you’re at any point away from a central singularity and you’re trying to hold a more distant object up against gravitational collapse, you can’t do it; collapse is inevitable. And all you need to crest past this limit in the first place is a star more massive than about 20–40 times the mass of our Sun.
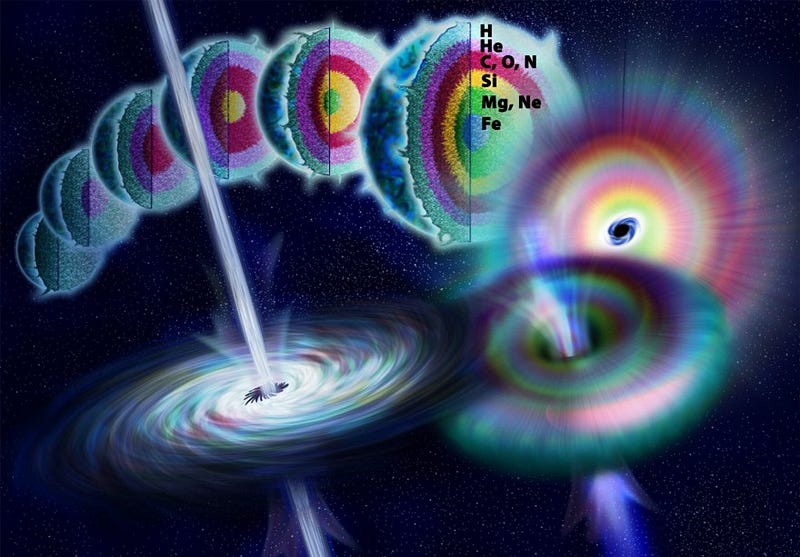
When it runs out of fuel in its core, the center will implode under its own gravity, creating a catastrophic supernovae, blowing off and destroying the outer layers but leaving a black hole at the center. These “stellar mass” black holes, somewhere in the neighborhood of 10 solar masses, will grow over time, consuming any matter or energy that dares to venture too close to it. Even if you move at the speed of light when you fall in, you’ll never get out again. Due to the extreme curvature of space inside, you’ll inevitably encounter the singularity at the center. When that happens, all you do is add to the energy of the black hole.
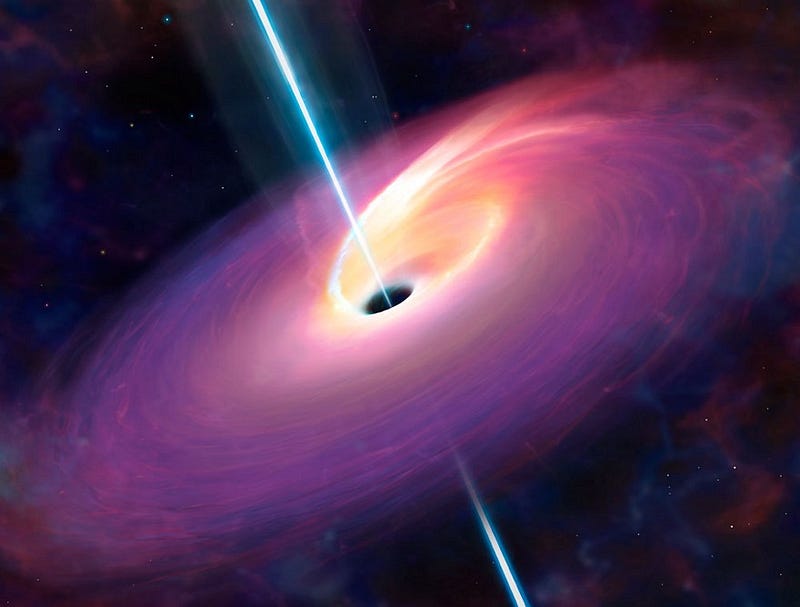
From the outside, we can’t tell whether a black hole was initially made up of protons and electrons, neutrons, dark matter or even antimatter. There are — as far as we can tell — only three properties that we can observe about a black hole from outside of it: its mass, its electric charge and its angular momentum, which is a measure of how fast it’s spinning. Dark matter, as far as we know, has no electric charge, nor does it have any of the other quantum numbers (color charge, baryon number, lepton number, lepton family number, etc.) that may or may not be conserved or destroyed as pertains to the black hole information paradox.
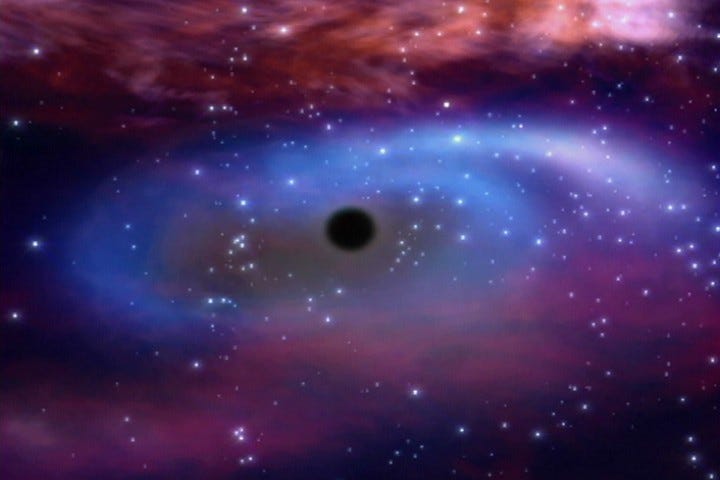
Because of how black holes are formed (from the explosions of supermassive stars), when they’re first formed, black holes are pretty much 100% normal (baryonic) matter, and just about 0% dark matter. Remember that dark matter interacts only gravitationally, unlike normal matter, which interacts via the gravitational, weak, electromagnetic and strong forces. Yes, there’s perhaps five times as much dark matter total in large galaxies and clusters as there is normal matter, but that’s summed up over the entire huge halo. In a typical galaxy, that dark matter halo extends for a few million light years, spherically, in all directions, while the normal matter is concentrated in a disk that’s just 0.01% the dark matter’s volume.
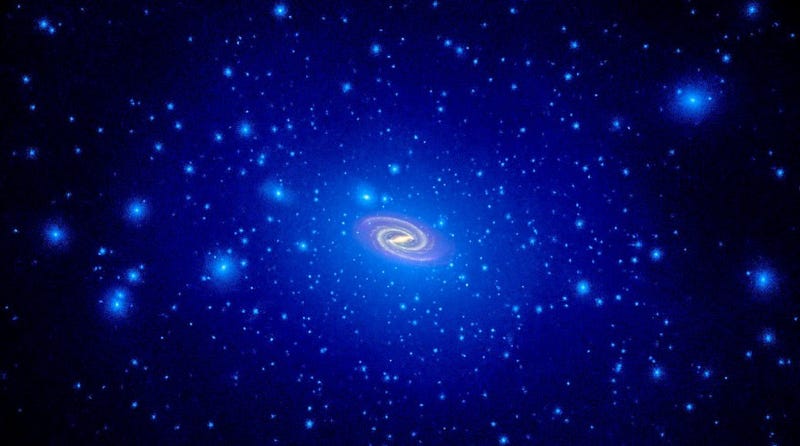
Black holes tend to form in the innards of the galaxy, where the normal matter totally dominates over dark matter. Consider just the region of space where we’re located: around our Sun. If we drew a sphere that was 100 AU in radius (where one AU is the distance of the Earth from the Sun) around our Solar System, we’d enclose all the planets, moons, asteroids and pretty much the entire Kuiper belt, but the baryonic mass — the normal matter — of what would be inside our sphere would be dominated by our Sun, and would weigh about 2 × 10³⁰ kg. On the other hand, the total amount of dark matter in that same sphere? Only about 1 × 10¹⁹ kg, or just 0.0000000005% the mass of the normal matter in that same region, or about the mass of a modest asteroid the size of Juno, approximately 200 km across.
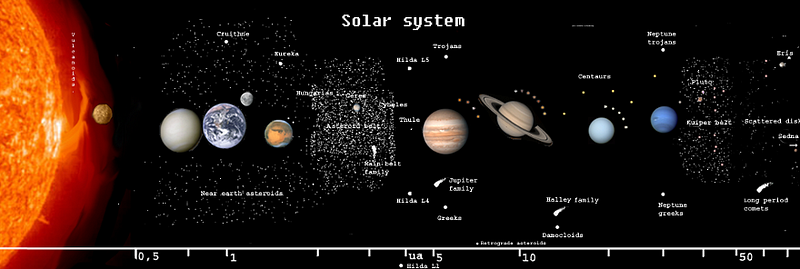
Over time, dark matter and normal matter both will collide with this black hole, getting absorbed and adding to its mass. The vast majority of black hole mass growth will come from normal matter and not dark matter, although at some point, many quadrillion years into the future, the rate of black hole decay will finally surpass the rate of black hole growth. The Hawking radiation process results in the emission of particles and photons from outside the black hole’s event horizon, conserving all the energy, charge and angular momentum from the black hole’s insides. This process may take anywhere from 10⁶⁷ years (for a solar mass black hole) to 10¹⁰⁰ years (for the most massive multi-billion solar mass black holes), but eventually what comes out is a mix of everything that’s possible.
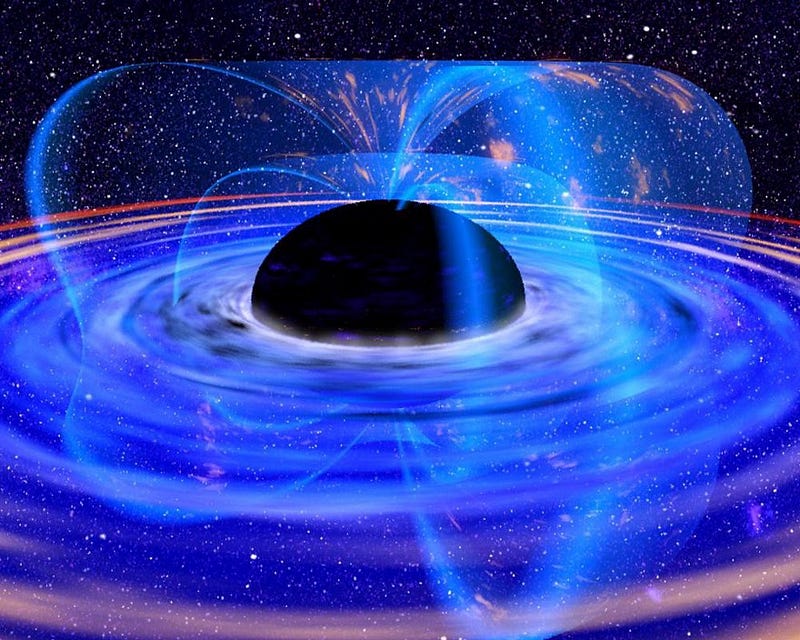
This means that some dark matter will come out of black holes, but that’s expected to be completely independent of whether a substantial amount of dark matter went into the black hole in the first place. All a black hole has memory of, once things have fallen in, is a small set of quantum numbers, and the amount of dark matter that went into it isn’t one of them. What comes out isn’t going to be the same as what you put in!
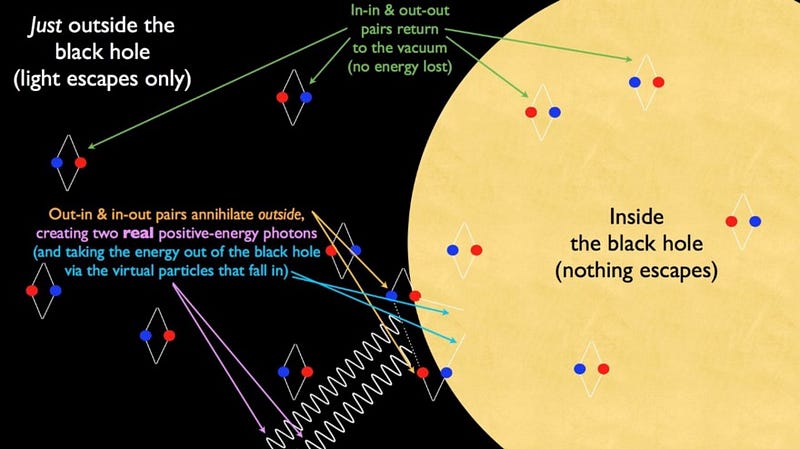
So at the end of the day, dark matter is just another food source for black holes, and not a very good one at that. Even worse: it’s not even an interesting source of food. What black hole “sees” is no different than shining a flashlight into a black hole and having your photons absorbed until, via E=mc^2, you’ve put in as much energy as there is mass in the dark matter that fell in. No other types of charge exist in dark matter, and other than the angular momentum from falling in off-center (which applies to photons, too), there’s no other effect on black holes at all, either going in or coming out.
Have a question you want featured on the next Ask Ethan? Send it in to startswithabang at gmail dot com!
This post first appeared at Forbes. Leave your comments on our forum, check out our first book: Beyond The Galaxy, and support our Patreon campaign!