Ask Ethan: Could We Be Living In A Steady-State Universe?
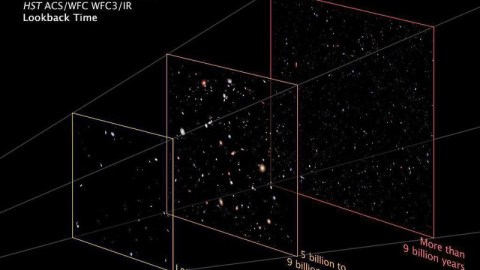
The Big Bang is our accepted origin of the Universe. But is there another possibility?
Since the mid-1960s and the discovery of the Cosmic Microwave Background, the Big Bang has stood alone, largely unchallenged, as the leading theory of our cosmic origins. Our Universe, at least the Universe as we observe it, started off in a hot, dense, mostly uniform state some 13.8 billion years ago, and has expanded, cooled, and gravitated ever since, giving rise to the star-and-galaxy-rich cosmos we see today. But the Big Bang didn’t emerge as our consensus position because we cannot consider any alternatives, but rather because every serious alternative that makes quantitative predictions fails to reproduce the Universe we have. Even the Big Bang’s most enduring competitor, the Steady-State theory, can’t replicate the Big Bang’s observational successes, despite an enormous, herculean effort by some of the most brilliant minds in history. Pbellas123 writes in to simply ask the following:
“Has the steady-state theory been disproven?”
In science, we don’t really prove or disprove hypotheses, but the data can either validate or refute the predictions of any particular hypothesis. In the case of the Steady-State theory, it yields at least four meaningful predictions that conflict with the data we have. This might serve as a practical refutation of the Steady-State theory’s central ideas, but it’s even more valuable as an illustration of how science successfully works. Let’s examine the evidence for ourselves.
Back in the 1920s, the first major clues toward the nature of our Universe’s origins — both from the observational and theoretical perspectives — were revealed to humanity. On the theoretical side, Alexander Friedmann first derived the most important solution to general relativity in all of modern cosmology: how a Universe filled with whatever ingredients you can imagine, uniformly, will evolve with time. Anything you can dream up, including exotic ingredients that Friedmann himself never imagined:
- normal matter,
- dark matter,
- black holes,
- neutrinos,
- a cosmological constant,
- dark energy,
- cosmic strings,
- spatial curvature,
- domain walls,
- magnetic monopoles,
- radiation,
and a whole lot more are all described by the same equations, known today as the Friedmann equations. These were derived all the way back in 1922, and they came to a startling conclusion: that if your Universe is filled with the same type and amount of stuff, everywhere and in all directions, on average, then it cannot be static, and will either expand or contract. A Universe that is evenly filled with stuff everywhere you look cannot be unchanging.
The very next year, 1923, marked the year that Edwin Hubble’s critical observations came in. By identifying a specific class of star in the Andromeda Nebula, he established the distance to that object, showing that it was far, far outside our own galaxy. Over the next few years, Hubble found that exact same type of star in many other spirals in the sky, establishing their distance from us and discovering along the way that the farther away a galaxy was from us, on average, the faster it appeared to recede from us. By the late 1920s, the notion of the expanding Universe began to be rapidly accepted.
In 1927, Georges Lemaître put the theory and the observations together for the first time, deriving what we now know as Hubble’s Law. In 1928, Howard Robertson did the same thing independently, but it was Edwin Hubble’s 1929 paper, which had much more and more comprehensive data than any of the prior analyses, that put all of the pieces together and reached the wider community. It became, in short order, very clear that the Universe was large, full of galaxies, and expanding. In many ways, this marked the birth of modern cosmology.
If the Universe was expanding, though, then what did that mean for our origin and our fate? Where did the Universe come from, how did it get to be the way we see it today, and where is it heading in the future? There were many possible answers with just this one piece of evidence — the expanding Universe — even taking the assumption that Einstein’s general relativity was our correct theory of gravity.
The most famous example today is the Big Bang, which hypothesized that the reason we see the Universe as large, clumpy, and expanding today is because it was smaller, hotter, and denser in the past. As time has gone on, the Universe expands, gravitates, and cools, giving rise to the Universe as we see it today. If we look earlier, it’s more uniform and hotter, which means that:
- galaxies should evolve, being smaller, intrinsically bluer, lower in heavy elements, and full of younger stellar populations the earlier we look,
- there should be a leftover “bath” of radiation, redshifted to just a few degrees above absolute zero by today, that was released when the Universe cooled enough to allow the formation of neutral atoms without immediately reionizing them,
- and there should be light elements — hydrogen, helium, and their various isotopes — produced in the earliest stages of the hot Big Bang.
In combination with the already-observed Hubble expansion, these four total criteria are the cornerstones of the Big Bang, and they’re all testable observationally.
On the other hand, there were many alternative theories floating around in these early days of cosmology, as there were so few constraints that many of them seemed viable. Perhaps general relativity wasn’t our correct theory of gravity, and something like the Milne Universe would be correct. Perhaps our light just got tired during this cosmic journey, and it appeared to be redshifted due to this factor, not due to cosmological expansion. Perhaps the Universe was an oscillating plasma. Perhaps there was a large rotational motion to the Universe, in addition to this expansion motion we observe.
But the most popular alternative is today known as the Steady-State theory. It was founded on what’s now known as the perfect cosmological principle, which hypothesized that the Universe wasn’t just the same, on average, everywhere in space, but also in time. That no matter when you looked at the Universe, on average, you’d always see the same thing. This is the core tenet of the Steady-State theory: that the Universe isn’t just the same everywhere, but also “everywhen.” The Steady-State Universe isn’t just eternal, but timeless.
That seems difficult to do in a Universe that’s full of stars, because stars burn based on the fuel inside them, and that fuel runs out. It seems difficult to do in a Universe that’s expanding, because the matter in it will dilute over time and become less dense, which means we expect the number of galaxies per-unit-volume to evolve over time. But the Steady-State theory had — depending on your perspective — either a brilliant fix or a catastrophic cop-out: it hypothesized that, as the Universe expands, new particles like protons and electrons get created. This matter-creation field, its proponents argued, would replenish the Universe as it expanded, allowing for it to appear timeless.
In the 1950s, the Steady-State model’s proponents derided the Big Bang as a religious idea, not a scientific theory. The name itself, “Big Bang,” derived from Steady-State proponent Fred Hoyle’s derogatory remarks about the hypothesis on BBC radio, while Big Bang proponent George Gamow delighted in how easy it was to provoke his scientific antagonists. All of this wasn’t decided, as we commonly tell the tale today, with the discovery of the predicted low-temperature background of radiation: the Cosmic Microwave Background. Rather, it was four subsequent, more detailed observations that have ruled out the Steady-State model as a viable alternative today.
1.) The measurement of the spectrum of the Cosmic Microwave Background. If the Big Bang is correct, the “leftover bath of radiation” should have a cosmic origin and be a perfect blackbody in its spectrum, following a particular energy distribution. If the Steady-State theory is correct, there may also be an omnidirectional bath of radiation: from starlight absorbed and re-radiated by dust. But this is good! The two background would be similar, but measurably different.
The reason is that the early Universe, under the assumptions of the hot Big Bang, will be a single perfect blackbody. But the Sun, like all stars, is actually a series of blackbodies at different temperatures, as the “photosphere” of any star is actually the final few dozen kilometers beneath its surface. Since the 1990s, our instruments have been good enough to tell the difference between these two scenarios, and the radiation spectrum shows it’s a single blackbody, not the sum of a series of many blackbodies. The Big Bang gets validated; the Steady-State theory gets busted.
2.) The observation that the Universe really was hotter in the distant past. This is a brilliant case of where we became able to measure something we had no idea how to measure initially: what the temperature of this leftover radiation background was not only today, but at different points throughout the Universe’s history. If the Steady-State theory were correct, the temperature of this background should be independent of time and redshift, but if the Big Bang were correct, the temperature should rise linearly with redshift: proportional to the quantity (1+z), where z is the observed redshift.
By looking at how radiation interacts with matter at a variety of redshifts, we can actually measure what the temperature of this background of radiation must have been at various distances and redshifts. As you can see, above, there’s not only a clear rise, but the observed rise (blue points, green points, and red points, all with error bars) follows the black dotted line very well: the exact predictions of the Big Bang. This direct measurement again agrees with the Big Bang and contradicts the Steady-State model.
3.) The discovery that galaxies and galaxy densities in the Universe really do evolve over time. With the advent of modern telescopes, we can look at the Universe and find galaxies not only millions, but billions or even tens of billions of light-years away. When we do, we find two pieces of evidence that enable us to discern between the Big Bang and the Steady-State theories: the number density of galaxies and the observed properties of the galaxies themselves.
If the Steady-State theory is correct, both of these properties should be identical today to what they are at great distances: the Universe should be uniform in both space and time. But if the Big Bang is correct, there should be greater numbers of galaxies-per-unit-volume in the past, as the Universe is predicted to have been denser, and those early galaxies should be smaller, bluer, and lower in heavy elements.
The Big Bang’s predictions are precisely what we observe, contradicting what the Steady-State model predicts and putting further nails into its coffin.
4.) The discovery that even in the most pristine populations of gas, there are still elements other than hydrogen. This is another very big one: if the Steady-State theory is correct, and matter — in the form of protons and electrons — is constantly being created in the spaces between galaxies, we should observe populations of gas that are made of pristine hydrogen and nothing else. However, if the Big Bang is correct, then there was a very hot and dense origin to the Universe, and there should have been a period where nuclear fusion occurred very early on.
That means that any gas we find, even if it’s never formed stars before, should still have not just plain old hydrogen with one proton and one electron, but deuterium, helium-3, helium-4, and a tiny bit of lithium-7. In 2011, we found the first “pristine” populations of gas, and they still were made of about ~25% helium (by mass). In addition, even the most metal-poor (with the fewest heavy elements, and hence the smallest amount of star-formation history) galaxies and gas clouds ever seen still have helium and deuterium, and lithium (where we can measure it). Again, the Big Bang’s predictions match our observations, and the Steady-State theory gives answers that conflict with what we observe.
You might ask yourself, quite reasonably, “Well, if this is what the evidence indicates, then surely everyone who didn’t accept the Big Bang in the 1960s had changed their tune by the late 1990s and beyond, right?”
If only.
Fred Hoyle, Thomas Gold, Hermann Bondi, Geoffrey Burbidge, and many other Steady-State theorists — including the academic descendants of these influential pioneers — continued to move the goalposts and come up with excuses and mental gymnastics to avoid the only acceptable conclusion: the evidence supports the Big Bang and not the Steady-State model. Yet they never reached that point, coming up with quasi-Steady-State models, deriding the existence of a “mysterious cosmic fog” (the Cosmic Microwave Background), and publishing useless paper after useless paper accusing their peers of groupthink and decrying the lack of good alternatives.
From 2001 to 2010, those four men, all clinging to their antiquated ideas of what science ought to be rather than what it was, all passed away. Of the quasi-Steady-State advocates of note, only Jayant Narlikar remains; of the arguments in support of it and against the Big Bang, there have been none of note for many years. The Steady-State theory has been condemned not by groupthink, but by the evidence. If anyone tells you differently, you now know exactly how to test it for yourself. Human beings may lie, but the Universe itself, if you ask it the right questions about itself, never will.
Send in your Ask Ethan questions to startswithabang at gmail dot com!
Starts With A Bang is written by Ethan Siegel, Ph.D., author of Beyond The Galaxy, and Treknology: The Science of Star Trek from Tricorders to Warp Drive.