Ask Ethan #79: The tiniest Neutron Star
What would happen if you pulled a tiny chunk out of a neutron star?
“Try to imagine what it will be like to go to sleep and never wake up… now try to imagine what it was like to wake up having never gone to sleep.” –Alan Watts
Sometimes, the most fun experiments in physics are the ones you can perform only in your head. Despite our physical limitations of not being able to actually go to, dissect, and study in gory detail any object in the Universe we want, our understanding of matter — in all its forms — and the laws that govern it get us awfully far.
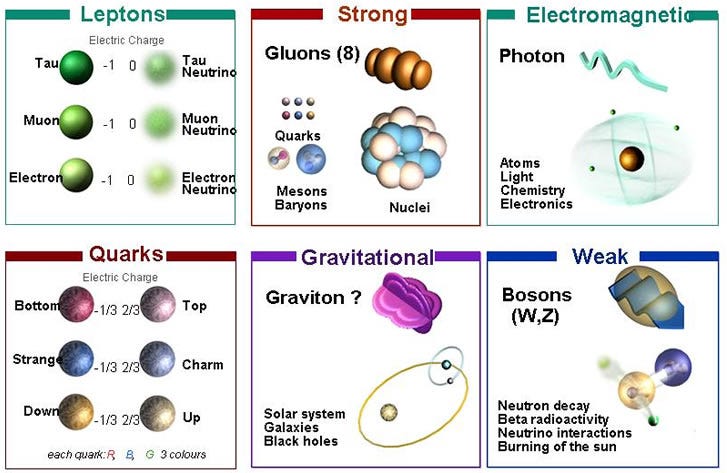
This week, I had a hard time choosing from all the interesting questions and suggestions I received, but I settled on this mind-bender from Rui Carvalho, who asks the following:
If we could take a bit of a neutron star (lets say a cubic centimeter) and pull that bit away from the star, what would happen to it?
What’s the deal with neutron stars, anyway?
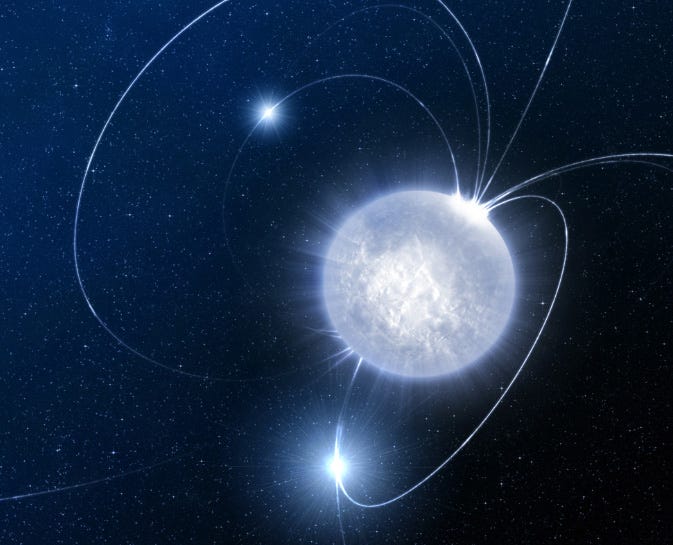
They are, as their name implies, a ball of neutrons, bound together through their intense gravitation, of approximately the mass of a star like our Sun. This is nuts, of course, since neutrons shouldn’t really exist for very long. After all, you can take any particle you like, leave it in isolation, and watch what happens. Of the three particles that make up most of the normal matter we know of — protons, neutrons and electrons — the results are very different.
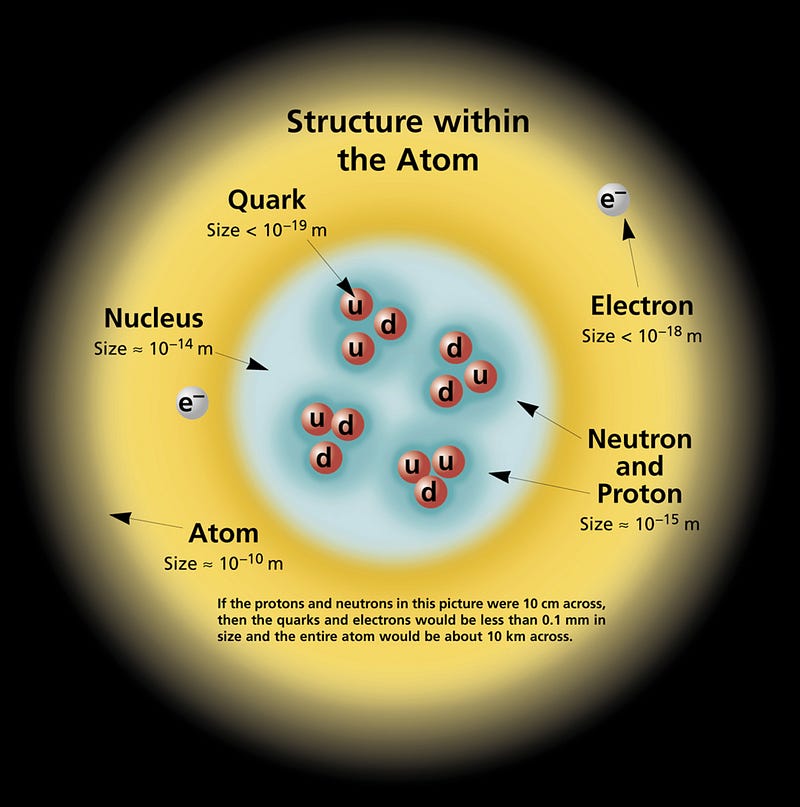
Electrons are fundamental particles, and the lightest stable particle with an electric charge. As far as we can tell, electrons are perfectly stable, with no possible path for decay.
Protons are composite particles, made up of quarks and gluons. In principle, there might be a way for protons to decay, so we’ve gone and looked for it. What we’ve done is build giant tanks filled with individual protons — huge tanks with some 10^33 protons inside — and waited years to see if even one of them decays. After decades of experiments like this, we’ve determined that if the proton is unstable, it has a half-life of at least 10^35 years, or some 10^25 times the present age of the Universe. As far as we can tell, protons are perfectly stable, too.
Not so with neutrons! Take a free, unbound neutron, watch it, and it’ll most likely be gone in about 15 minutes, having decayed into a proton, an electron, and an antineutrino. (Its half-life is less: about 10 minutes.)
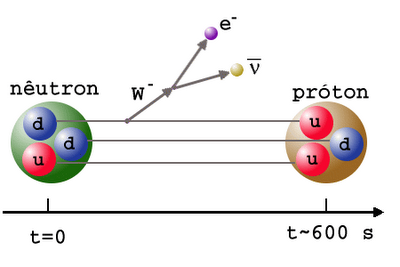
So how, then, can we hope to have such an entity as a neutron star?
There’s a difference between a free neutron and a bound neutron, which is also the reason why many of the elements and isotopes don’t decay: when nuclei are bound together, there’s a certain amount of binding energy there: enough to keep the neutrons stable!
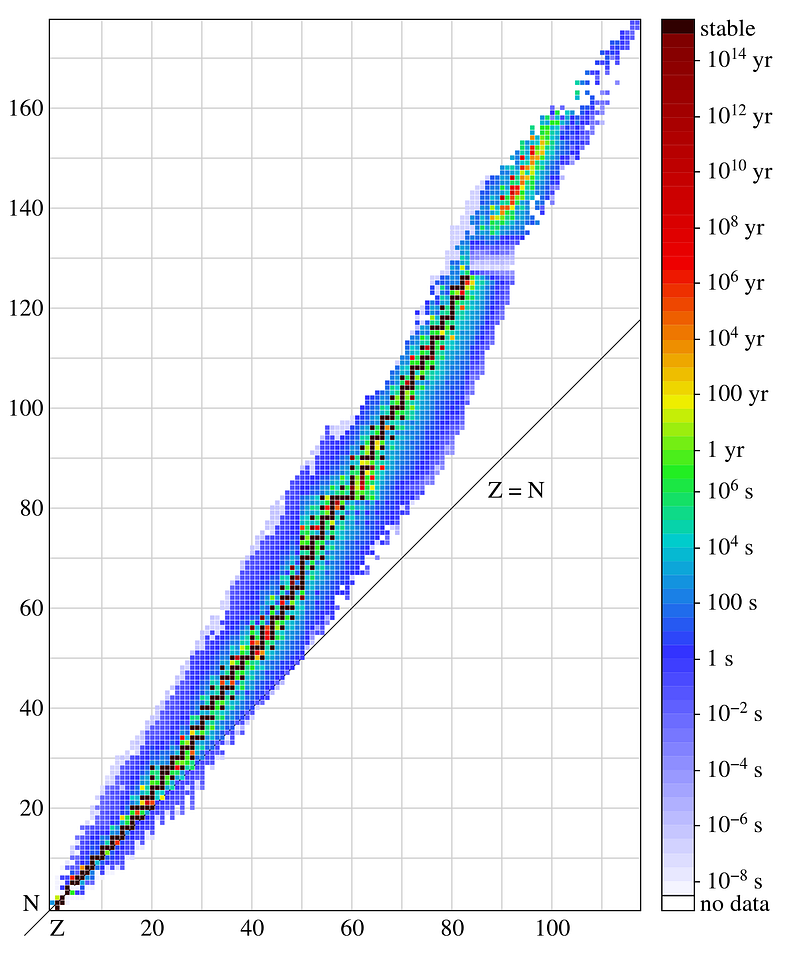
For the elements, certain configurations are more stable than others, with a little over 254 possible configurations, as far as we can tell, being completely stable against radioactive decay. (It’s conceivable that on long enough timescales, a great many of these will turn out to be unstable; we simply haven’t observed that yet.) But none of these are very heavy, or consist of very many neutrons at all. The heaviest stable element? That’s lead, element 82, with four known, stable isotopes: Pb-204, Pb-206, Pb-207 and Pb-208.
So of all the elements known, an atomic nucleus with 82 protons and 126 neutrons is the heaviest stable one.
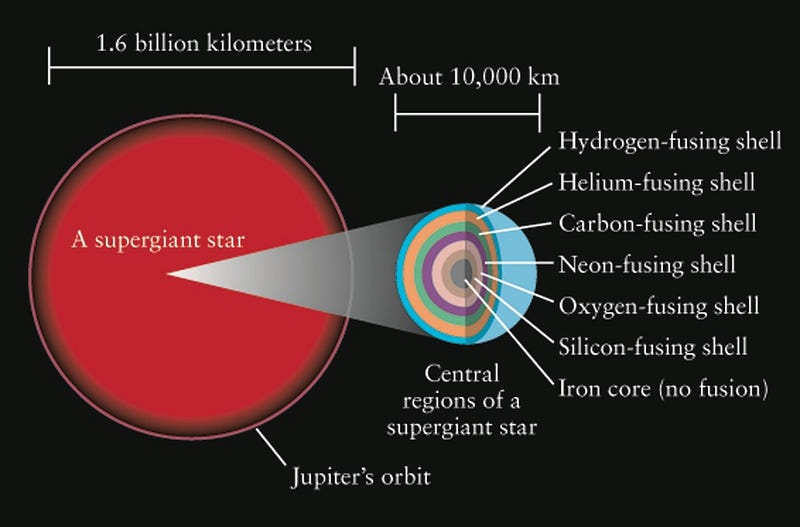
But that’s assuming that the nuclear force is what’s binding you together. In the case of a neutron star, there’s something else responsible. To understand what’s happening here, let’s understand how a neutron star gets created.
In the most massive stars — the brightest and bluest ones created in young star clusters — they fuse hydrogen into helium in their cores, like all young stars. Unlike stars like the Sun, though, it doesn’t take them billions of years to burn through their fuel, but rather only a few million (or even less), since the extremely hot temperatures and densities inside lead to an incredibly rapid rate-of-fusion.
When they run out of hydrogen fuel in their core, the interior begins to contract, causing it to heat up. When it reaches a certain critical temperature, the helium in the core begins fusing into carbon, resulting in an even larger rate-of-energy release.
After just a few thousand years, the helium fuel becomes exhausted, and the interior collapses even farther, heating up to temperatures our Sun’s core will never achieve. Under these extreme conditions, the carbon in the core begins fusing into oxygen, and then in similar, successive reactions, oxygen fuses into silicon and sulphur, silicon fuses into iron, and then… well, then we have a problem.
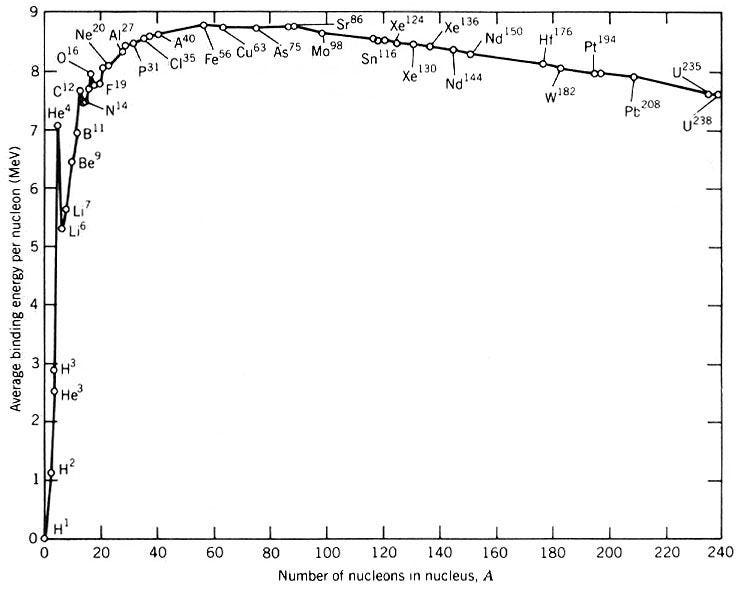
Iron, you see, is the most stable element. With 26 protons and 30 neutrons in its nucleus, it has the highest binding-energy-per-nucleon, which means that any other configuration is less stable than that one. (By some metrics, nickel-62 is more stable, but we’ll go with iron-56 for simplicity.) You know that heavier elements than iron exist, but you don’t create them by fusing iron with any other element. Rather, when the core becomes filled with iron, it begins to gravitationally contract, and there’s no longer a source of fuel to burn. All that you’re left with is an incredibly hot, dense plasma that’s becoming hotter and denser over time.
But finally, a threshold is reached, and — quite surprisingly — electrons and protons begin to fuse together, creating neutrons, neutrinos and energy!

This runaway reaction produces so much energy that the entire outer layer of the star is destroyed in a supernova, with the fusion of electrons and protons into neutrons and neutrinos taking only a matter of seconds.

While the outer layers will take weeks-to-months to be blown off, the core condenses down to a ball of neutrons under the tremendous influence not of the nuclear force, but of gravity.
At its core, a neutron star is about a Sun’s worth of mass condensed into a volume just a few kilometers in radius. Its density is some 10^19 kilograms-per-cubic-meter, or the densest physical, three-dimensional object known in the Universe.
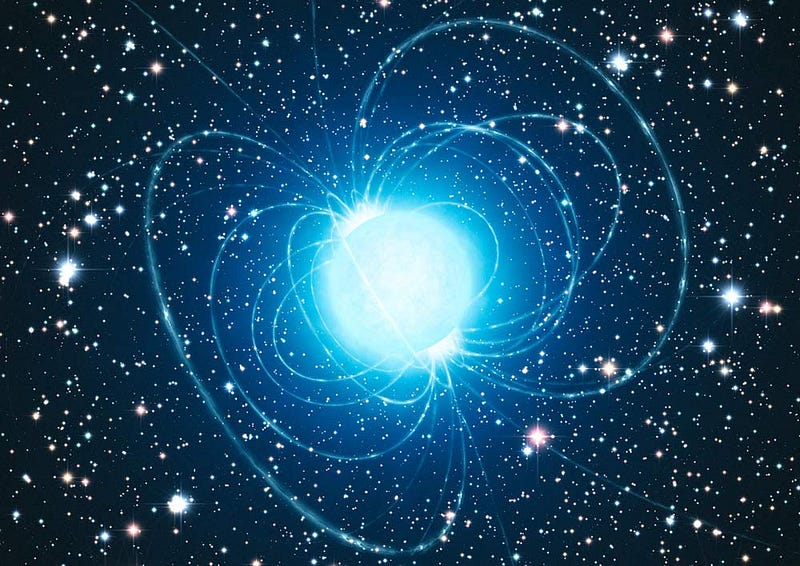
In order for a neutron to be stable against radioactive decay, it needs to have a binding energy that’s greater than the mass difference between a neutron and a proton, or about 1 MeV, about 0.1% of the neutron’s mass. And while the neutrons at the core will be easily bound, the ones on the surface will be the most tenuous. If we take a neutron star to be equal to the mass of the Sun and only 3 kilometers in radius, a neutron bound at its surface would have about 400 MeV of binding energy: plenty to keep it from decaying.
But what if we pulled a cubic centimeter of this matter, as Rui asks, from the neutron star itself? What would we have, then?
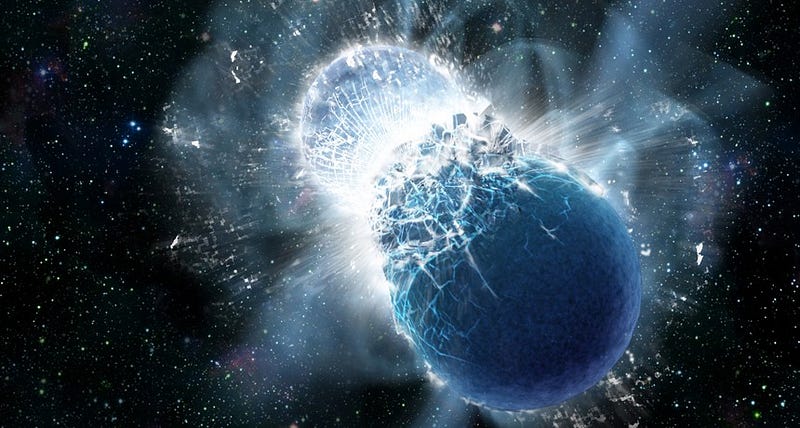
Unfortunately, the gravitational binding energy of the neutrons on the surface would be only about 0.07 electron-Volts, a woefully insufficient amount to keep the neutrons from decaying!
We actually run into a somewhat analogous situation to this in the natural Universe: when neutron stars collide with other neutron stars. While most of the matter might merge to form a black hole, about 3% of the mass gets ejected. Instead of leading to exotic matter, it all decays away incredibly rapidly, giving rise to a large fraction of the heaviest elements in the periodic table. If you ever wondered where most of the elements like gold on Earth come from, this is it: from the merger of neutron stars!
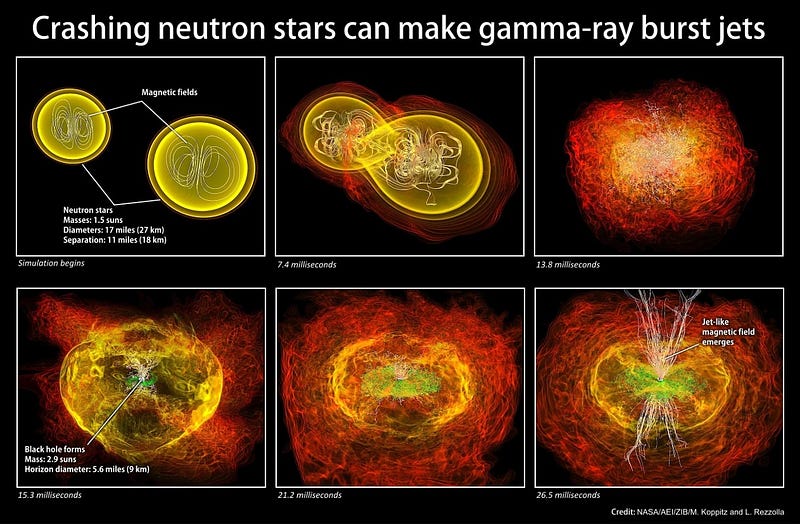
So if you pulled out too small of a mass of neutrons, it would simply fragment and decay into stable (or long-lived) elements and isotopes of the periodic table in short order, on the timescale of a neutron’s lifetime at most, and possibly on much shorter ones.
If we wanted to pull off a large enough chunk of mass to keep the neutrons at the surface stable? You’d need it to be about 200 meters in radius, or about eight times the diameter of Disney’s Spaceship Earth at Epcot.
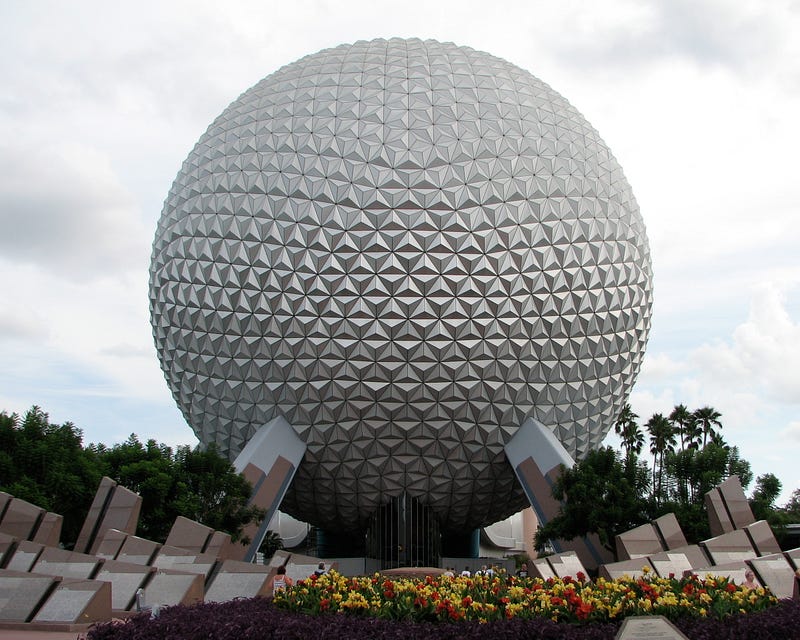
At this point, you’re dealing with enough matter to be comparable to about the mass of Saturn, and that’s the lower-limit of what you’d need. Anything less massive, and your ball of neutrons will decay.
So as much as you might like to believe that neutron-star-matter is what the Mighty Thor’s hammer is made out of…
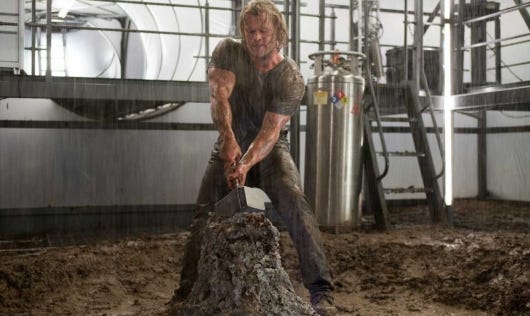
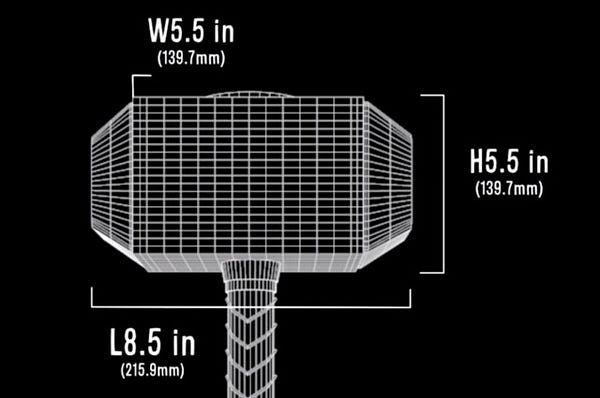
physics simply won’t allow it. It’s too small, the gravitational binding energy at the surface is too little, and it would simply (and catastrophically) radioactively decay.
So thanks for a great question, Rui, and I hope if you have dreams of creating the tiniest neutron star, you start thinking big! If you’ve got a question or suggestion for next week’s Ask Ethan, go ahead and send it in, and I’ll see you back here soon for more wonders of the Universe!
Leave your comments at the Starts With A Bang forum on Scienceblogs!