7 Independent Pieces Of Evidence For Dark Matter
With the full suite of evidence, there’s no escaping dark matter.
“A cosmic mystery of immense proportions, once seemingly on the verge of solution, has deepened and left astronomers and astrophysicists more baffled than ever. The crux… is that the vast majority of mass of the universe seems to be missing.” –William J. Broad
When we look out at the Universe, it’s only natural to imagine that the same stuff we see wait out there — among the stars, galaxies, and in the great, dark void of intergalactic space — would be made out of the same stuff that’s close to home: protons, neutrons and electrons. After all, our world and everything on it, our Solar System and everything in it, and our Milky Way (to the best of our knowledge) and all it consists of is made out of exactly that.
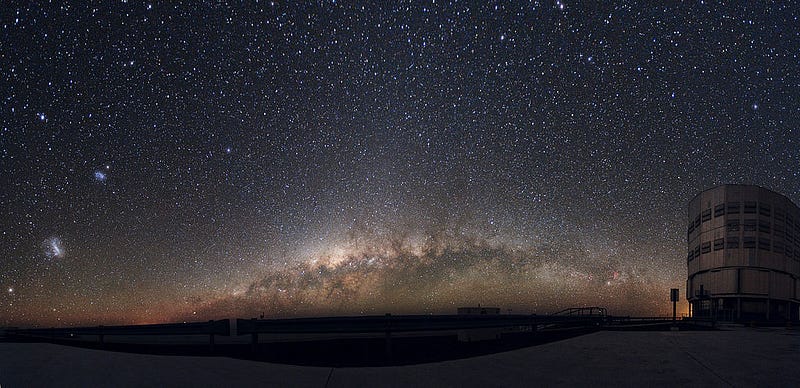
Even if that somehow weren’t the case, we’d still expect they’d be made up of some combination of the known, discovered fundamental particles. When it comes to every form of matter that’s known to exist, the Standard Model of elementary particles covers it all. If it’s been created, measured or observed in a laboratory setting, it’s contained in this chart, below.
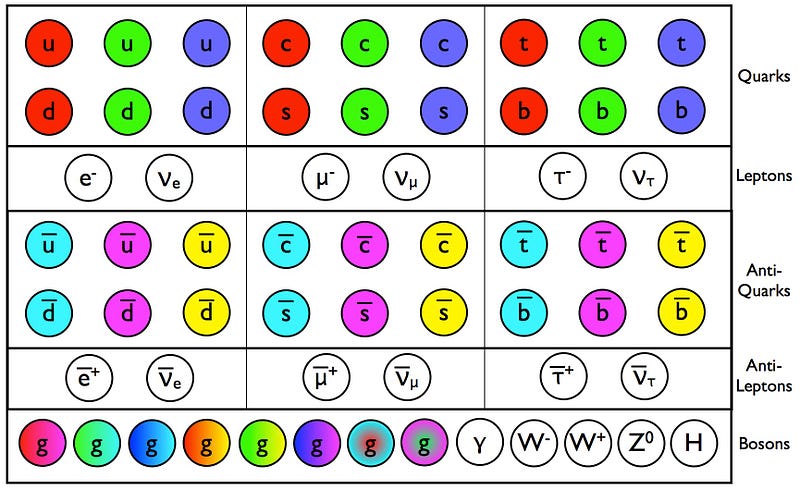
And yet, that seems not to be the case. The overwhelming consensus among physicists is that the stuff that’s “known,” or made up of all the particles (and antiparticles) contained in the Standard Model in the entire Universe, is only a tiny fraction of the mass that’s out there.
What would lead us to such a conclusion? Below are seven facts about the Universe — facts anyone could investigate and find out for themselves — that leads us to the inescapable conclusion that the vast majority of matter in the Universe isn’t found in the Standard Model, isn’t made of protons, neutrons and electrons, but rather is some new form of dark matter that must exist.
Let’s start!
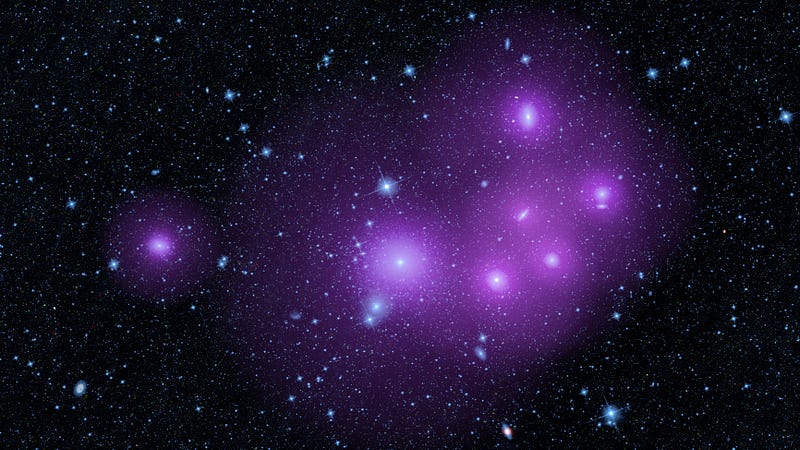
1.) The amount of normal matter in the Universe is a known quantity!
There are two ways to approach this problem:
- Measure and quantify all the normal matter in all its various forms everywhere in the Universe and add it all up.
- Figure out a way to relate the quantity you want to understand — how much matter is present — to something you can measure, and then measure it!
The first way is the most straightforward, and includes not only planets and stars, but all forms of matter imaginable, including gas, dust, plasma, free electrons, white dwarfs, brown dwarfs, neutron stars, black holes, antimatter and neutrinos, just to name a few of the most major ones. We add them all up, and we get a number.
But there’s another way that precludes this matter from hiding in some hitherto undiscovered form.
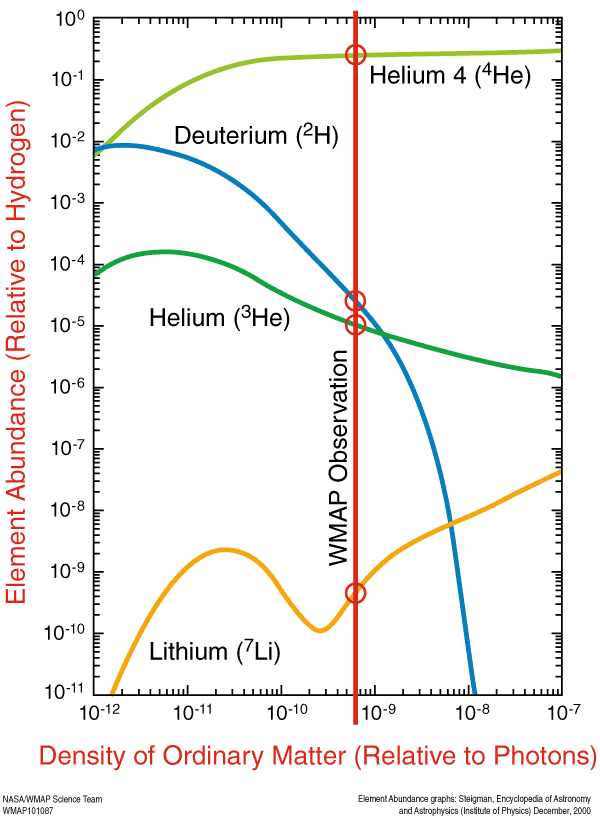
Since we know the Universe emerged from a hot, dense state, we know it at one point formed the first stable atomic nuclei. If we can find a sample of matter — of neutral gas — before any stars ever formed in it, we can measure what the ratios of the various elements were. The laws of physics are known, and make very specific predictions for how much hydrogen, deuterium, helium-3, helium-4, and lithium-7 ought to be present in the Universe. That’s five independent, measurable quantities, defined by only one parameter: the amount of normal matter in the Universe.
We’ve measured all five, and now we know: the normal matter is only about 5% of what’s required to be responsible for all the energy in the Universe.
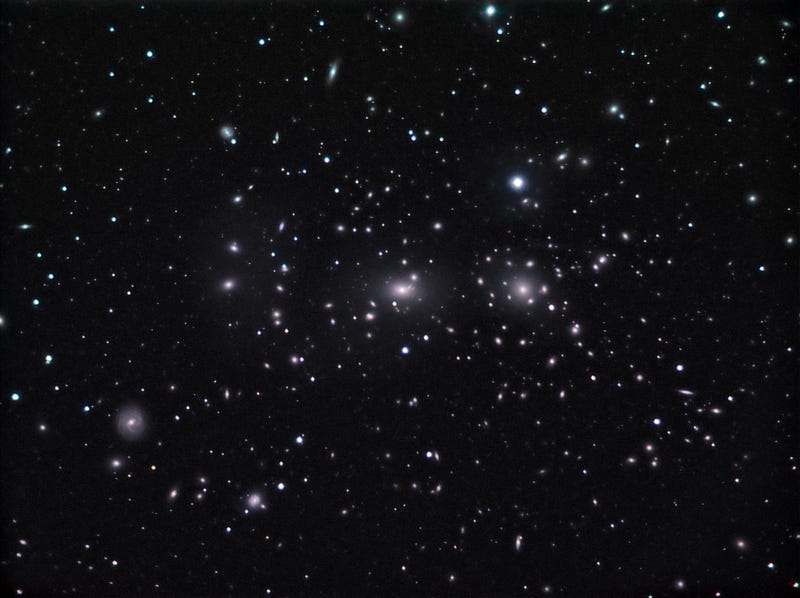
2.) Clusters of galaxies are bound together!
When we look at clusters of galaxies — some of the largest bound structures in the Universe — we find that they contain anywhere from hundreds to many thousands of individual galaxies, all bound together within a relatively compact region of space. Based on how quickly they’re moving (and the known laws of gravity), we can infer how much total mass has to be in there in order to keep the clusters bound together.
We can also, based on all the matter we observe: starlight, gas, dust, plasma, X-rays when the gas gets heated, etc., conclude how much normal matter must be in there. There’s a lot! But it’s not enough. It’s only about 13–17% of the total mass necessary to keep the clusters bound. There has to be some other form of matter in there to account for the mass: some form of dark matter.
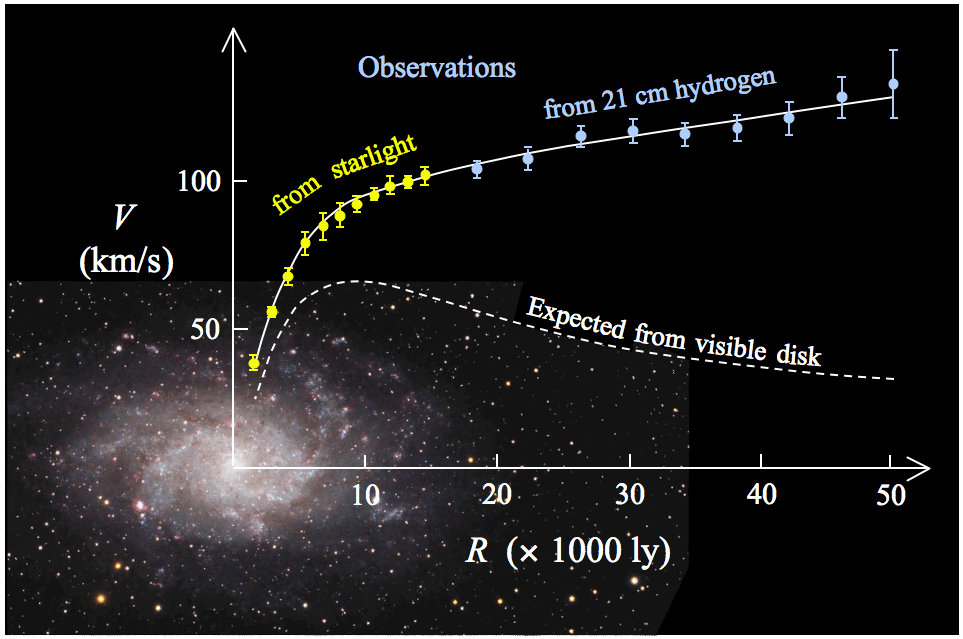
3.) Individual galaxies must have more than gas and dust in them to account for their observed dynamics.
If there’s one thing you know about spiral galaxies, it should be this: they rotate, and it’s that rotation that gives rise to that classic spiral structure you know so well. But when a galaxy faces us edge-on, we can tell which parts of the galaxy are rotating towards us and which parts are rotating away from us, thanks to the red-and-blue-shifting of the light.
Not only that, but we can measure how quickly it’s rotating at different distances from its center. If most of the mass were concentrated centrally, which normal matter in all its forms ought to do, we’d see the outskirts rotate more slowly than the inner portions. But this doesn’t happen, leading to the idea that there must be a “halo” of dark matter surrounding each and every galaxy to account for the observed rotation curves.
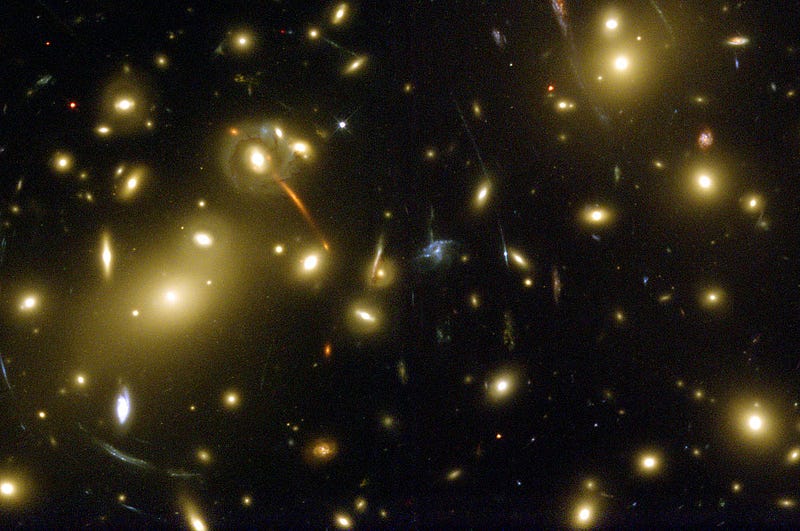
4.) Gravitational lensing measures total mass, and tells us there’s more than normal matter alone permits!
When we look out at the Universe, we don’t only measure light from a galaxy or cluster to infer information about the Universe. Thanks to Einstein’s General Relativity, we have an incredible mechanism for measuring mass: the fact that mass itself can act like a lens, bending all the light from the objects behind it, a phenomenon known as gravitational lensing. This can come in the form of strong lensing, above, which shows how great rings, arcs, and multiple images can form, or weak lensing, below, which distorts the shapes of background galaxies in a well-understood fashion.
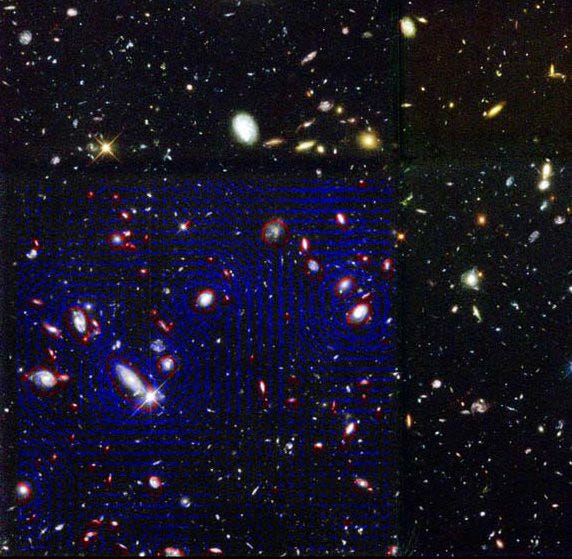
You can measure one or both of these effects, and so long as you have enough background light coming through, you can infer how much mass is present in the lensing (foreground) object. Through every observation ever made, we’ve measured a “total mass” that’s consistent with being approximately six times as great as the amount of mass that we expect from the normal matter alone.
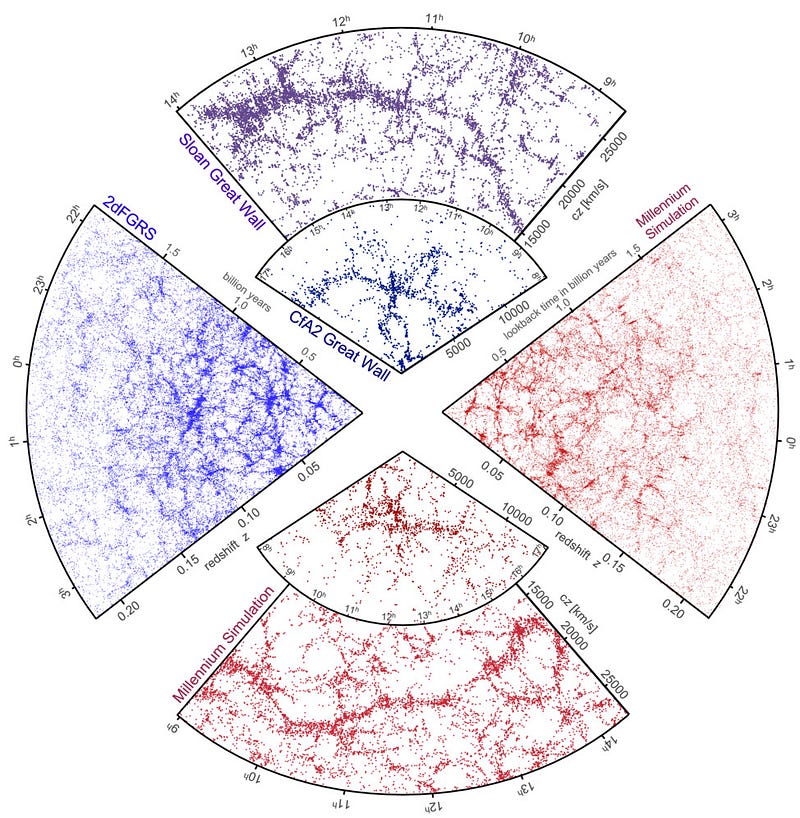
5.) Large-scale-clustering requires dark matter to reproduce the observed structure.
When we make our most accurate maps of galaxies in the Universe on the largest scales, we find that there absolutely has to be some type of matter that’s different from normal matter — protons, neutrons and electrons — to reproduce the structures we see on the largest scales. In particular, dark matter produces a hierarchical cosmic web, where we have tiny, dwarf galaxies, larger spirals of various sizes, groups containing multiple large spirals, clusters with many spirals and giant ellipticals, filaments connecting the clusters, and great voids with very little matter in the space between.
If there were no dark matter, the Universe we’d see would be very, very different.
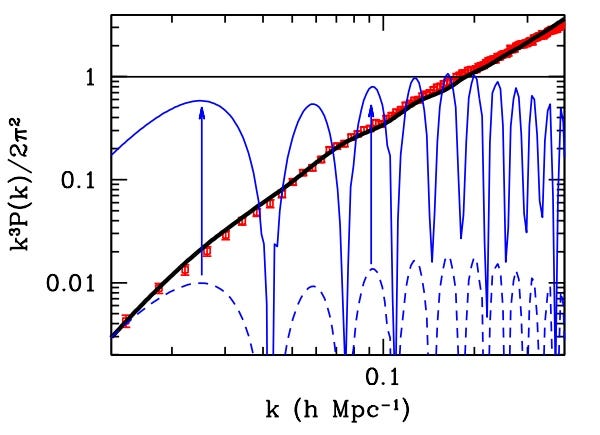
For one, there would be a cutoff in large-scale-structure; we wouldn’t have any below a certain size. For another, there would be “valleys,” or scales on which there were no clustered objects. And finally, the “acoustic features” (or wiggles) in the graph above would be greatly exaggerated. Those wiggles are created by normal matter and suppressed by dark matter; the observed amount of “wiggles” is again consistent with a 5:1 ratio of dark matter to normal matter.
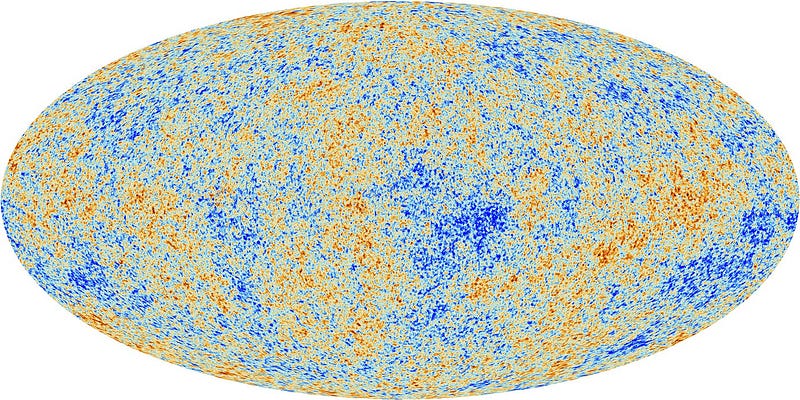
6.) Fluctuations in the Cosmic Microwave Background (CMB).
This is a huge one! When we look out at the leftover glow from the Big Bang (the CMB), we find that there’s a very specific pattern in how these fluctuations are clustered together. While the fluctuations start out the same on all scales, interactions between radiation and matter create “waves” similar to ripples in a body of water on very specific scales. If there’s dark matter present, it affects the radiation and normal matter due to gravity, but doesn’t interact the way normal matter does with itself or radiation.
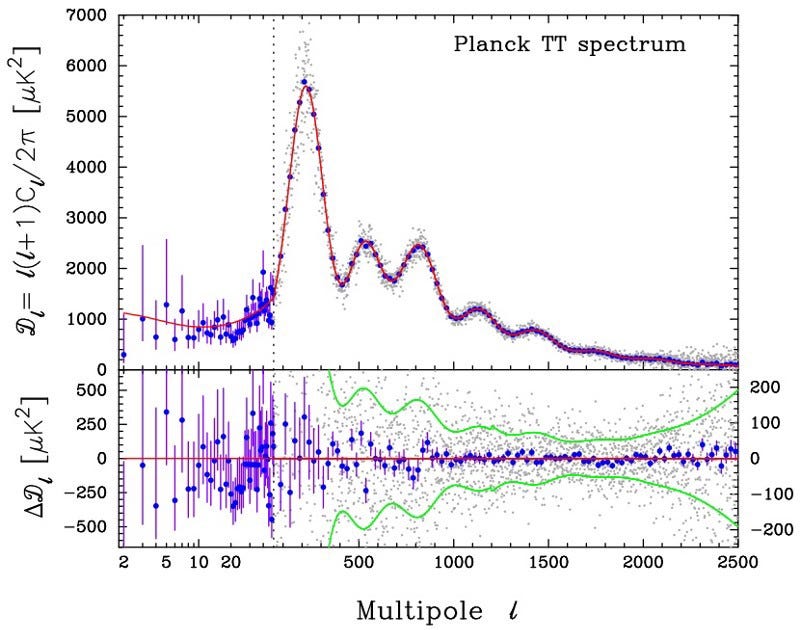
So we reconstruct this pattern of fluctuations, and find that it’s only consistent with a Universe that’s made up of 5% normal matter, 27% dark matter and 68% dark energy. While the dark energy is interesting in its own right, the important takeaway here is that, again, we see that same 5:1 ratio of dark matter to normal matter.
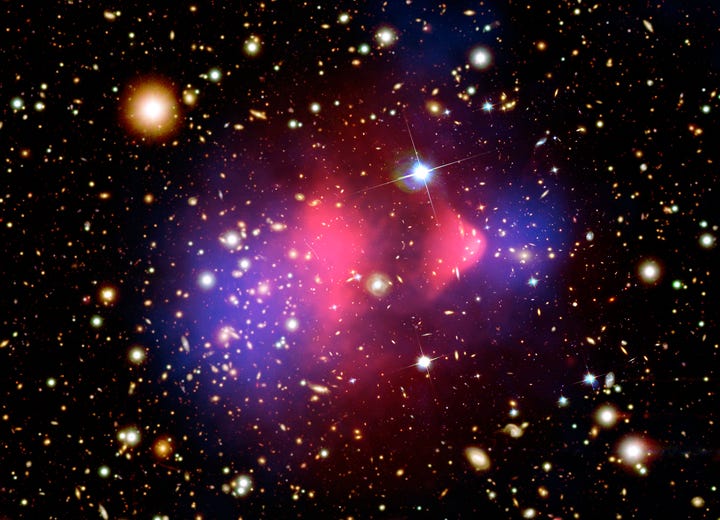
7.) Colliding galaxy clusters show that the majority of gravitation isn’t where the majority of normal matter is!
Finally, the most dramatic and extraordinary piece of evidence comes from colliding galaxy clusters. That’s right: every so often, albeit incredibly rarely, two galaxy clusters find each other in this vast, empty Universe, brought together by their tremendous mutual gravitational attraction. The clusters collide, and while the collapsed objects (like individual stars) pass right through one another, the diffuse, neutral gas inside collides with the gas in the other cluster. When that happens, the gas heats up and slows down, collecting in the center and emitting X-rays (shown in pink). But when we use the technique of weak gravitational lensing to reconstruct where the mass is (in blue), we find that it passed through, along with the stars.
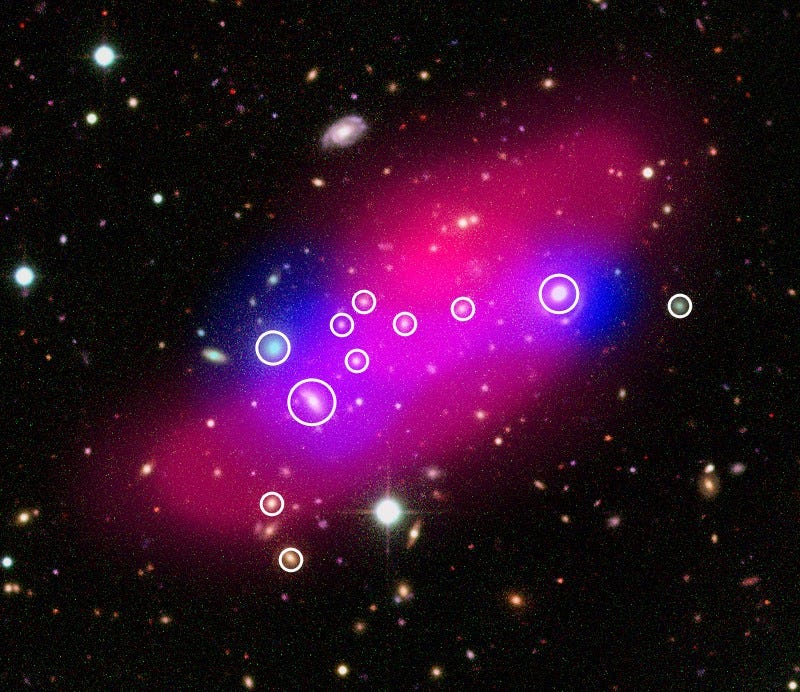
Since stars are only a small fraction of the normal matter’s mass, we know there’s got to be some form of dark matter responsible for the vast majority (again, about 85%) of the mass in these clusters. There have been many clusters where this effect has been observed, down to groups (above) just a few times larger than our own small local group.
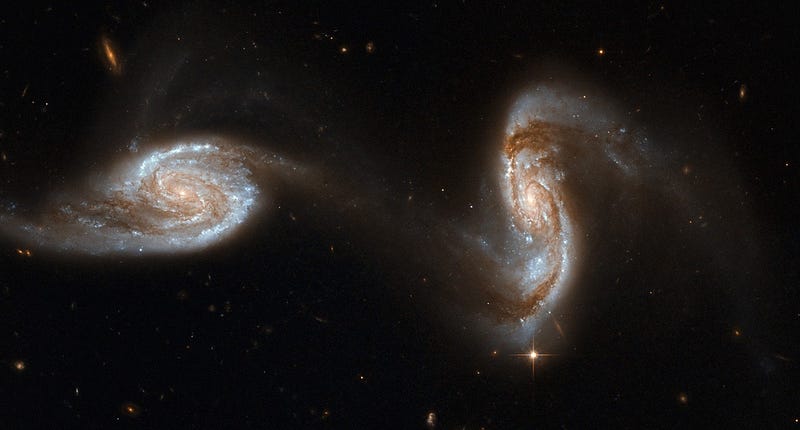
There are many other independent ways to measure dark matter’s abundance, normal matter’s insufficiency or the dark-matter-to-normal-matter ratio, including from peculiar velocities of galaxy pairs, through the magnitude of the acoustic peak from baryon acoustic oscillations, the insufficient magnitude of MACHOs (or baryonic dark matter) within our galaxy, etc. While any one piece of evidence on its own may be argued away or can replace dark matter with an alternate explanation, the full suite of evidence points towards the incontrovertible existence of dark matter.
Any Universe without it simply wouldn’t look like ours.
Leave your comments at our forum, and support Starts With A Bang on Patreon!