6 Supermassive Questions On The Eve Of The Event Horizon Telescope’s Big Announcement

What should a black hole look like? Our theoretical predictions are about to meet our first observations.
In science, there’s no moment more exciting than when you get to confront a longstanding theoretical prediction with the first observational or experimental results. Earlier this decade, the Large Hadron Collider revealed the existence of the Higgs boson, the last undiscovered fundamental particle in the Standard Model. A few years ago, the LIGO collaboration directly detected gravitational waves, confirming a longstanding prediction of Einstein’s General Relativity.
And in just a few days, on April 10, 2019, the Event Horizon Telescope will make a much-anticipated announcement where they’re expected to release the first-ever image of a black hole’s event horizon. At the start of the 2010s, such an observation would have been technologically impossible. Yet not only are we about to see what a black hole actually looks like, but we’re about to test some fundamental properties of space, time, and gravity as well.
If you want to image any object in the Universe, you have to meet the following two challenges:
- You must gather enough light to see your target, and reveal its details against the background noise of both your instruments and the other objects in the vicinity of your object of interest.
- You need sufficient resolution (or resolving power) to reveal the structure of the object you’re looking at, otherwise all of your data will be confined to one mere pixel.
So if you want to image a black hole’s event horizon, you need to both gather enough light that the radiation around the black hole stands out against the rest of the environment, and also to probe angular scales that are narrower than the diameter of the event horizon itself.
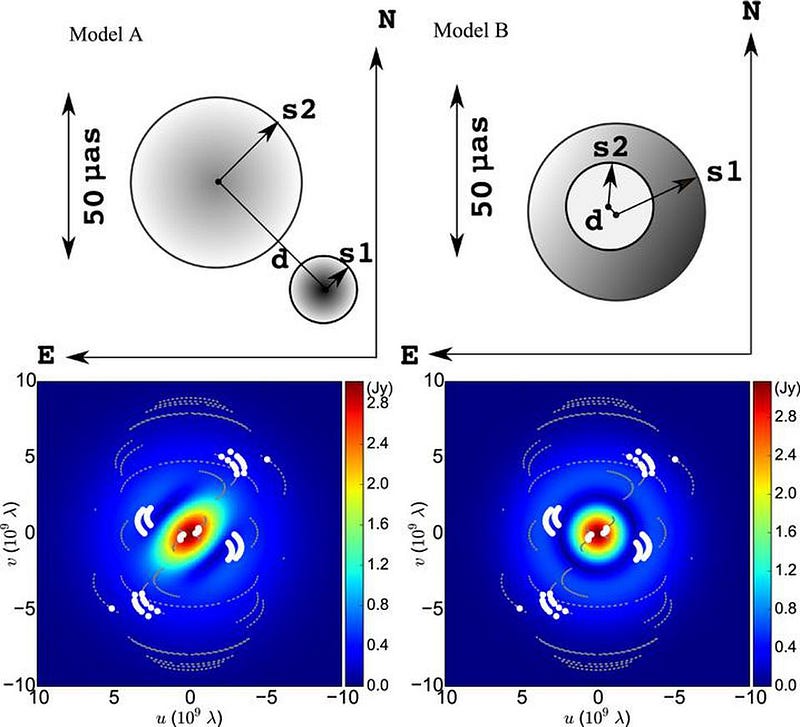
The only way we have of doing both of those is with an enormous, ultra-sensitive array of radio telescopes that observe the largest black holes, in terms of angular size, that are visible from Earth. The more massive your black hole is, the bigger the diameter of its event horizon will be, but it will appear smaller dependent on its distance. That means the largest black hole will be Sagittarius A*, the supermassive one at the center of the Milky Way, while the second largest will be the ultramassive one at the center of the galaxy M87, some 60 million light-years away.
While single-dish radio telescopes might be able to detect the emissions from either one — i.e., they have sufficient light-gathering power — they cannot resolve the event horizon. But an array of telescopes, all observing the target together, can get us there.
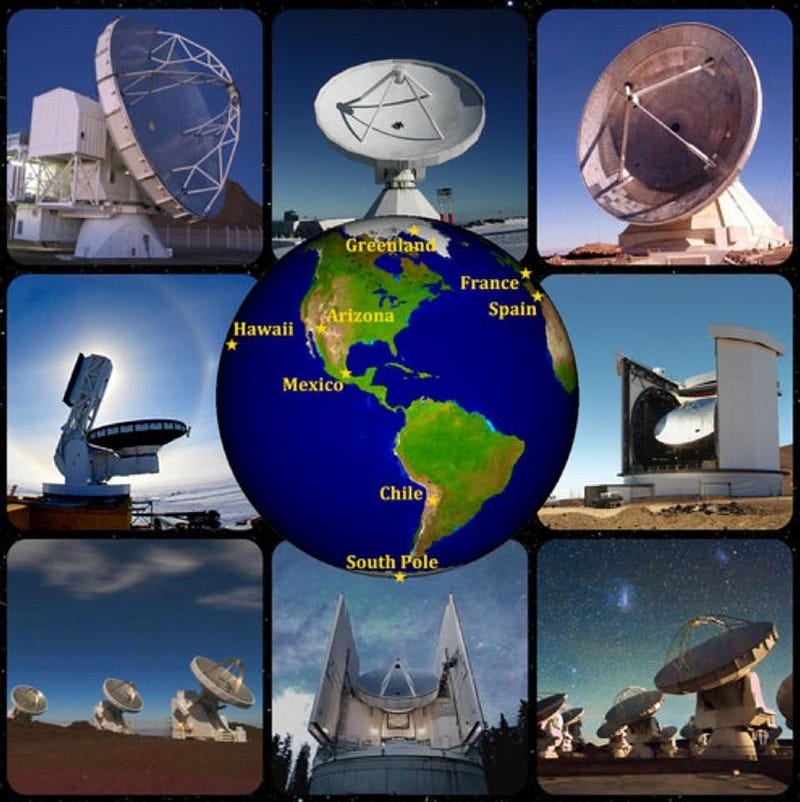
Black holes ought to be surrounded by matter that’s in the slow process of being devoured. This material will be strewn about the black hole’s outsides, spinning around, heating up, and emitting radiation as it falls in. That radiation should come in the radio part of the spectrum, and be observable to a sensitive-enough telescope array.
The Event Horizon Telescope (EHT) is exactly the radio array we need — with the most stunning advances coming from the inclusion of ALMA in South America — to not only gather the radio information, but to get that excessive resolution. The EHT consists of scores of individual dishes with enough combined light-gathering power to reveal the radiation surrounding the black hole, with the distances between the dishes providing the resolution necessary to image the event horizons in question themselves.
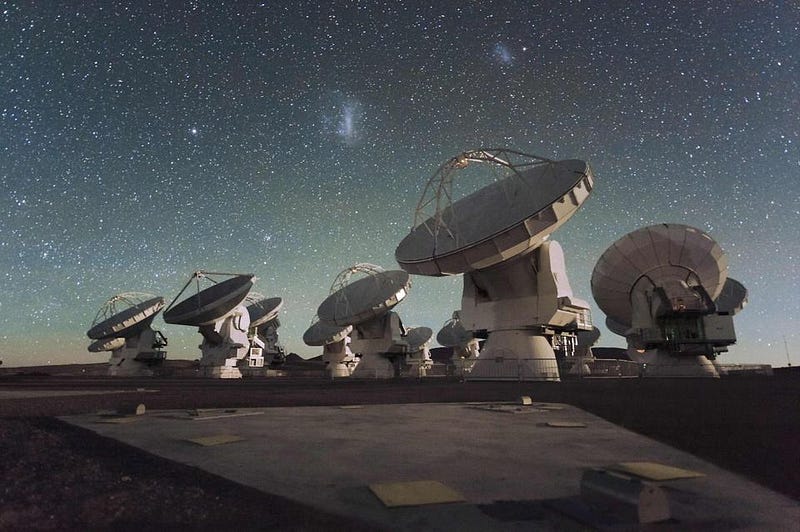
We’ve used this technique before, of long-baseline interferometry, to reveal details that would be invisible with even an enormous single-dish telescope. So long as the features you’re attempting to observe are bright enough and show up in the telescopes you’re using to take the observations simultaneously, you can achieve imaging resolutions that correspond to the distance between the telescopes, rather than of the diameter of the individual telescopes themselves.
Most spectacularly, telescope arrays have been used thus far to image erupting volcanoes on the surface of Jupiter’s moon Io, even at the moment that Io falls into the shadow of another of Jupiter’s moons.
The EHT uses this exact same concept to probe the radiation coming from around the black holes with the largest angular diameters as seen from Earth. Here are the six things we’re poised to learn when the first-ever images are released.
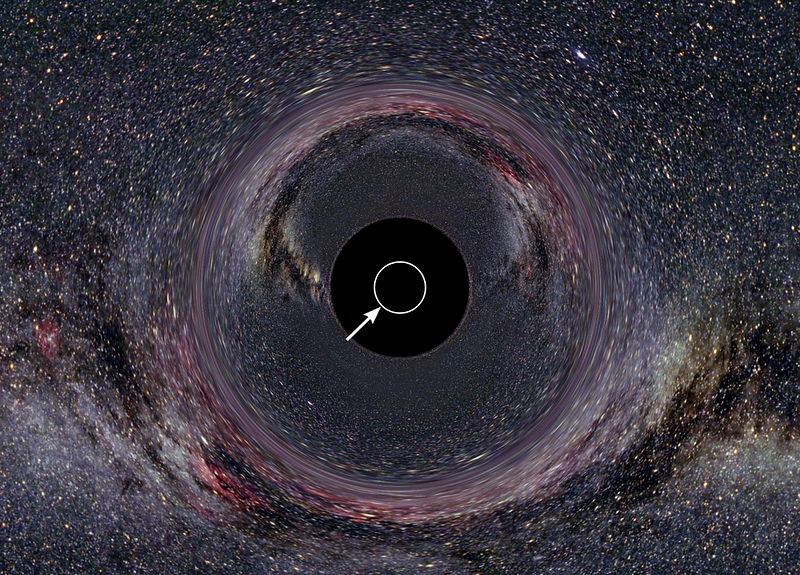
1.) Do black holes have the correct sizes that General Relativity predicts? According to Einstein’s theory, based on the measured gravitational mass of the black hole of the Milky Way’s center, the event horizon itself should be 11 micro-arc-seconds (μas) in diameter, but there should be no emissions coming from within 37 μas, owing to the fact that within that angular diameter, matter should quickly spiral in towards the singularity. With a resolution of 15 μas, the EHT should be able to see a horizon, and measure whether the size matches our predictions or not. It will be a fabulous test of General Relativity.
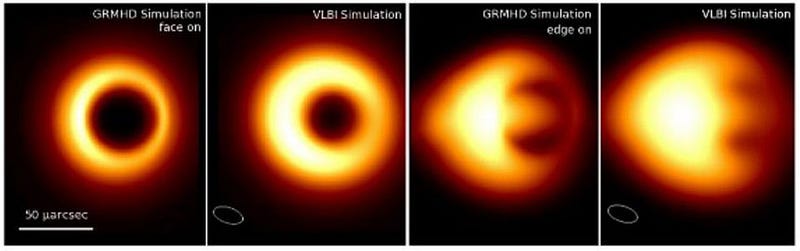
2.) Are the accretion disks aligned with the black hole, the host galaxy, or randomly? We’ve never observed an accretion disk before, and in fact the only real indication we have about the orientation of the matter surrounding black holes comes from the cases where either:
- there’s an emitted jet that we can detect from the black hole,
- or there’s extended emission coming from the surrounding region.
But neither one of those observations are a substitute for a direct measurement. The EHT, when these first images come out, should be able to tell us whether the accretion disk is edge-on, face-on, or at any other orientation.
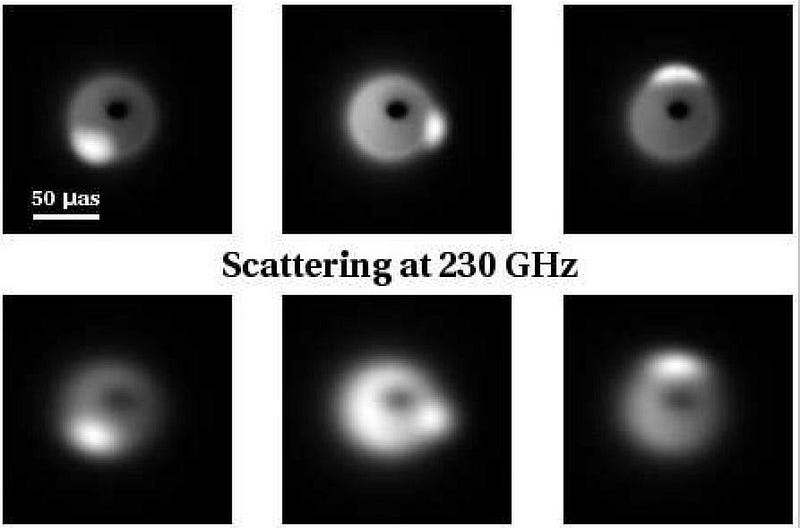
3.) Is a black hole’s event horizon circular, as predicted, or does it take on a different shape? Although all physically realistic black holes are expected to spin to some degree, the event horizon’s shape is predicted to be indistinguishable from that of a perfect sphere.
But other shapes are possible. Some objects bulge along their equators when they rotate, creating a shape known as an oblate spheriod, such as planet Earth. Others creep up along their rotational axes, resulting in a football-like shape known as a prolate spheroid. If General Relativity is correct, a sphere is what we anticipate, but there’s no substitute for making the critical observations ourselves. When the images come out on April 10, we should have our answers.
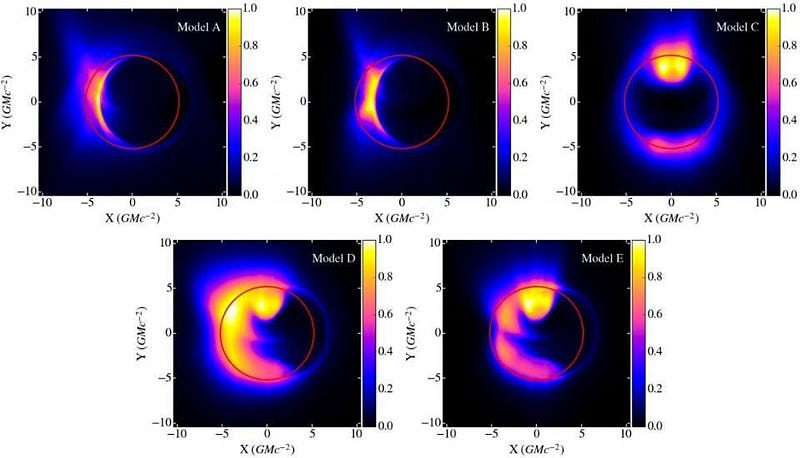
4.) Why do black holes flare? When a black hole is in a non-flaring state, there are specific signatures that we anticipate will show up around the event horizon. But then, when a black hole flares, there are different features that the radiation surrounding it will exhibit.
But what will those emissions look like? Will there be turbulent features that show up in the disk at all times? Will there be “hot spots,” as predicted, that are most visible in the flaring state? If we get lucky and see either of these signatures, we might be well on our way to learning why black holes flare, just by observing the extended radio emissions surrounding them. We should also learn, based on these observations, additional information about the strength of the magnetic fields surrounding these black holes.
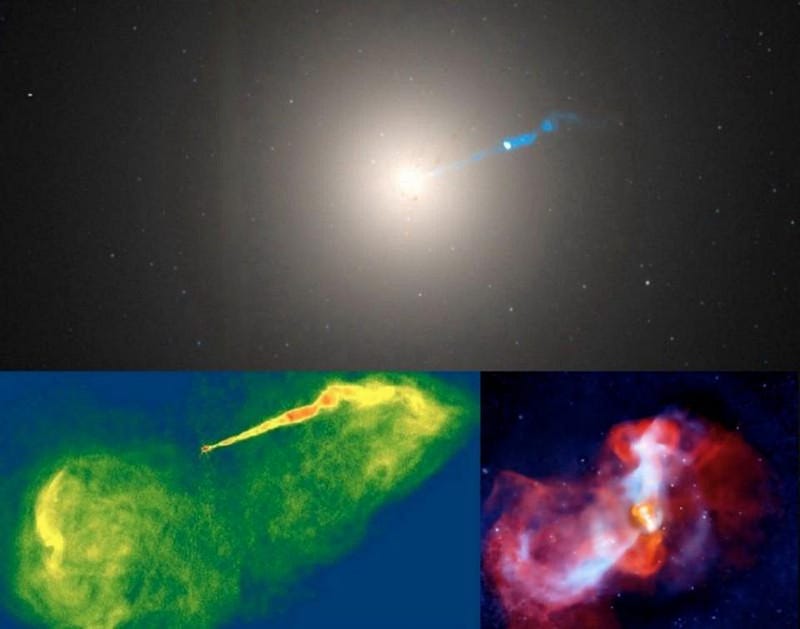
5.) Are the X-ray estimates of a black hole’s mass biased towards lower values? There are, at present, two ways to infer the mass of a black hole: from measuring its gravitational effects on stars (and other objects) that orbit it, and from the (X-ray) emissions of the gas that orbits it. We can easily make the gas-based measurements for most black holes, including the one at the center of the Milky Way, which gives us a mass of approximately 2.5–2.7 million solar masses.
But the gravitational measurement is far more direct, despite being a greater observational challenge. Still, we’ve done it in our own galaxy, and have inferred a mass of approximately 4 million solar masses: about 50% higher than the X-ray observation indicates. We fully expect that this will be the size of the event horizon we measure. If the measurements of M87 show a higher value than the X-ray emission indicates, we could learn that the X-ray estimates are systematically low, showing us there’s new astrophysics (but not new fundamental physics) at play.
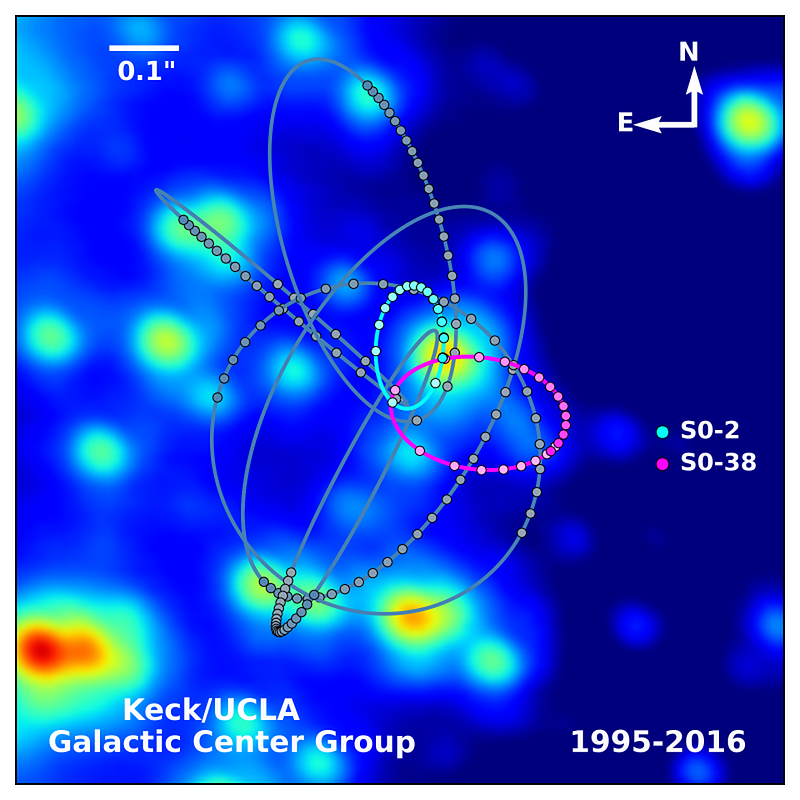
6.) Can we see the black hole “jittering” over time, as predicted?This one may not come out right away, especially if all we get from these initial observations is a single image of one or two black holes. But one of the science goals of the EHT is to observe how black holes evolve with time, meaning that they plan on taking multiple images at different times and reconstructing a movie of these black holes.
Because of the presence of stars and other masses, the apparent position of the black hole will change significantly over time, as it gets gravitationally pushed around. Although it will likely take years to observe a black hole move by an appreciable amount, we have data that was taken over the course of a long time. At the centers of galaxies, EHT-imaged black holes may begin to exhibit signs of this jitter: the cosmic equivalent of Brownian motion.
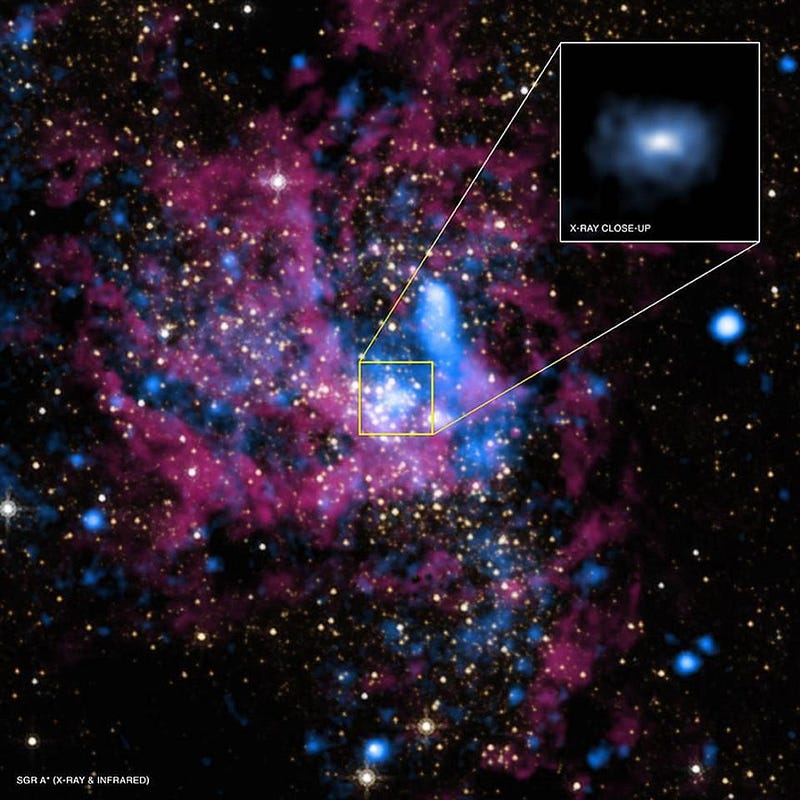
The critical observations for creating the first image of a black hole, assuming the EHT publishes one of the black hole at the Milky Way’s center, were taken way back in 2017: two full years ago. It’s taken this long to analyze, clean up, cut, adjust, and synthesize the full suite of data, which equates to some 27 petabytes for the critical observation. (Although only about 15% of that data is relevant and usable for constructing an image.)
At 9 AM Eastern Time (6 AM Pacific Time) on April 10, the EHT collaboration will hold a press conference where they’re expected to release the first image of an event horizon, and it’s possible that many — or possibly even all — of these questions will be answered. Whatever the results, this is a monumental step forward for physics and astrophysics, and ushers in a new era of science: direct tests and images of a black hole’s event horizon itself!
Ethan Siegel is the author of Beyond the Galaxy and Treknology. You can pre-order his third book, currently in development: the Encyclopaedia Cosmologica.